Abstract
Background:
Developing novel prognostic markers is crucial for childhood T-cell acute lymphoblastic leukemia (T-ALL).Objectives:
This study aimed to investigate whether the prognostic impact of CD34, a progenitor marker, is associated with outcomes.Methods:
This retrospective cohort study included 82 risk-adapted pediatric patients with T-ALL who were treated under the National Protocol of Childhood ALL in China (NPCLC)-ALL2008 from March 2008 to December 2016.Results:
CD34 expression was observed in 37.8% of all T-ALL patients, with a median expression level of 62.7%. Patients with CD34 expression had a favorable event-free survival (EFS, 74.2% [66.3% - 82.1%]) with a hazard ratio of 0.69 (P = 0.33) and a reduced cumulative incidence of relapse (CIR, 22.6% [15.0% - 30.2%]; P = 0.86) at five years. Even after adjusting for potential factors and competing events in multivariable regression models, there was no connection between the CD34 immunophenotype and the risk of recurrence.Conclusions:
CD34 expression did not serve as a predictive factor for relapse in this limited series. Larger multicenter studies are recommended to determine whether this biomarker at initial diagnosis can predict outcomes.Keywords
1. Background
T-cell acute lymphoblastic leukemia is a malignant expansion of immature T cells, accounting for approximately 15% of pediatric acute lymphoblastic leukemia (ALL) cases (1). Long-term survival rates for childhood ALL approach or even exceed 90% with current therapies (2). However, even with risk-adapted and intensive frontline therapy, nearly 30% of children with the T-cell subtype of ALL experience relapse or refractory disease (3, 4).
Several demographic and clinical characteristics have historically been used as prognostic markers for therapeutic stratification, including age, initial white blood cell (WBC) count, and early response to prednisone (5, 6). Minimal residual disease (MRD) is a powerful prognostic factor (7), but it requires strict standardization and is generally not available at baseline. Immunophenotyping and gene expression analysis of T-ALL cells have revealed heterogeneity (8, 9), partly associated with maturation arrest at different developmental stages, each with a unique set of biological and molecular features related to outcomes.
Specific immunophenotypic markers such as CD56 and CD99, which involve distinct cytogenetic groups, have been investigated for their association with prognosis, with varying findings (8, 10, 11). CD34 surface expression has been studied as a potential prognostic biomarker for leukemia, not only because it is present on immature cells but also due to its role in migration and adhesion (12). In B-lineage ALL, the co-culture of bone marrow mesenchymal stromal cells with leukemic cells results in the upregulation of CD34 (13). However, these findings have not yet been validated from a clinical perspective, and there is no firm evidence linking immunophenotypes, including CD34, to clinical outcomes.
Although some studies suggest that the CD34 expression pattern may have prognostic utility in B-ALL, similar data on pediatric T-ALL are rarely reported in the literature. Here, we report the expression and prognostic impact of CD34 in a series of pediatric T-ALL patients.
2. Objectives
The primary objective of this study was to assess the association between CD34 expression and relapse in pediatric T-cell acute lymphoblastic leukemia.
3. Methods
3.1. Patients
Children aged 1 to 16 years who were newly diagnosed with T-ALL at the Children’s Hospital of Zhejiang University School of Medicine between March 2008 and December 2016 were included in this retrospective study. The last follow-up was on December 31, 2021. This study was approved by the medical ethics committee of the Children’s Hospital of Zhejiang University School of Medicine, and written informed consent was obtained from the patients' guardians.
The diagnosis of T-ALL was established using morphological, histochemical, and flow cytometric analyses of leukemic cells for immunophenotypic characterization. Cytogenetic abnormalities were identified through conventional cytogenetic analysis and PCR.
3.2. Immunophenotyping
Immunophenotyping was performed at diagnosis using standard methods. Mononuclear cells were collected using Ficoll gradient centrifugation, washed, and stained with fluorochrome-labeled monoclonal antibodies. They were then analyzed using a FACS Calibur with CellQuest software from BD Biosciences. Antibodies against the markers CD1a, CD2, surface (s) CD3, cytoplasmic (c) CD3, CD4, CD5, CD7, and CD8 were used for the T-cell lineage, while CD19, CD10, CD20, cCD22, sCD22, and sIgM were used for the B-cell lineage. CD13, CD14, CD15, CD33, CD117, and MPO were used for the myeloid lineage, and CD34, CD45, CD56, HLA-DR, and nTdT were used as non-lineage markers. The population of blasts was gated based on scatter parameters and the expression of CD45, CD7, and/or cytoplasmic CD3. Antigen expression was considered positive when more than 20% of the lymphoblasts reacted with the monoclonal antibodies, according to the EGIL criteria (14). The ETP-ALL immunophenotype was defined according to Coustan-Smith et al. (15): (1) lack of CD1a and CD8 expression (< 5% of blast cells); (2) absence or weak expression of CD5 (< 75% positive blasts); and (3) expression of one or more myeloid or stem cell markers, including CD117, CD11b, CD13, CD33, CD34, and HLA-DR.
MRD was measured by flow cytometry, and the quadruple labeling antibody combinations used are detailed in Appendix 1 in Supplementary File. MRD results below the individual lower limits of detection were set to 10-5. MRD was monitored at the following time points (TP): TP1, day 15 of induction therapy; TP2, day 33 of induction therapy; and TP3, week 10 (at the end of consolidation therapy). Leukemic involvement of less than 0.01% of nucleated bone marrow cells was defined as MRD negative.
3.3. Treatment
Based on their biological and clinical features, as well as their early phase response to treatment, the patients were classified into intermediate-risk (IR) or high-risk (HR) groups according to the revised National Cancer Institute (NCI) criteria. Patients diagnosed with T-ALL through immunophenotyping were classified as IR. Those with at least one of the following risk factors were classified as HR: Initial peripheral WBC ≥ 100 × 109/L, more than 25% blasts in the bone marrow on day 15 of induction therapy, failure to achieve complete remission (CR) at the end of induction therapy, presence of MLL gene rearrangement, hypodiploidy, TCF3-HLF fusion, MRD-TP2 ≥ 1%, or MRD-TP3 ≥ 0.1%.
Chemotherapy was administered according to the National Protocol of Childhood ALL in China (NPCLC)-ALL2008 (16). The response to treatment was assessed using prednisone response on day 8, bone marrow remission status on days 15 and 33 of induction therapy, and MRD at the three time points.
CR was defined as fewer than 5% blasts in regenerating bone marrow, absence of leukemia blasts in peripheral blood and cerebrospinal fluid (CSF), and no signs of extramedullary disease. Relapse was defined as the recurrence of more than 5% leukemic blasts or localized leukemic infiltrates at any site.
3.4. Statistical Analysis
The primary outcome of this study was event-free survival (EFS). EFS and overall survival were defined as previously published (17). Failure to achieve remission due to early death or non-response was considered an event at time zero. Survival estimates with 95% confidence intervals (CI) were calculated using the Kaplan-Meier method and compared with the log-rank test. Cumulative incidence functions for competing risks were analyzed using Gray’s test (18). Hazard ratios (HRs) and 95% CIs were estimated using the Cox proportional hazards model and the regression model of competing risk (19).
Covariate imbalances at baseline were assessed using standardized differences, as suggested by Mark et al. (20) and Thomas and Pencina (21), rather than P-values. Standardized differences of 0.2, 0.5, and 0.8 correspond to small, medium, and large effect sizes, respectively. For hypothesis testing, a P-value < 0.05 was considered statistically significant. Statistical analyses were performed using SPSS 21.0 software and R software (version 4.1.2).
4. Results
The cohort in this study included 82 children with newly diagnosed T-ALL, aged 1.5 - 15.3 years (median, 9.3), who were admitted to our hospital between March 2008 and December 2016. A summary of the demographic, clinical, and hematologic characteristics of these patients is provided in Table 1. The median follow-up time was 5.8 years (95% CI, 5.3 - 6.5). Nineteen patients experienced leukemic relapse, including 14 cases of isolated hematologic relapse, 2 cases of isolated CNS leukemia relapse, 1 case of combined hematologic and CNS relapse, and 2 cases of combined hematologic and testicular relapse. Translocation was found in 22 patients (26.8%; see Table 1). CD34 was positive in 31 (37.8%) patients. The demographic and clinical characteristics at baseline were similar between the CD34-positive patients and the CD34-negative group (Table 2). Analysis of other antigens showed no significant preferential expression of CD34 with the antigens CD33/CD13, CD117, and CD10 (Table 3).
Patient Data for Continuous Variables Median (IQR, Range) is Given, for Categorical Variables Number (%) is Given a
Clinical Characteristics | Values |
---|---|
Age (y) | 9.3 (1.5 - 15.3) |
Gender (male/female) | 64/18 (78/22) |
Initial WBC | 91.2 (25.8 - 193.5,1 - 638.5) × 109/L |
SIL-TAL1 | 9 (11.0) |
BCR-ABL | 5 (6.1) |
TEL/AML1 | 3 (3.7) |
MLL-ENL | 2 (2.4) |
HOX11 rearrangements | 2 (2.4) |
CBFβ/MYH11 | 1 (1.2) |
EOI FCM-MRD | 1.56 × 10-3 (5 × 10-4 -7.4 × 10-3, LOD -1.6 × 10-1) |
Follow-up time (y) | 5.8 (95%CI, 5.3 - 6.5) |
Relapse | 19 (23.7%, CI 18.9 - 28.5% b) |
Non-relapse mortality | 7 (8.6%, CI 5.5 - 11.7% b) |
Demographic and Clinical Characteristics According to CD34 Expression
Characteristics | CD34-Positive; (n = 31) | CD34-Negative; (n = 51) | Standardized Difference a |
---|---|---|---|
Male, No. (%) | 22 (71) | 42 (82) | 0.26 |
Age (y) b | 8.7 (6.7 - 10.6) | 9.9 (5.8 - 12.2) | 0.34 |
WBC count, × 109/L b | 111.7 (23.6 - 206.1) | 90.5 (34.9 - 191.6) | 0.14 |
Hemoglobin, g/L c | 97.1 (74.8 - 120.6) | 101.4 (75.4 - 127.4) | 0.15 |
Platelet, × 109/Lb | 69 (45 - 96) | 55 (36 - 96.5) | 0.17 |
Cytogenetic group | 7 (22.5) | 6 (11.7) | 0.29 |
Immunophenotypic Marker Expression on T-cell Acute Lymphoblastic Leukemia a
Immunophenotype | CD34-Positive | CD34-Negative | Standardized Difference |
---|---|---|---|
CD33/CD13 | 0.26 | ||
Positive | 5 (16.1) | 4 (7.9) | |
Negative | 26 (83.9) | 47 (92.1) | |
CD117 | 0.24 | ||
Positive | 4 (12.9) | 3 (5.9) | |
Negative | 27 (87.1) | 48 (94.1) | |
CD10 | 0.02 | ||
Positive | 2 (6.5) | 3 (5.9) | |
Negative | 29 (93.5) | 48 (94.1) |
Of the 82 treated cases, 53 (64.6%) patients were found to be responsive to prednisone. Sixty-two (75.6%) achieved CR on day 15 after induction therapy, and 75 (91.4%) achieved CR on day 33. Ultimately, 78 (95.1%) patients achieved CR before consolidation therapy, while the 5-year OS and 5-year EFS were 69.3% (95% CI, 63.9% - 74.4%) and 67.6% (95% CI, 62.4% - 72.8%), respectively. We examined the relationship between surface antigen expression and induction therapy response and found no correlation between CD34 surface expression and MRD level at the end of induction therapy (day 33) (Appendix 2 in Supplementary File). Given the considerable differences in consolidation between the positive and negative end-of-induction MRD groups, we comprehensively analyzed these two factors in four subgroups (Appendices 3 and 4 in Supplementary File).
We further analyzed the predictive impact of CD34 expression on EFS in conjunction with early clinical risk-related factors (age ≥10 years, WBC ≥ 100 × 109/L, and prednisone response) and myeloid markers (CD33 and/or CD13, CD10, or CD117), as well as a genetic group. The 5-year EFS was slightly longer but not significantly different in CD34+ T-ALL patients (P = 0.33; Table 4 and Figure 1A). The observed difference in the cumulative incidence of relapse between the two groups did not reach statistical significance (Figure 1B) after accounting for competing risks. Of all potential predictors, gender and CD33/CD13 were the two most significant (Table 4), in addition to CD34. Even after adjusting for CD33/CD13 and gender, CD34 expression did not show any association with the risk of recurrence in both the Cox regression model (HR 1.64, 95% CI [0.7 - 3.83]; P = 0.25) and the competing risks multivariable analysis (HR 0.97, 95% CI [0.38 - 2.45]; P = 0.95) (Table 5).
Event-Free Survival in T-cell Acute Lymphoblastic Leukemia
Parameter | 5 Years EFS; % (CI %) a | Plog-Rank |
---|---|---|
Clinical | ||
Age at diagnosis, (y) | 0.64 | |
<10 | 65.3 (59.6 - 70.0) | |
≥10 | 70.7 (62.9 - 78.5) | |
Gender | 0.21 | |
Male | 71.1 (65.4 - 76.8) | |
Female | 55.6 (43.9 - 67.3) | |
WBC | 0.95 | |
≤ 50 | 66.5 (57.8 - 75.2) | |
> 50 | 68.0 (61.4 - 74.6) | |
Prednisone response | 0.86 | |
Good | 67.9 (59.1 - 76.9) | |
Poor | 67.4 (60.9 - 73.9) | |
Immunophenotype | ||
CD34 expression | 0.33 | |
CD34+ | 74.2 (66.3 - 82.1) | |
CD34- | 63.5 (56.6 - 70.4) | |
CD33/CD13 | 0.43 | |
CD33/CD13+ | 76.2 (61.4 - 91.0) | |
CD33/CD13- | 66.5 (60.9 - 72.1) | |
CD117 | 0.88 | |
CD117+ | 66.7 (47.5 - 85.9) | |
CD117- | 67.5 (62.0 - 73.0) | |
Cytogenetic group | 0.59 | |
Mutant | 61.5 (48.0 - 75.0) | |
Wild-type | 68.8 (63.1 - 74.5) |
A, the 5-year EFS rate of T-cell acute lymphoblastic leukemia (T-ALL) patients compared between CD34+ and CD34- groups; B, the 5-year cumulative incidence of relapse of T-ALL patients compared between CD34+ and CD34- groups
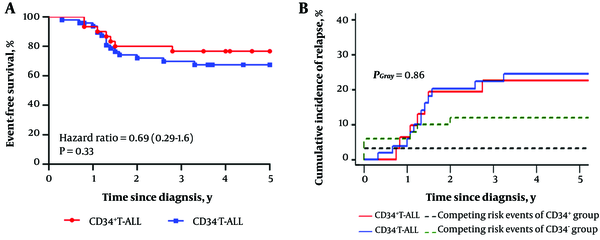
Associations of CD34 Expression with Risk of Relapse After T-ALL Diagnosis with Adjustment for Gender and CD33/CD13
Parameter | Hazard Ratio | 95%CI | P-Value |
---|---|---|---|
CD34-positive (Cox proportional hazard model) | 1.64 | 0.70 - 3.83 | 0.25 |
CD34-positive (Competing risks regression) | 0.97 | 0.38 - 2.45 | 0.95 |
5. Discussion
In this study, we assessed the predictive impact of CD34 expression on relapse in a cohort of pediatric patients with T-ALL who underwent treatment with a standardized, risk-adapted therapy protocol. The extended follow-up period enhances the reliability of the clinical outcomes.
CD34 is expressed on hematopoietic stem cells and thymic T-cell precursors, and its expression may indicate early T-cell maturation arrest in T-ALL (22). According to the World Health Organization (WHO) classification criteria, CD34 is associated with a unique subtype of T-ALL, early T-cell precursor acute lymphoblastic leukemia (ETP-ALL) (23), but not according to TCR criteria. Despite challenges in the diagnosis and management of ETP-ALL, evidence suggests that ETP cells share similarities with hematopoietic stem cells and myeloid progenitor cells in gene expression profiling. Previous studies have reported poor outcomes in both adult and pediatric patients with ETP-ALL compared to other T-ALL subtypes (15, 24). However, the prognostic impact of the ETP-ALL phenotype alone is a subject of debate (25, 26), as is the relationship between leukemia-associated immunophenotype and prognosis (8, 27). Similarly, in a study of 493 T-ALL patients, the CD34 antigen was found to have no independent prognostic significance (8), although an earlier study did show a correlation between CD34-positive T-ALL and worse outcomes (28). Cox regression analysis in this study showed that CD34 positivity was not associated with the risk of recurrence, even after controlling for the most significant potential confounding factors. Additional competing risk regression analyses confirmed these results and marginally narrowed the confidence intervals. Our results are consistent with those published by Pui et al. (22). Several factors, including heterogeneity in study populations, treatment regimens, and flow cytometry sensitivity, may explain these conflicting results.
Additionally, the therapy adapted according to MRD in our study might obscure an inverse relationship between CD34 and prognosis. Although there is no consensus on the optimal timing for MRD assessment, end-induction MRD has been widely recognized as a significant predictor for risk stratification and clinical outcomes (29, 30). Moreover, the combined effect of CD34 and MRD status on EFS might not alter the prognostic significance of MRD, but the effect is too minimal to warrant statistical analysis. It is also noteworthy that molecular and genetic heterogeneity is prevalent in CD34-positive leukemias, potentially contributing to variations in the prognostic value of CD34 expression. Indeed, a protein-protein interaction (PPI) network analysis revealed an overrepresentation of genes associated with the JAK-STAT signaling pathway in some CD34-positive leukemias (12).
The prognosis for pediatric T-ALL has seen significant advancements due to the adoption of intensive therapeutic strategies. Our cohort study reports a 5-year overall survival (OS) rate of 69.3%, an indicator of progress that remains modestly lower than the rates reported by Vora et al. (31) and Burns et al. (30). There are two potential reasons for this. First, the study was conducted over a long period, and the medical technology and economic conditions during different periods might have influenced the study results. Second, the application of risk stratification and treatment protocols for T-ALL patients at our center, which were similar to those used for B-ALL, may have inadvertently contributed to the observed suboptimal prognosis. Furthermore, the 5-year EFS of 67.6% in this study was close to the OS, underscoring the limited likelihood of achieving a second remission upon relapse in pediatric T-ALL patients. The prognosis is poor regardless of the MRD level post-induction. Identifying HR patients and applying more intensive therapy, such as hematopoietic stem cell transplantation, may be beneficial.
Nevertheless, the retrospective design introduces the risk of patient selection bias, which is the most significant limitation of the present study. Additionally, the relatively small sample size in a single center, due to the low incidence of the disease, may limit the generalizability of these findings. Finally, it is worth mentioning the absence of a power analysis. Prospective power analysis is valuable at the trial planning stage but is not applicable here. Therefore, we did not conduct a post-hoc power analysis and instead used confidence intervals to understand and interpret the results, as reported previously (32). Due to the wide CI in our study, we cautiously accept the hypothesis that CD34 expression is not predictive of relapse in this cohort of pediatric patients with T-ALL. Nonetheless, further multicenter studies are needed to evaluate the significance of immunophenotype in the future.
Despite these limitations, we found that CD34 expression is not associated with early treatment response and relapse. This single-institution study may not be adequately powered to detect a modest predictive effect of this immunophenotype. Thus, larger multicenter studies will be needed.
References
-
1.
Bodaar K, Yamagata N, Barthe A, Landrigan J, Chonghaile TN, Burns M, et al. JAK3 mutations and mitochondrial apoptosis resistance in T-cell acute lymphoblastic leukemia. Leukemia. 2022;36(6):1499-507. [PubMed ID: 35411095]. [PubMed Central ID: PMC9177679]. https://doi.org/10.1038/s41375-022-01558-5.
-
2.
Cui L, Li ZG, Chai YH, Yu J, Gao J, Zhu XF, et al. Outcome of children with newly diagnosed acute lymphoblastic leukemia treated with CCLG-ALL 2008: The first nation-wide prospective multicenter study in China. Am J Hematol. 2018;93(7):913-20. [PubMed ID: 29675840]. https://doi.org/10.1002/ajh.25124.
-
3.
Bride KL, Vincent TL, Im SY, Aplenc R, Barrett DM, Carroll WL, et al. Preclinical efficacy of daratumumab in T-cell acute lymphoblastic leukemia. Blood. 2018;131(9):995-9. [PubMed ID: 29305553]. [PubMed Central ID: PMC5833263]. https://doi.org/10.1182/blood-2017-07-794214.
-
4.
Rodriguez S, Abundis C, Boccalatte F, Mehrotra P, Chiang MY, Yui MA, et al. Therapeutic targeting of the E3 ubiquitin ligase SKP2 in T-ALL. Leukemia. 2020;34(5):1241-52. [PubMed ID: 31772299]. [PubMed Central ID: PMC7192844]. https://doi.org/10.1038/s41375-019-0653-z.
-
5.
Rowe JM, Buck G, Burnett AK, Chopra R, Wiernik PH, Richards SM, et al. Induction therapy for adults with acute lymphoblastic leukemia: Results of more than 1500 patients from the international ALL trial: MRC UKALL XII/ECOG E2993. Blood. 2005;106(12):3760-7. [PubMed ID: 16105981]. https://doi.org/10.1182/blood-2005-04-1623.
-
6.
Smith M, Arthur D, Camitta B, Carroll AJ, Crist W, Gaynon P, et al. Uniform approach to risk classification and treatment assignment for children with acute lymphoblastic leukemia. J Clin Oncol. 1996;14(1):18-24. [PubMed ID: 8558195]. https://doi.org/10.1200/jco.1996.14.1.18.
-
7.
Pui CH, Pei D, Coustan-Smith E, Jeha S, Cheng C, Bowman WP, et al. Clinical utility of sequential minimal residual disease measurements in the context of risk-based therapy in childhood acute lymphoblastic leukaemia: A prospective study. Lancet Oncol. 2015;16(4):465-74. [PubMed ID: 25800893]. [PubMed Central ID: PMC4612585]. https://doi.org/10.1016/s1470-2045(15)70082-3.
-
8.
Fuhrmann S, Schabath R, Möricke A, Zimmermann M, Kunz JB, Kulozik AE, et al. Expression of CD56 defines a distinct subgroup in childhood T-ALL with inferior outcome. Results of the ALL-BFM 2000 trial. Br J Haematol. 2018;183(1):96-103. [PubMed ID: 30028023]. https://doi.org/10.1111/bjh.15503.
-
9.
Petit A, Trinquand A, Chevret S, Ballerini P, Cayuela JM, Grardel N, et al. Oncogenetic mutations combined with MRD improve outcome prediction in pediatric T-cell acute lymphoblastic leukemia. Blood. 2018;131(3):289-300. [PubMed ID: 29051182]. https://doi.org/10.1182/blood-2017-04-778829.
-
10.
Ebadi M, Jonart LM, Ostergaard J, Gordon PM. CD99 antibody disrupts T-cell acute lymphoblastic leukemia adhesion to meningeal cells and attenuates chemoresistance. Sci Rep. 2021;11(1):24374. [PubMed ID: 34934147]. [PubMed Central ID: PMC8692434]. https://doi.org/10.1038/s41598-021-03929-x.
-
11.
Enein AA, Rahman HA, Sharkawy NE, Elhamid SA, Abbas SM, Abdelfaatah R, et al. Significance of CD99 expression in T-lineage acute lymphoblastic leukemia. Cancer Biomark. 2016;17(2):117-23. [PubMed ID: 27002769]. https://doi.org/10.3233/cbm-160608.
-
12.
Modvig S, Wernersson R, Øbro NF, Olsen LR, Christensen C, Rosthøj S, et al. High CD34 surface expression in BCP-ALL predicts poor induction therapy response and is associated with altered expression of genes related to cell migration and adhesion. Mol Oncol. 2022;16(10):2015-30. [PubMed ID: 35271751]. [PubMed Central ID: PMC9120905]. https://doi.org/10.1002/1878-0261.13207.
-
13.
Kihira K, Chelakkot VS, Kainuma H, Okumura Y, Tsuboya N, Okamura S, et al. Close interaction with bone marrow mesenchymal stromal cells induces the development of cancer stem cell-like immunophenotype in B cell precursor acute lymphoblastic leukemia cells. Int J Hematol. 2020;112(6):795-806. [PubMed ID: 32862292]. https://doi.org/10.1007/s12185-020-02981-z.
-
14.
Ratei R, Schabath R, Karawajew L, Zimmermann M, Möricke A, Schrappe M, et al. Lineage classification of childhood acute lymphoblastic leukemia according to the EGIL recommendations: results of the ALL-BFM 2000 trial. Klin Padiatr. 2013;225 Suppl 1:S34-9. [PubMed ID: 23700065]. https://doi.org/10.1055/s-0033-1337961.
-
15.
Coustan-Smith E, Mullighan CG, Onciu M, Behm FG, Raimondi SC, Pei D, et al. Early T-cell precursor leukaemia: A subtype of very high-risk acute lymphoblastic leukaemia. Lancet Oncol. 2009;10(2):147-56. [PubMed ID: 19147408]. [PubMed Central ID: PMC2840241]. https://doi.org/10.1016/s1470-2045(08)70314-0.
-
16.
Liao C, Xu X, Shen D, Song H, Xu W, Zhao F, et al. Minimal residual disease-guided risk restratification and therapy improves the survival of childhood acute lymphoblastic leukemia: Experience from a tertiary children's hospital in China. J Pediatr Hematol Oncol. 2019;41(6):e346-54. [PubMed ID: 30640823]. https://doi.org/10.1097/mph.0000000000001412.
-
17.
Möricke A, Zimmermann M, Valsecchi MG, Stanulla M, Biondi A, Mann G, et al. Dexamethasone vs prednisone in induction treatment of pediatric ALL: Results of the randomized trial AIEOP-BFM ALL 2000. Blood. 2016;127(17):2101-12. [PubMed ID: 26888258]. https://doi.org/10.1182/blood-2015-09-670729.
-
18.
Scrucca L, Santucci A, Aversa F. Competing risk analysis using R: An easy guide for clinicians. Bone Marrow Transplant. 2007;40(4):381-7. [PubMed ID: 17563735]. https://doi.org/10.1038/sj.bmt.1705727.
-
19.
Scrucca L, Santucci A, Aversa F. Regression modeling of competing risk using R: An in depth guide for clinicians. Bone Marrow Transplant. 2010;45(9):1388-95. [PubMed ID: 20062101]. https://doi.org/10.1038/bmt.2009.359.
-
20.
Mark DB, Lee KL, Harrell FJ. Understanding the role of P values and hypothesis tests in clinical research. JAMA Cardiol. 2016;1(9):1048-54. [PubMed ID: 27732700]. https://doi.org/10.1001/jamacardio.2016.3312.
-
21.
Thomas LE, Pencina MJ. Do not over (P) value your research article. JAMA cardiology. 2016;1(9):1055.
-
22.
Pui CH, Hancock ML, Head DR, Rivera GK, Look AT, Sandlund JT, et al. Clinical significance of CD34 expression in childhood acute lymphoblastic leukemia. Blood. 1993;82(3):889-94. [PubMed ID: 7687897].
-
23.
Arber DA, Orazi A, Hasserjian R, Thiele J, Borowitz MJ, Le Beau MM, et al. The 2016 revision to the World Health Organization classification of myeloid neoplasms and acute leukemia. Blood. 2016;127(20):2391-405. [PubMed ID: 27069254]. https://doi.org/10.1182/blood-2016-03-643544.
-
24.
Jain N, Lamb AV, O'Brien S, Ravandi F, Konopleva M, Jabbour E, et al. Early T-cell precursor acute lymphoblastic leukemia/lymphoma (ETP-ALL/LBL) in adolescents and adults: a high-risk subtype. Blood. 2016;127(15):1863-9. [PubMed ID: 26747249]. [PubMed Central ID: PMC4915808]. https://doi.org/10.1182/blood-2015-08-661702.
-
25.
Patrick K, Wade R, Goulden N, Mitchell C, Moorman AV, Rowntree C, et al. Outcome for children and young people with Early T-cell precursor acute lymphoblastic leukaemia treated on a contemporary protocol, UKALL 2003. Br J Haematol. 2014;166(3):421-4. [PubMed ID: 24708207]. https://doi.org/10.1111/bjh.12882.
-
26.
van Grotel M, Meijerink JP, van Wering ER, Langerak AW, Beverloo HB, Buijs-Gladdines JG, et al. Prognostic significance of molecular-cytogenetic abnormalities in pediatric T-ALL is not explained by immunophenotypic differences. Leukemia. 2008;22(1):124-31. [PubMed ID: 17928886]. https://doi.org/10.1038/sj.leu.2404957.
-
27.
Tolba FM, Foda ME, Kamal HM, Elshabrawy DA. Expression of CD133 in acute leukemia. Med Oncol. 2013;30(2):527. [PubMed ID: 23532815]. https://doi.org/10.1007/s12032-013-0527-6.
-
28.
van Grotel M, van den Heuvel-Eibrink MM, van Wering ER, van Noesel MM, Kamps WA, Veerman AJ, et al. CD34 expression is associated with poor survival in pediatric T-cell acute lymphoblastic leukemia. Pediatr Blood Cancer. 2008;51(6):737-40. [PubMed ID: 18683236]. https://doi.org/10.1002/pbc.21707.
-
29.
Xue YJ, Wang Y, Lu AD, Jia YP, Zuo YX, Ding MM, et al. Clinical Analysis of Pediatric T-Cell Acute Lymphoblastic Leukemia Using the MRD-Oriented Strategy System. Clin Lymphoma Myeloma Leuk. 2023;23(7):477-83. [PubMed ID: 37080879]. https://doi.org/10.1016/j.clml.2023.03.013.
-
30.
Burns MA, Place AE, Stevenson KE, Gutiérrez A, Forrest S, Pikman Y, et al. Identification of prognostic factors in childhood T-cell acute lymphoblastic leukemia: Results from DFCI ALL Consortium Protocols 05-001 and 11-001. Pediatr Blood Cancer. 2021;68(1). e28719. [PubMed ID: 33026184]. [PubMed Central ID: PMC8369809]. https://doi.org/10.1002/pbc.28719.
-
31.
Vora A, Goulden N, Mitchell C, Hancock J, Hough R, Rowntree C, et al. Augmented post-remission therapy for a minimal residual disease-defined high-risk subgroup of children and young people with clinical standard-risk and intermediate-risk acute lymphoblastic leukaemia (UKALL 2003): a randomised controlled trial. Lancet Oncol. 2014;15(8):809-18. [PubMed ID: 24924991]. https://doi.org/10.1016/s1470-2045(14)70243-8.
-
32.
Hung ML, Wu JX, Li N, Livhits MJ, Yeh MW. Association of radioactive iodine administration after reoperation with outcomes among patients with recurrent or persistent papillary thyroid cancer. JAMA Surg. 2018;153(12):1098-104. [PubMed ID: 30140908]. [PubMed Central ID: PMC6583014]. https://doi.org/10.1001/jamasurg.2018.2659.