Abstract
Background:
Ventilator-associated pneumonia (VAP) is one of the most common healthcare-related infections in pediatric and neonatal intensive care units. In the present study, due to the importance of prevention, early identification, and treatment of VAP, nasal and endotracheal microorganisms were determined in intubated patients admitted to the pediatric intensive care unit.Objectives:
The present study aims to determine the nasal and endotracheal (ETT) microorganisms in pediatric patients with intubated VAP admitted to the pediatric intensive care unit (PICU).Methods:
This cross-sectional two-group case-control study was conducted on 90 intubated patients: Sixty patients with VAP (30 nasal, 30 tracheal tubes) and 30 patients without VAP, hospitalized in the PICU department of Amir al Mominin Ali (AS) Hospital in Zabol, Iran, from March 2018 to December 2018. The criteria for diagnosing VAP was the clinical pulmonary infection score (CPIS). In both case and control groups, samples were collected from nasal tubes using nasal swabs and from tracheal tubes (ETT) as tracheal aspirates from intubated patients, and sent to the laboratory for culture. The collected samples were interpreted in the microbiology laboratory to identify microorganisms. Data collection was done using a demographic information questionnaire and the clinical pulmonary infection score CPIS. The data was entered into SPSS 20 statistical software and analyzed.Results:
The results of the present study showed that gender and age of patients do not affect the incidence of VAP, but children with VAP have a significantly longer stay in the PICU. Microbiological examination showed that Klebsiella and Escherichia coli (13.3%) were the most frequent among the positive nasal samples, and Klebsiella and E. coli (36.7%) were the most frequent among the positive tracheal samples. The diversity of microbes observed in ETT samples was also higher.Conclusions:
The present study showed that the duration of hospitalization in the pediatric intensive care unit, as well as the age of hospitalized patients, can increase the risk of VAP. Additionally, nasal sampling is effective in identifying and differentiating VAP patients. It is suggested to reduce the duration of hospitalization in these departments as much as possible and to pay more attention to older children to prevent the increase of VAP. The results indicate that nasal bacteria play a role in the more severe occurrence of ventilator-induced pneumonia. Therefore, it is recommended to use broad-spectrum antibiotics and implement preventive nursing measures. If possible, starting feeding earlier through a gastric tube is also suggested.Keywords
VAP Nosocomial Infection Endotracheal Tube (ETT) Nasal Microorganism Pediatrics Pediatric Intensive Care Unit
1. Background
After bloodstream infections, ventilator-associated pneumonia (VAP) is the second most frequently occurring nosocomial infection in Pediatric Intensive Care Units (PICU), accounting for up to 20% of all such infections (1, 2). Ventilator-associated pneumonia not only contributes to prolonged hospital stays and increased costs but also to increased mortality and morbidity (3). According to the definition by the Center for Disease Control, VAP is identified in patients who are supported by mechanical ventilation for more than 48 hours (4). Diagnosis of VAP based on the International Committee on Nosocomial Infections (NNIS) criteria in children includes a chest radiograph showing a new or progressive and persistent infiltrate, solidification, or cavitation occurring after 48 hours of starting mechanical ventilation, and the presence of at least three clinical criteria: Temperature instability, leukopenia less than 4000 or leukocytosis more than 15000, the appearance of purulent sputum or an increase in sputum amount, apnea, tachypnea or nasal flaring, wheezing or rales or rhonchi in lung auscultation, bradycardia or tachycardia, gas ventilation, and increased need for supplemental oxygen. A positive blood culture that is not related to another infectious source and a positive pleural fluid culture can replace any of the clinical criteria (5).
Ventilator-associated pneumonia increases the length of stay in the ICU by 28%, and for each day of hospitalization in the ICU, an average of 10% of patients will get pneumonia, most often in the form of VAP (6). The detection frequency is not always constant and changes depending on the duration of mechanical ventilation. The highest risk is in the first 5 days of hospitalization, and after about 2 weeks, it reaches a steady-state of 1% per day (7).
Ventilator-associated pneumonia can be seen in two forms: Early and late. Early VAP occurs 48 to 96 hours after artificial ventilation and is often caused by bacteria sensitive to antibiotics. Late VAP occurs more than 96 hours after intubation and is usually the result of antibiotic-resistant pathogens (8). Studies have shown that patients on ventilators are quickly infected with Gram-negative microorganisms (9). Common causes of VAP in many hospitals include Pseudomonas aeruginosa and methicillin-resistant Staphylococcus aureus (MRSA). Viral and fungal agents, though less prevalent, can cause VAP in immunocompromised individuals. Other pathogens that cause VAP include Enterobacter, Staphylococcus aureus, Acinetobacter, Klebsiella, and Escherichia coli, which can vary in prevalence depending on the hospital, diseases, and departments (10).
Previously, it was thought that pathogens entered the lungs from mechanical ventilation equipment, but studies have shown that the main cause of pneumonia in ventilated patients originates from sources other than the mechanical ventilation equipment itself (11). The main path of pathogens causing ventilator-associated pneumonia is a combination of two paths: Bacteria in the upper airway and upper digestive tract, and aspiration of materials into the lower airway (12).
Studies on nasal secretions in hospitalized individuals have shown that the normal flora of nasal microorganisms includes Staphylococcus albus and aureus, diphtheria bacilli, and, to a lesser extent, Moraxella catarrhalis (13). Pneumococcus has been found in nasal secretion cultures in children with colds. Additionally, the presence of streptococci and pneumococci bacteria is observed in nasal cultures of individuals with underlying diseases, sinus problems, and traumas (14).
Promptness in diagnosis is key to prognosis. However, this can be challenging, despite many guidelines, because of the wide spectrum of differential diagnoses for inpatient respiratory declines. Diagnosis is based on the clinical presentation of symptoms that occur after 48 hours of mechanical ventilation and require a change in ventilator settings. Many studies agree that new lung infiltrates in chest imaging are major evidence supporting a VAP diagnosis. Several tests need to be performed quickly to isolate the etiological pathogen and tailor targeted antibiotic treatment. This includes sampling sputum and blood for cultures, obtaining PCR-based tracheal sputum (from suctioning or from BAL procedure), and/or nasopharyngeal swabs, along with imaging methods. Imaging remains the gold standard for VAP diagnosis, together with pathogen identification and/or signs of inflammation and/or impaired gas exchange (3).
The prevention and timely treatment of ventilator-induced pneumonia are crucial for preventing patient death, reducing ICU hospitalization duration, and cutting treatment costs. Choosing the appropriate antibiotic treatment, maintaining hygiene, using sterile equipment, and observing sterile conditions in ICU patients, especially those with VAP, require sufficient knowledge of the causative microorganisms. Due to the lack of similar studies in this context for children and the high importance of microorganisms colonized in the upper airways and tracheal tubes in the development of VAP, we decided to compare nasal and tracheal microorganisms in children hospitalized in the Pediatric Intensive Care Unit (PICU).
2. Objectives
The present study aims to determine the nasal and endotracheal (ETT) microorganisms in pediatric patients with intubated VAP admitted to the pediatric intensive care unit (PICU).
3. Methods
This cross-sectional study was conducted on 90 intubated patients hospitalized in the PICU department of Amirul Mominin Ali (AS) Hospital from March 2018 to December 2018. The sample size required to determine the difference in the frequency of positive cultures in the two groups (92.3% for cases and 63.2% for controls) was based on Nachian's study (16), considering a type 1 error of 0.05 and a statistical power of 0.80. Using Stata software Version 14, the statistical calculations determined a need for 30 people in each group.
P0: Frequency of positive culture in the control group; P1: Frequency of positive culture in the VAP group.
A demographic information questionnaire and the clinical pulmonary infection scoring tool (CPIS) were used to collect data. The demographic information form included age, gender, weight, and length of hospitalization. The CPIS included six main indicators for diagnosing pneumonia: Body temperature, white blood cell count, chest X-ray, oxygenation, culture of tracheal secretions, and the volume and appearance of tracheal secretions (17). This instrument consists of 6 subscales including: (1) temperature: Score 0 if 36.5 ≤ T ≤ 38.4, score 1 if 38.5 ≤ T ≤ 38.9, score 2 if T < 36.5 or T ≥ 39; (2) white blood cell (WBC) Count: Score 0 if 4000 ≤ WBC ≤ 11000, score 1 if WBC > 11000 or WBC < 4000, score 2 if WBC > 1100 or WBC < 4000; (3) oxygenation: Score 0 if PaO2/FiO2 > 240 or ARDS is present, score 2 if PaO2/FiO2 ≤ 240 and no ARDS; (4) chest radiography: No infiltration: Score 0, diffuse infiltration: Score 1, local infiltration: Score 2; (5) tracheal secretion culture: Pathogenic bacteria did not grow or only a small amount: Score 0, moderate to high growth of pathogenic bacteria: Score 1, growth of pathogenic bacteria of the same type seen in gram staining: Score 2; (6) volume and appearance of tracheal secretions: Absence of pulmonary secretions: Score 0, presence of non-purulent pulmonary secretions: Score 1, presence of purulent pulmonary secretions: Score 2.
Each subscale is scored between 0 and 2, with a total score of 12. In this model, scores ≥ 6 at baseline or after the entry of microbiological results were considered indicative of pneumonia.
This scoring system had 93% sensitivity and 100% specificity. The range of scores was from 0 to 12, and a score higher than 6 was consistent with the diagnosis of pneumonia (15).
Inclusion criteria included hospitalization in the pediatric intensive care unit, endotracheal intubation, consent of the patient's supervisor to participate in the study, age between 1 month and 14 years, no severe injury in the mouth and jaw area, less than 12 hours since hospitalization in the hospital, no antibiotic use before and during hospitalization, no chronic respiratory disease, no immune system disorder and fever above 38 degrees, no use of anticoagulants, no diabetes or malignancy, and absence of wounds, infections, and oral trauma. Exclusion criteria included the death of patients less than 48 hours after intubation and lack of consent from the patient's supervisor.
In this study, first, a CBC test was taken from patients hospitalized in the pediatric intensive care unit, and then with the help of a Sysmex KX21 device (made by Sysmex, Japan), cell differentiation tests and peripheral blood cell counts were performed (16). For all patients, one type of device was used and calibrated by the hospital's medical equipment engineer. The chest image was taken with a portable radiology device model 01344-43014T made by PTW company in Germany (17). The same type of device was used for all patients and calibrated by medical equipment engineers. Arterial oxygen saturation was measured with a Nellcor NPB-295 pulse oximeter with a pediatric probe, which measures the percentage of oxygen saturation (18). Its performance was checked by an anesthesiologist. To measure tracheal tube cuff pressure, a standard manometer (Ireland Mallinckrodt model, Athlon Medical, with an accuracy of 2 cm of water) was used, which has an international standard. A mercury glass thermometer model (19) was used to measure body temperature, and its reliability was checked by the researcher by comparing it with two other mercury glass thermometers.
Based on the clinical condition of the patient and CPIS criteria, 90 intubated patients were randomly divided into two groups: Sixty patients with VAP (nasal and ETT group) and 30 patients without VAP (control group participants).
From qualified and intubated patients, a sample of nasal secretions was taken from inside the nose with a sterile swab and placed inside a sterile test tube, while tracheal secretions were collected by catheter suction and sent to the laboratory for culture. The samples collected from the nose and trachea were cultured for the identification of microorganisms in the microbiology laboratory using blood Agar (BA) for the growth of aerobic and facultative anaerobic microorganisms, and eosin methylene blue (EMB) for the isolation of bacteria, according to standard methods. All bacterial cultures were incubated at 37°C for 48 hours, while fungal cultures were kept at ambient temperature for 1 week. The isolated microbial colonies on the culture medium were then examined, and stained slides were prepared from each colony to identify different genera and species of microbial agents.
All culture media were prepared by Merck, Germany, and the same type of culture medium from the same manufacturer was used for all patients. To avoid errors, all experiments were conducted by experienced laboratory science personnel.
3.1. Data Analysis
The data and information were recorded and analyzed using SPSS 20 statistical software. The characteristics of individuals in the two groups were compared using independent t-tests and chi-square tests. A significance level of P < 0.05 was considered significant.
3.2. Ethical Consideration
The present article is derived from the thesis of a doctoral student and received permission from the ethics committee (ethics code IR.ZBMU.REC.1399.126) at Zabol University of Medical Sciences. The research was conducted with the coordination of hospital officials and in compliance with all ethical considerations.
4. Results
The results of the present study showed that the average age of patients in the VAP and control groups was 6.6 and 5.3 years, respectively, and this difference was not statistically significant (P = 0.199). However, the average length of hospitalization in the intensive care unit was 13.8 days in the VAP group and 9.4 days in the control group, which was statistically significant (P = 0.003). In this study, 43.3% of hospitalized children were male, and 56.7% were female.
Microbiological analysis of nasal samples showed that 58.3% of cases were negative. Among the positive samples, Klebsiella and E. coli accounted for 28.3% and 13.3%, respectively. The microbiological examination of tracheal samples showed that 61.7% of the cases were negative. The results of the different observed strains are shown in Table 1.
Frequency Distribution of Microorganisms
Culture Results and Category | No. (%) |
---|---|
Nasal | |
Negative | 35 (58.3) |
Klebsiella | 17 (28.3) |
E. coli | 8 (13.3) |
ETT | |
Negative | 37 (61.7) |
Klebsiella | 12 (20) |
Proteus | 2 (3.3) |
Salmonella | 3 (5) |
E. coli | 6 (10) |
Total | 60 (100) |
A comparison of the frequency of microbiological results of nasal samples in the two study groups showed that 50% of the nasal samples from VAP patients and 66.7% of the nasal samples from the control group were microbiologically negative. Klebsiella infection was positive in 36.7% of the nasal samples from patients with VAP and 20% of the nasal samples from the control group. The difference between the two groups in terms of the frequency of types of microbes in nasal samples was not statistically significant (P = 0.335) (Table 2). Figure 1 illustrates the distribution of microbiological results of nasal samples, showing that Klebsiella and E. coli were the most common organisms found in these samples in both VAP and non-VAP groups, with no significant difference in their distribution between the two groups.
Comparison of the Frequency of Microbiology Results of Nasal Samples
Microbiology Results of Nasal Samples | Group | P-Value | |
---|---|---|---|
VAP | Non-VAP | ||
0.335 | |||
Negative | 15 (50) | 20 (66.7) | |
Klebsiella | 11 (36.7) | 6 (20) | |
E. coli | 4 (13.3) | 4 (13.3) |
Comparison of the frequency of microbiology results of nose samples
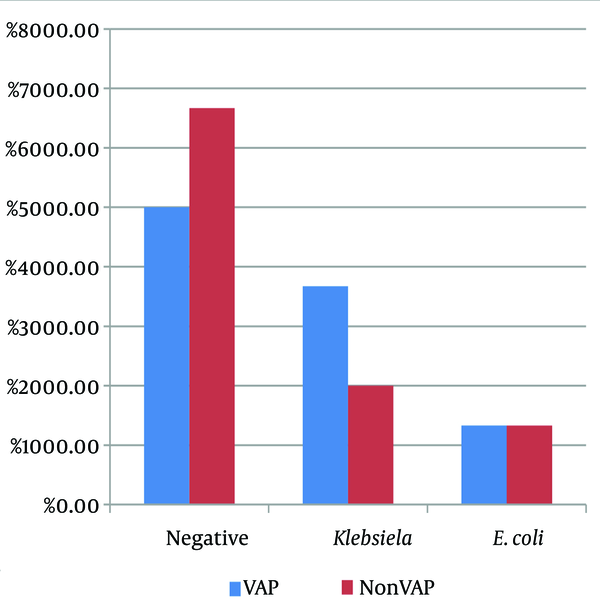
Comparing the frequency of the microbiological results of the ETT samples showed that 73.3% of the tracheal tube samples from VAP patients and 50% of the ETT samples from the control group were microbiologically negative. The results related to other types of microbes in tracheal tube samples are shown in Table 3.
Comparing the Frequency of Microbiology Results of ETT Samples
Microbiological Results of ETT Samples | Groups | P-Value | |
---|---|---|---|
VAP | Non-VAP | ||
ETT | 0.073 | ||
Negative | 22 (73.3) | 15 (50) | |
Klebsiella | 4 (13.3) | 8 (26.7) | |
Proteus | 0 (0) | 2 (6.7) | |
E. coli | 4 (13.3) | 2 (6.7) | |
Salmonella | 0 (0) | 3 (10) |
The difference between the two groups in terms of the frequency of microbes in the tracheal samples was not statistically significant (P = 0.335). Figure 2 illustrates the distribution of microbiological results of ETT samples append in the studied community. The findings indicated that Klebsiella, E. coli, Salmonella, and Proteus were the most common microorganisms found in these samples. However, the frequency of these microorganisms in the two groups (VAP and non-VAP) was not significantly different (Figure 2).
Comparison of frequency of microbiological results of ETT samples
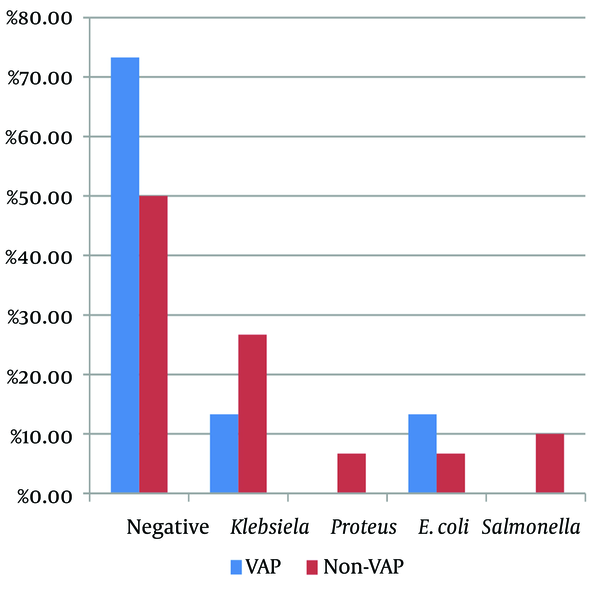
The raw and adjusted ratios of various factors for VAP showed that each one-year increase in the age of patients hospitalized in the special department increased the risk of VAP by 9%, which was not statistically significant. However, in the adjusted analysis, after accounting for factors such as gender and length of hospitalization, the effect of age on VAP became significant. On the other hand, each additional day of hospitalization in the special ward increased the chance of VAP by 16% in the raw analysis and by 21% in the adjusted analysis, both of which were statistically significant. However, gender did not have a significant effect on the development of VAP in any of the analyses.
5. Discussion
Ventilator-associated pneumonia VAP is one of the most common healthcare-associated infections in pediatric and neonatal intensive care units, associated with prolonged PICU stays, the use of mechanical ventilation, and extensive use of antibiotics. However, the incidence of VAP in neonatal and pediatric ICUs varies considerably worldwide (6). Therefore, this study aimed to determine nasal and tracheal microorganisms in intubated patients admitted to the pediatric intensive care unit, considering the importance of prevention, early identification, prognosis, and treatment of patients with VAP.
Examining the samples of VAP patients showed that these patients are mostly female, but they do not differ from non-VAP patients in terms of gender. Therefore, it seems that the incidence of VAP is not related to gender. The samples of VAP patients showed that these patients had an average age of 6.6 years, but there was no difference in the average age compared to non-VAP patients. However, adjusted analysis showed that each additional year of age could increase the risk of VAP in these patients by 20%. Therefore, the incidence of VAP seems to be related to the age of the patients. These results were consistent with the findings of the study by Antalova et al., which found that the gender of children did not affect the incidence of VAP. However, a significant relationship was found between the mean age and the incidence of VAP (3).
Accordingly, the probability of VAP in patients with a higher average age (40 months) was higher than in patients with a lower average age (9 months). In general, there is no consensus on the effect of gender on the incidence of VAP. Some studies have reported male gender as a risk factor for pneumonia, while others have found a higher incidence in females. However, the probability of VAP occurrence appears to be the same in both sexes (15, 20).
The results also showed that the obvious difference between the two groups was that VAP patients were hospitalized in the PICU for an average of 14 days, significantly longer than the control group. This indicates that the length of hospitalization can affect the incidence of VAP. The results of multivariate analysis also showed that each additional day of hospitalization in the intensive care unit increases the risk of VAP by more than 20%. In their study, Almuneef et al. also evaluated children with VAP in the PICU and stated that the length of stay in the PICU for the VAP group (34 days) was longer than for the group without VAP (15 days), which has a significant effect on the incidence of this disease (16).
In Patria et al.'s study, the results showed that compared to non-VAP children, children with VAP had longer PICU stays, higher hospitalization rates, and increased mortality (17). Since VAP is associated with increased medical costs and higher mortality, it is necessary and important to conduct multicenter studies to improve treatment approaches and prevention.
The microbiological examination of the samples showed that about 50% of the nasal samples from VAP patients were microbially positive, which was not significantly different from the control group. This indicates that the microbiological results of nasal samples in the studied VAP patients did not significantly aid in distinguishing these patients from other patients hospitalized in the PICU. Among the positive cases, the highest frequency was related to Klebsiella, which accounted for 36.7% of all samples.
On the other hand, the microbiological examination of tracheal samples showed that only about 27% of tracheal samples in VAP patients were microbially positive, which was not significantly different from the control group. Among the positive tracheal samples, the highest frequencies were related to Klebsiella and E. coli, each accounting for 13.3% of the total samples. In a study conducted by Almuneef et al. on patients with VAP in Saudi Arabia, the most common organisms found in tracheal samples were Pseudomonas aeruginosa, followed by Staphylococcus aureus (16). Ergul et al. also identified Pseudomonas, Klebsiella, and Acinetobacter as the most common organisms in their study (18). In their study on identifying microorganisms isolated from tracheal tubes in children with VAP, they stated that the most common microorganism isolated was Acinetobacter. They also found that secretion culture and antibiogram showed the highest resistance to ciprofloxacin, possibly due to extensive trial use before cultivation results. These bacteria showed sensitivity to imipenem and piperacillin-tazobactam (19, 21, 22).
These results demonstrate the diversity of bacteria responsible for ventilator-associated pneumonia (VAP). Solutions must be sought to reduce the incidence of this condition. One reason for this variation is the high bacterial load in tracheal aspiration, which acts as a reservoir of infection, where bacterial proliferation and biomass are stronger. The diversity of bacteria, incomplete antibiotic treatments, different host immune responses, and the duration of intubation collectively exacerbate the condition.
In Nateghian et al.'s study, the most common organisms identified were Acinetobacter (33%) and Pseudomonas (29%) (20). It has been reported that infections caused by these organisms can originate from internal sources, such as the oropharynx or stomach, or from external sources, such as contaminated respiratory tools (3). A comparison of nasal and tracheal samples in VAP patients showed that although neither had higher diagnostic accuracy than samples from non-VAP patients, the accuracy of tracheal samples appeared slightly higher. However, the diversity of microbes in endotracheal tube ETT samples was also higher. The results indicate that nasal bacteria contribute to the more severe occurrence of pneumonia in VAP cases. Therefore, it is recommended to use broad-spectrum antibiotics and preventive nursing measures, and, if possible, to start feeding earlier through a gastric tube.
One limitation of the study was the absence of several influencing variables, such as underlying diseases or drugs, which could affect the development of VAP. Another limitation was the small sample size and the restriction to teaching hospitals as the research environment.
The researcher acknowledges that, in the case of microbiology of VAP, the diagnostic criteria of non-bronchoscopic BAL and bronchoscopic BAL are very valuable. However, in the research environment of Zabul city, facilities are limited to aspiration and direct culture of ETT, and there are no relevant sub-specialties (respiration of children, adults, thoracic surgeon). Therefore, the current research has used these methods for sampling.
5.1. Conclusions
The present study showed that the duration of hospitalization in the pediatric intensive care unit, as well as the advanced age of hospitalized patients, can be effective in increasing the risk of VAP. Additionally, nasal sampling is effective in identifying and differentiating VAP patients. Considering that all patients in this study were from one hospital in one city and no significant differences were observed in the microbiological results of the samples, it is suggested that future studies include a larger sample size and involve patients from multiple hospitals. To prevent the increase of VAP, it is recommended to reduce the duration of hospitalization in these departments as much as possible and to pay more attention to older children.
Acknowledgements
References
-
1.
Rosenthal VD. Health-care-associated infections in developing countries. Lancet. 2011;377(9761):186-8. [PubMed ID: 21146208]. https://doi.org/10.1016/S0140-6736(10)62005-3.
-
2.
Kalamitsou S, Violaki A, Iosifidis E, Avramidou V, Mantzafleri P, Karaiskou E, et al. Ventilator associated pneumonia (VAP) in children: A diagnostic challenge. Signa Vitae. 2023;19(4). https://doi.org/10.22514/sv.2023.050.
-
3.
Antalova N, Klucka J, Rihova M, Polackova S, Pokorna A, Stourac P. Ventilator-associated pneumonia prevention in pediatric patients: Narrative review. Children (Basel). 2022;9(10). [PubMed ID: 36291475]. [PubMed Central ID: PMC9600673]. https://doi.org/10.3390/children9101540.
-
4.
Papakyritsi D, Iosifidis E, Kalamitsou S, Chorafa E, Volakli E, Pena-Lopez Y, et al. Epidemiology and outcomes of ventilator-associated events in critically ill children: Evaluation of three different definitions. Infect Control Hosp Epidemiol. 2023;44(2):216-21. [PubMed ID: 35506391]. https://doi.org/10.1017/ice.2022.97.
-
5.
Kumar A, Tanwar S, Chetiwal R, Kumar R. Nosocomial infections-related antimicrobial resistance in a multidisciplinary intensive care unit. MGM J Med Sci. 2022;9(1):12-8. https://doi.org/10.4103/mgmj.mgmj_110_21.
-
6.
Raycheva R, Rangelova V, Kevorkyan A. Cost analysis for patients with ventilator-associated pneumonia in the neonatal intensive care unit. Healthcare (Basel). 2022;10(6). [PubMed ID: 35742032]. [PubMed Central ID: PMC9223030]. https://doi.org/10.3390/healthcare10060980.
-
7.
Akhatar SMS, Mwipopo EE, Zhao D, Li W, Rahmathullah MN, Hassan MA. Endo-tracheal tube associated bacterial infection, prevalence and their drug susceptibility pattern in NICU. Inter J Med Dental Sci. 2016. https://doi.org/10.19056/ijmdsjssmes/2016/v5i2/100629.
-
8.
Kharel S, Bist A, Mishra SK. Ventilator-associated pneumonia among ICU patients in WHO southeast asian region: A systematic review. PLoS One. 2021;16(3). e0247832. [PubMed ID: 33690663]. [PubMed Central ID: PMC7942996]. https://doi.org/10.1371/journal.pone.0247832.
-
9.
Giacobbe DR, Roberts JA, Abdul-Aziz MH, de Montmollin E, Timsit JF, Bassetti M. Treatment of ventilator-associated pneumonia due to carbapenem-resistant Gram-negative bacteria with novel agents: a contemporary, multidisciplinary ESGCIP perspective. Expert Rev Anti Infect Ther. 2022;20(7):963-79. [PubMed ID: 35385681]. https://doi.org/10.1080/14787210.2022.2063838.
-
10.
Labovská S. Pseudomonas aeruginosa as a Cause of Nosocomial Infections. Pseudomonas aeruginosa-Biofilm Formation, Infections and Treatments. IntechOpen; 2021. https://doi.org/10.5772/intechopen.95908.
-
11.
Lynch J3, Zhanel GG. Pseudomonas aeruginosa pneumonia: Evolution of antimicrobial resistance and implications for therapy. Semin Respir Crit Care Med. 2022;43(2):191-218. [PubMed ID: 35062038]. https://doi.org/10.1055/s-0041-1740109.
-
12.
Jongers B, Hotterbeekx A, Bielen K, Vervliet P, Boddaert J, Lammens C, et al. Identification of potential urinary metabolite biomarkers of pseudomonas aeruginosa ventilator-associated pneumonia. Biomark Insights. 2022;17:11772719221099100. [PubMed ID: 35592849]. [PubMed Central ID: PMC9112676]. https://doi.org/10.1177/11772719221099131.
-
13.
Klompas M. New insights into the prevention of hospital-acquired pneumonia/ventilator-associated pneumonia caused by viruses. Semin Respir Crit Care Med. 2022;43(2):295-303. [PubMed ID: 35042261]. https://doi.org/10.1055/s-0041-1740582.
-
14.
Basset A, Wall E, Mitsi E, Deshusses C, Daly R, Pojar S, et al. Targeted transcriptomic screen of pneumococcal genes expressed during murine and human infection. Infect Immun. 2022;90(7). e0017522. [PubMed ID: 35674445]. [PubMed Central ID: PMC9302103]. https://doi.org/10.1128/iai.00175-22.
-
15.
Moghaddam RS, Rahnama M, Abdollahimohammad A, Naderifar M. Comparison of rinsing with chlorhexidine and matrica mouthwashes on the ventilator-associated pneumonia. Romanian J Military Med. 2022;125(2):213. https://doi.org/10.55453/rjmm.2022.125.2.6.
-
16.
Almuneef M, Memish ZA, Balkhy HH, Alalem H, Abutaleb A. Ventilator-associated pneumonia in a pediatric intensive care unit in Saudi Arabia: A 30-month prospective surveillance. Infect Control Hosp Epidemiol. 2004;25(9):753-8. [PubMed ID: 15484800]. https://doi.org/10.1086/502472.
-
17.
Patria MF, Chidini G, Ughi L, Montani C, Prandi E, Galeone C, et al. Ventilator-associated pneumonia in an Italian pediatric intensive care unit: A prospective study. World J Pediatr. 2013;9(4):365-8. [PubMed ID: 24235070]. https://doi.org/10.1007/s12519-013-0444-y.
-
18.
Ergul AB, Cetin S, Altintop YA, Bozdemir SE, Ozcan A, Altug U, et al. Evaluation of microorganisms causing ventilator-associated pneumonia in a pediatric intensive care unit. Eurasian J Med. 2017;49(2):87-91. [PubMed ID: 28638248]. [PubMed Central ID: PMC5469850]. https://doi.org/10.5152/eurasianjmed.2017.16262.
-
19.
Rebmann T, Greene LR. Preventing ventilator-associated pneumonia: An executive summary of the association for professionals in infection control and epidemiology, Inc, elimination guide. Am J Infect Control. 2010;38(8):647-9. [PubMed ID: 20868931]. https://doi.org/10.1016/j.ajic.2010.08.004.
-
20.
Nateghian A, Omrani A, Alipour Z, Haerinejad M. [Causes of ventilator associated pneumonia in pediatrics ICU]. Iran South Med J. 2016;19(1):98-105. Persian. [PubMed Central ID: PMC27717603]. https://doi.org/10.7508/ismj.1395.01.009.
-
21.
Abdollahi A, Shoar S, Shoar N. Microorganisms’ colonization and their antibiotic resistance pattern in oro-tracheal tube. Iran J Microbiol. 2013;5(2):107-2. [PubMed ID: 23825725]. [PubMed Central ID: PMC3696843].
-
22.
Khanbabaee G, Akbarizadeh M, Sayyari A, Ashayeri-Panah M, Abdollahgorji F, Sheibani K, et al. A survey on pulmonary pathogens and their antibiotic susceptibility among cystic fibrosis patients. Braz J Infect Dis. 2012;16(2):122-8. [PubMed ID: 22552452].