Abstract
Background:
Numerous research studies have unveiled the significant impacts of neurofeedback (NFB) on individuals suffering from depression, anxiety, and stress.Objectives:
In light of these findings, the current trial aimed to assess the efficacy of neurofeedback in alleviating stress symptoms among students experiencing high levels of stress, utilizing the neurofeedback method.Methods:
The trial comprised 30 students aged between 15 and 25 years old who were identified as experiencing psychological distress. To ensure an in-depth investigation and account for potential confounding variables, we employed a randomized controlled trial design, randomly allocating participants to either the experimental or control group. The first group received an 8-week course of neurofeedback treatment, while the control group did not receive any specific treatment but underwent psychological assessments at predetermined intervals. Baseline measurements were taken at the commencement of the study, utilizing the Depression Anxiety Stress Scales (DASS-21) and salivary tests. Subsequent measurements were conducted after 4 weeks and at the conclusion of the research. The collected data underwent repeated measures of variance analysis for statistical evaluation.Results:
At the conclusion, the mean of the experimental group (36.8) and control group (76.67) were found to be significantly different (P < 0.001). Furthermore, after 4 weeks into the study, the repeated measures variance analysis revealed that the mean of the experimental group was lower than that of the other group (P < 0.05, ES: 0.651).Conclusions:
The results of this trial suggest that neurofeedback (NFB) can be considered an effective, low-risk, non-invasive treatment option for individuals experiencing high levels of stress, as indicated by the significant reduction in salivary biomarkers.Keywords
Psychological Stress Neurofeedback, Salivary Cortisol α-amylase Level Brain Waves
1. Background
Stress refers to the incapability to effectively manage a perceived danger to one's physical, emotional, or psychological well-being (1, 2). Stress-related conditions can significantly impact an individual's quality of life and lead to disruptions in daily functioning, which, in turn, can interfere with social interactions (3). Numerous studies have investigated the effects of stress on various aspects, including behavioral (4, 5), cognitive (6), neurovascular (7), and cardiovascular (8).
Furthermore, neuroimaging studies have provided evidence of the consequences of stress-related disorders, leading to physiological and mental deterioration (9, 10), as well as cognitive impairment (11). These studies have shown associations between stress-related disorders, shrinkage of the hippocampus and prefrontal cortex (PFC) (12), and weakening of the prefrontal network (13, 14). Physiologically, glucocorticoids (GCs) are stress hormones released in response to traumatic situations. Based on Cannon's theory, GCs, principally cortisol in humans and corticosterone in animals, are the end results of activity in the hypothalamic–pituitary–adrenal (HPA) axis (9, 15). The prefrontal cortex and the hippocampus conduct inhibitory activities in the HPA axis, with the hippocampus regulating negative feedback of the stress axis (16). Chronic exposure to GCs has been extensively studied from various perspectives in recent decades. Researchers have described the neuroendocrine profiles of certain stress-related diseases and investigated possible mechanisms underlying cognitive deficits, psychopathologies, and therapeutic approaches. Therefore, in this paper, we discuss a novel method of neurotherapy, specifically focusing on neurofeedback (NFB), which is considered one of the earliest techniques (17-19).
Among nonpharmacological treatments, NFB treatment has been utilized as a potentially successful intervention for years. Neurofeedback is a type of biofeedback that focuses on the neuronal activity of the brain (20). The training method is based on reinforcement learning, where real-time feedback provided to the trainee is supposed to reward and reinforce desired brain activity or inhibit unfavorable activity patterns. During EEG-neurofeedback education, participants learn to regulate their emotions through operant conditioning using electrical brain activities (21, 22). The overall aim of neurofeedback is to improve mental states, which can be assessed by emotional variables in all types of populations (23-25). Several studies have provided evidence for the effects of this training in ADHD, depression, and OCD patients, supporting its potential therapeutic application for stress and anxiety-related pathologies (26, 27). Initial trials evaluating the effectiveness of EEG-NFB in treating mood swings, agitation, and post-traumatic stress disorder (PTSD) have presented promising outcomes (28). Moreover, research investigating PTSD or generalized anxiety disorder (GAD) has indicated that individuals who received EEG-NFB demonstrated significant improvements compared to control groups (28-30). However, it is worth noting that there are studies that present more cautious conclusions regarding the effectiveness of this treatment (27).
Extensive interventions are used to reduce anxiety and depression symptoms. To augment the validity of employing NFB for mood disorders (20), it is important to measure objective correlations (31) (i.e., with biological variables) alongside subjective variables (32) (i.e., with psychometric tests). Cortisol, a proven biomarker of stress (33), is expected to decrease following the implementation of a clinical NFB intervention. This expectation is rational because one of the asserted benefits of NFB is the induction of calmness and relaxation among patients with anxiety (34), which can be measured by a reduction in both psychological symptomatology and salivary cortisol levels (35). In light of these points, NFB can be proposed as an effective treatment that provides a comprehensive psychophysiological exploration of its effects on the neural basis (36). Also, none of the previous studies have investigated the effect of neurofeedback on biological and psychometric indicators simultaneously.
2. Objectives
In this study, we intended to assess both subjective improvements reported by self-assessment (questionnaire) and observable issues in the form of hormonal appraisals. To achieve this, we employed an experimental plan in accordance with recent suggestions for investigating the effects of NFB in student populations (5).
3. Methods
3.1. Participants
A total of 60 volunteer undergraduate students, comprising 30 young men and 30 young women who were right-handed, were selected to participate in this trial. The participants fell within the age range of 18 to 25 years (mean = 21.81, SD = 2.5). Prior to the commencement of the trial, a screening process was conducted on 60 students within the age range of 18 to 25 years. However, 8 students did not meet the determined inclusion criteria, while 4 students were excluded according to the specified exclusion criteria (psychedelic pill usage). Consequently, 24 students were assigned to the experimental group, while another 24 students were allocated to the control group. During the trial, 18 students in weeks 3 - 4 declined to continue their participation. Ultimately, both groups consisted of 15 students who successfully completed the trial. The control group did not receive any form of treatment and solely underwent assessments at the designated intervals.
The inclusion criteria for participant selection entailed achieving a score above 18 on the stress scale of the Stress, Anxiety, and Depression Questionnaire (DASS-21), being between the ages of 18 and 25 years. The exclusion criteria encompassed a history of severe psychiatric disorders (including periods of severe depression, anxiety disorders, and substance use disorders), smoking and psychedelic pill usage, intake of psychiatric medications, epilepsy, various cardiovascular and respiratory disorders, blood pressure abnormalities, recent consumption of Aspirin and acetaminophen within the past 48 hours, history of cancer, diabetes, hypothyroidism or hyperthyroidism, addison's disease, Cushing's syndrome, hypertension, and use of corticosteroids and steroids. All participants were advised to refrain from swallowing (except water) and smoking for a minimum of 1 hour before the trial. Furthermore, all students provided informed consent, which included information about the study's purpose, the right to withdraw from the study, remaining anonymous, publishing information, and any possible risks by signing the necessary documentation.
This randomized, parallel-group clinical trial was conducted over a two-month period, specifically from July 2021 to November 2021, at the Mehr Psychiatric Hospital, affiliated with Lorestan University of Medical Sciences. The trial protocol received approval from the ethical committee of Baqiyatallah Hospital (approval ID: IR.BMSU.BAQ.REC.1399.003). Moreover, the trial procedures adhered to the principles outlined in the Declaration of Helsinki.
3.2. Determination of Sample Size
Statistical indexes, namely the mean and standard deviation, were calculated for 2 distinct groups. The mean score for group 1 was found to be 2.85, while for group 2, it was 2.44. Furthermore, the standard deviations for group 1 and group 2 were determined to be 0.7 and 0.4, respectively. These calculations were based on prior studies and a pilot study conducted by previous authors (5).
To ensure adequate statistical power (α) of 0.05 and a power (β) of 0.2, it was initially determined that a minimum of 15 patients were essential for each group, resulting in a total of 30 patients for both groups (n = 30). However, considering the potential attrition rate, a statistics specialist recommended increasing the sample size. Therefore, it was proposed to recruit 30 patients for each of the two groups, resulting in a total sample size of 60 patients (n = 60). By adopting this larger sample size, it was anticipated that the study would maintain a statistical power of 80% (P < 0.05), ensuring a greater likelihood of detecting meaningful effects or differences between the groups.
3.3. Randomization
The randomization method employed in this study was a simple randomization approach, with the individual participant as the unit of randomization. To ensure a fair distribution, participants were randomly assigned to the 2 groups using a table of random numbers. Specifically, students with even numbers were allocated to the control group, while students with odd numbers were allocated to the experimental group. The responsibility for random allocation rested with the researcher, ensuring impartiality in the process. It is noteworthy that neither the clinical caregiver nor the data analyzer had prior knowledge of the patient allocation. To maintain blinding during the study, researchers utilized consecutively numbered, opaque, and sealed envelopes for rating and allocating participants. The treatment allocation remained concealed from the psychiatrist and the data analyzer. Participants were given the opportunity to select envelopes from a box containing them, and these envelopes were enclosed in an unspecified wrapper. Although participants were aware of the experimental group they were assigned to, the specific treatment they would receive remained concealed until the envelope was opened.
3.4. Interventions
3.4.1. Instrument
3.4.1.1. Depression Anxiety Stress Scales
The DASS-21 is a psychometric tool consisting of 21 items distributed across three subscales, each containing seven items. The questionnaire is designed to evaluate symptoms related to depression, anxiety, and general stress. For instance, depressive symptoms may encompass feelings of being down-hearted and experiencing a sense of melancholy, while anxiety symptoms may involve sensations of being on the verge of panic. General stress symptoms may manifest as a tendency to overreact to various situations.
Participants are requested to assess their status on a 4-point questionnaire, where 0 indicates that the given item did not relate to them at all, and 3 indicates that it related to them almost always. It is important to note that higher scores on the DASS-21 indicate a greater degree of psychological distress (4, 5, 37). Confirmatory factor analyses were conducted to evaluate the underlying structure of the DASS-21. Moreover, Cronbach's alpha coefficients (Cronbach α: 0.761 to 0.906) and correlation coefficients were computed to evaluate the psychometric indexes of the DASS-21. Specifically, correlation coefficients were assessed between the factor scores of the DASS-21 and the indexes of the validated Vietnamese form of the Duke Health Profile Adolescent (ADHP-V) (10, 38). Based on the findings, it can be concluded that the DASS-21 demonstrates satisfactory internal consistency and convergent validity. Therefore, it is considered a reliable and appropriate instrument for assessing symptoms associated with common mental health problems, particularly depression and anxiety (39, 40).
3.4.1.2. Assessment of Salivary Biomarkers
The study participants were required to provide a total of four saliva samples, each consisting of 5 mL of saliva deposited into plastic vials. The collection time for each sample was limited to no more than 5 minutes. Subsequently, the samples underwent centrifugation at 3000 rpm for 15 minutes, resulting in a clear supernatant with low viscosity, which was then stored at a temperature of -20°C. The analysis of the samples was conducted using the Salimetrics commercial salivary cortisol enzyme-linked immunosorbent assay kit obtained from Newmarket, UK. The laboratory responsible for the analysis was located in Khorram Abad, Lorestan, Iran. The assay kit exhibited a sensitivity of 0.007 ug/dL. To ensure accuracy, all students were assessed equally within the same trial.
To assess the precision of the assay, both within-assay and inter-assay variation coefficients were determined. Importantly, all variation coefficients were found to be below 10%, indicating a high level of consistency and reliability.
3.4.1.3. Neurofeedback
The electroencephalogram (EEG) signals underwent a series of recording, processing, filtering, and representation procedures using the BioGraph Infinity EEG Suite SA7950 software. This software amplified the amplitude of the frequency bands while the hardware Pro Comp 2 Infinity (Technology Ltb; Montreal, Quebec) transmitted the signal to the computer. The EEG served both as a means of recording and as a source of feedback, with a sampling rate of 256 Hz samples per second, and the data were redirected to the computer via an A/D converter. An active scalp electrode was positioned at C4 for theta/beta1 training, while the reference and ground electrodes were placed on the right and left earlobe, respectively. Impedance was consistently maintained below 10 KΩ, and artifact rejection thresholds were established to postpone feedback in the presence of eye movements or other motor activities that could cause fluctuations in the EEG.
The EEG data from site C4 underwent fast Fourier transform (FFT), band-pass filtering (ranging from 0.1 to 60 Hz), and notch filtering (50 Hz) to remove electrical interference. This allowed for continuous measurement of the amplitude values for beta1 (16 - 21 Hz), SMR (12 - 15 Hz), and theta (4 - 7 Hz) in microvolts (μV), peak-to-peak, based on the suggestions from recent studies. Hence, our protocols involved theta inhibition and beta1/SMR reinforcement, which have been deemed valid in prior research (15).
Before the NFB training, a 4-minute baseline was recorded to determine the reinforcement threshold for each band. To maintain a consistent level of reinforcement during the training session, the feedback thresholds were automatically adjusted between blocks. The software utilized in our study obtained four mean amplitude values per second. In the case of beta1 and SMR thresholds, if the participant exceeded 3 out of 4 means above the mean amplitude of the prior block, a reward was awarded, and the threshold was increased by 0.10 microvolts. Conversely, for the theta band, the participant had to fall below 3 out of 4 means below the mean amplitude of the past block.
An audio-visual protocol dependent on the threshold was employed as reward stimuli feedback. When the EEG signals remained within the specified thresholds, the participant received a reward in the form of continuous music playback and various images. However, if the participant failed to meet the threshold criteria, the audio would cease, and the images would remain static.
3.5. Procedure
Upon completion of the informed consent process and the medical questionnaire by the participants, a preliminary examination was conducted to establish a baseline. Subsequently, the NFB sessions were administered twice a week in the experimental group until a total of 8 sessions had been completed. Each NFB session followed a pre-post design in which anxiety levels were assessed, and samples of salivary cortisol were collected before and after the NFB intervention. At the same time, saliva samples and assessments using the DASS-21 were collected at baseline, as well as in weeks 4 and 8, for both the experimental and control groups. It is important to note that the control group did not receive any NFB treatment and was solely assessed at the predetermined intervals.
The NFB training protocol consisted of three distinct components. First, electrodes were attached to the participant's scalp, and an initial EEG measurement was taken to establish the baseline brain activity. During a 4-minute period of open-eyed rest, without any feedback provided, the students were instructed to relax and focus on a symbol displayed on a computer. The preliminary EEG measurement was obtained using the software's programs, utilizing specific channels and filtering frequency bands of interest.
Subsequently, the NFB session commenced, lasting 45 minutes and incorporating real-time feedback. Participants were instructed to relax, listen to accompanying music, and observe the changing imagery on the computer screen throughout the entire NFB training session. Following this, a 4-minute EEG recording, along with an additional cortisol sample and anxiety assessment, were obtained. Finally, participants were scheduled for a final assessment using the DASS-21 to evaluate any alterations resulting from the NFB intervention.
4. Results
A total of 60 students aged between 18 and 25 years underwent screening for the trial. However, it was determined that 8 students did not meet the inclusion criteria, and 4 additional students were excluded based on the exclusion criteria. Consequently, 24 students were assigned to the experimental group, while another 24 students were randomly allocated to the control group. During weeks 3 - 4, 18 students declined to continue participating in the trial, resulting in a final count of 15 students in each group who successfully completed the trial (Figure 1). It is worth noting that there were no significant differences in baseline characteristics, particularly with regard to age, between the two groups (Tables 1 and 2).
The population of the trial
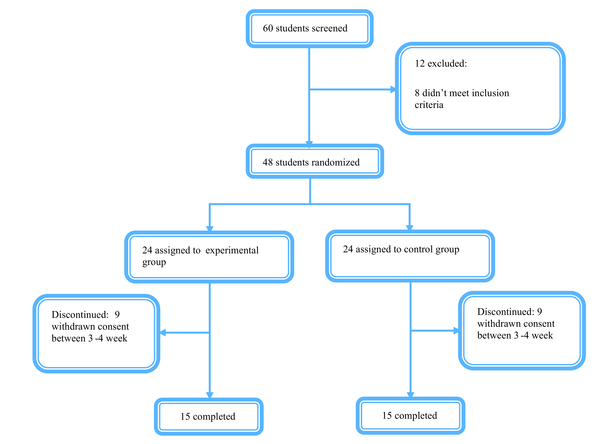
Demographic Characteristic
Variables | Groups | Total | P-Value | ||||
---|---|---|---|---|---|---|---|
Control | Experimental | ||||||
No. | % | No. | % | No. | % | ||
Sex | |||||||
Male | 9 | 60.0 | 10 | 66.7 | 19 | 63.3 | 0.705 |
Female | 6 | 40.0 | 5 | 33.3 | 11 | 36.7 | |
Total | 15 | 100.0 | 15 | 100.0 | 30 | 100.0 | |
Marital status | 0.456 | ||||||
Single | 8 | 53.3 | 10 | 66.7 | 18 | 60.0 | |
Married | 7 | 46.7 | 5 | 33.3 | 12 | 40.0 | |
Total | 15 | 100.0 | 15 | 100.0 | 30 | 100.0 | |
Education | 0.464 | ||||||
Bachelor | 7 | 46.7 | 9 | 60.0 | 16 | 53.3 | |
MA | 8 | 53.3 | 6 | 40.0 | 14 | 46.7 | |
Total | 15 | 100.0 | 15 | 100.0 | 30 | 100.0 |
Comparative Analysis of Variables Conducted Between the Experimental and Control Groups
Variables | N | Mean | S.E Mean | Difference Mean | P-Value Leven Test | P-Value Difference Mean |
---|---|---|---|---|---|---|
Age | -1.13 | 0.383 | 0.14 | |||
Experimental | 15 | 21.33 | 0.475 | |||
Control | 15 | 22.47 | 0.576 | |||
Depression | 0.93 | 0.169 | 0.625 | |||
Experimental | 15 | 32.40 | 1.222 | |||
Control | 15 | 31.47 | 1.440 | |||
Anxiety | -1.73 | 0.829 | 0.21 | |||
Experimental | 15 | 32.27 | 1.012 | |||
Control | 15 | 34.00 | 0.894 | |||
Stress | -0.27 | 0.988 | 0.894 | |||
Experimental | 15 | 31.87 | 1.302 | |||
Control | 15 | 32.13 | 1.492 | |||
Total | -1.07 | 0.779 | 0.766 | |||
Experimental | 15 | 96.53 | 2.280 | |||
Control | 15 | 97.60 | 2.713 | |||
Cortisol | 0.08 | 0.358 | 0.954 | |||
Experimental | 15 | 27.98 | 1.081 | |||
Control | 15 | 27.90 | 0.827 | |||
α-amylase | 887.07 | 0.522 | 0.416 | |||
Experimental | 15 | 160765.67 | 696.422 | |||
Control | 15 | 159878.60 | 817.216 | |||
proportion alfa/cor | 70.23 | 0.183 | 0.813 | |||
Experimental | 15 | 5871.94 | 236.969 | |||
Control | 15 | 5801.72 | 175.293 |
Initially, a chi-square test was employed to match the qualitative demographic characteristics of the participants in the experimental and control groups. Additionally, a mean test of the mean of two independent communities was utilized to compare the mean values of the quantitative variables prior to the intervention. The results indicated homogeneity in the two groups, signifying that there was no statistically significant variation in terms of gender, marital status, education level, age, cortisol, and alpha-amylase levels, as well as DASS questionnaire indicators, prior to the intervention (P-value > 0.05).
Subsequently, due to the observed homogeneity between the two groups, an independent two-sample t-test was conducted to evaluate the effect of the intervention during the fourth and eighth weeks. The results of this analysis have been presented in the form of designed tables. Furthermore, in the concluding section of this study, the ratio of alpha-amylase to cortisol was compared and contrasted between the experimental and control groups. This comparison serves as a clinical indicator for psychological diagnosis.
As presented in Table 3, a comparative analysis of variables was conducted between the experimental and control groups after four weeks. The findings revealed a statistically significant impact of the intervention on reducing psychological and hormonal indicators, as evidenced by a P-value below 0.05. Notably, the ratio of alpha-amylase to cortisol exhibited a noteworthy decline by approximately one-third in the experimental group. Specifically, the average ratio was 60 in the control group and 220 in the experimental group. This disparity also demonstrated statistical significance (Figures 2 and 3).
Comparative Analysis of Variables Conducted Between the Experimental and Control Groups After 4 Weeks
Variables | N | Mean | S.E Mean | Difference Mean | P-Value Leven Test | P-Value Difference Mean | ES |
---|---|---|---|---|---|---|---|
Depression | -13.60 | 0.065 | < 0.001 | 0.233 | |||
Experimental | 15 | 12.53 | 0.816 | ||||
Control | 15 | 26.13 | 1.638 | ||||
Anxiety | -17.73 | 0.157 | < 0.001 | 0.243 | |||
Experimental | 15 | 11.33 | 1.008 | ||||
Control | 15 | 29.07 | 1.498 | ||||
Stress | -18.44 | 0.152 | < 0.001 | 0.20 | |||
Experimental | 15 | 11.33 | 1.008 | ||||
Control | 15 | 29.77 | 1.498 | ||||
Total | -49.07 | 0.12 | < 0.001 | 0.461 | |||
Experimental | 15 | 35.20 | 2.444 | ||||
Control | 15 | 84.27 | 3.799 | ||||
Cortisol | -5.46 | 0.286 | < 0.001 | 0.391 | |||
Experimental | 15 | 20.64 | 0.863 | ||||
Control | 15 | 26.10 | 0.695 | ||||
α-amylase | -113783.23 | 0.889 | < 0.001 | 0.431 | |||
Experimental | 15 | 44767.51 | 796.806 | ||||
Control | 15 | 158550.73 | 773.683 | ||||
proportion alfa/cor | -3907.96 | 0.058 | < 0.001 | 0.33 | |||
Experimental | 15 | 2228.32 | 108.724 | ||||
Control | 15 | 6136.28 | 168.930 |
Cortisol index after 4 - 8 weeks
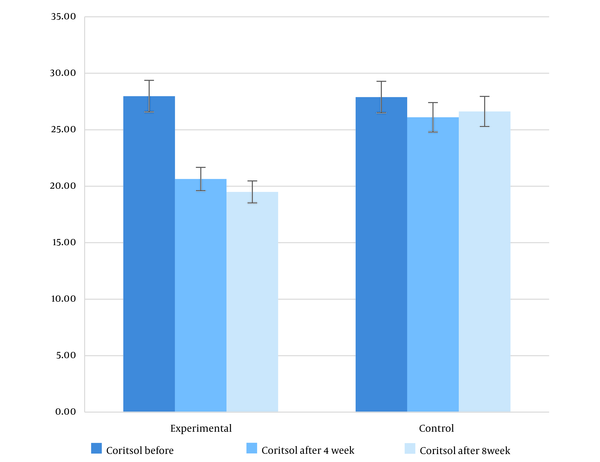
α-amylase index after 4 - 8 weeks
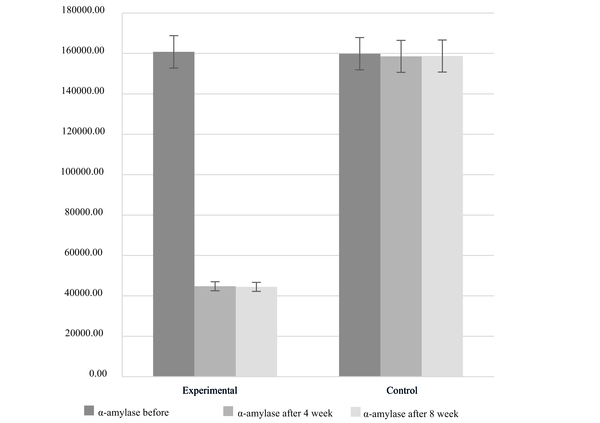
Table 4 further illustrates the outcomes observed during the eighth week, which closely resembled those observed in the fourth week. These results indicate that the intervention's effectiveness in reducing the studied factors remained consistent, with no significant changes observed between weeks 4 and 8. Consequently, the findings of this study affirm that a 4-week duration is sufficient to achieve favorable outcomes through the intervention.
Comparative Analysis of Variables Conducted Between the Experimental and Control Groups After 8 Weeks
Variables | N | Mean | S.E Mean | Difference Mean | P-Value Leven Test | P-Value Difference Mean | ES |
---|---|---|---|---|---|---|---|
Depression | -13.60 | 0.065 | < 0.001 | 0.373 | |||
Experimental | 15 | 12.53 | 0.82 | ||||
Control | 15 | 26.13 | 1.64 | ||||
Anxiety | -12.40 | 0.41 | < 0.001 | 0.293 | |||
Experimental | 15 | 12.00 | 1.28 | ||||
Control | 15 | 24.40 | 2.12 | ||||
Stress | -13.87 | 0.972 | < 0.001 | 0.25 | |||
Experimental | 15 | 12.27 | 1.03 | ||||
Control | 15 | 26.13 | 1.55 | ||||
Total | -39.87 | 0.113 | < 0.001 | 0.561 | |||
Experimental | 15 | 36.80 | 2.04 | ||||
Control | 15 | 76.67 | 3.53 | ||||
Cortisol | -7.13 | 0.444 | < 0.001 | 0.501 | |||
Experimental | 15 | 19.50 | 0.78 | ||||
Control | 15 | 26.62 | 0.69 | ||||
α-amylase | -114279.43 | 0.468 | < 0.001 | 0.478 | |||
Experimental | 15 | 44449.37 | 881.43 | ||||
Control | 15 | 158728.80 | 752.77 | ||||
Proportion alfa/cor | -3667.43 | 0.638 | < 0.001 | 0.467 | |||
Experimental | 15 | 2345.99 | 125.48 | ||||
Control | 15 | 6013.42 | 143.23 |
Furthermore, this study investigated the ratio of alpha-amylase to cortisol. Among healthy individuals, this ratio typically ranges around 60 in international units. However, participants in the intervention group displayed a ratio of 20. These findings suggest that clinical testing and calculation of the alpha-amylase to cortisol ratio may serve as an effective means of diagnosing depression. Specifically, a ratio exceeding 2 indicates a likelihood of depression, whereas a ratio below 2 suggests the absence of depression (Figures 4 and 5). As such, it is recommended that clinicians first administer a clinical test and compute this index instead of relying solely on questionnaire-based assessments. This clinical approach holds potential as a psychological diagnostic method within the field of clinical psychology.
The ratio of α-amylase/cortisol after 4 - 8 weeks
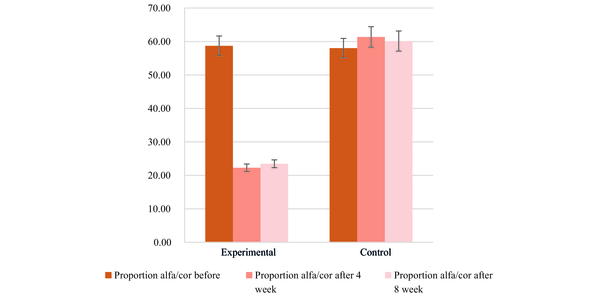
Ratio of α-amylase/cortisol
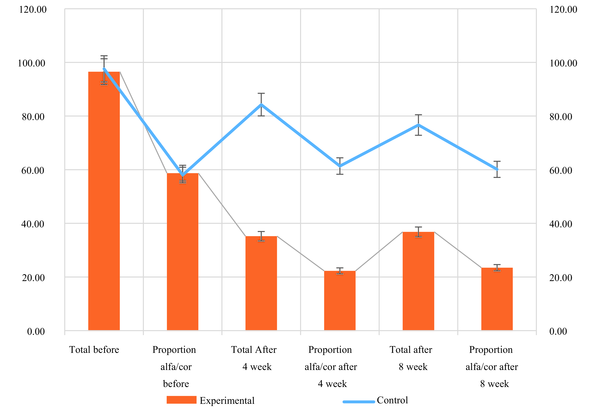
5. Discussion
The initial purpose of this clinical trial was to evaluate variations in EEG activity over an 8-week period of NFB training using a well-established theta/beta1 training NFB protocol. The overall outcome of the study provided positive results in support of our hypothesis. Specifically, we anticipated observing a significant improvement in the beta1 band and a reduction in the theta band compared to baseline levels. An interesting finding of this trial was that even after 4 weeks of treatment, participants reported a reduction in symptoms. This finding can indicate the early and cost-effective effect of neurofeedback, which can be investigated in future studies. Additionally, the experimental group exhibited a one-third decrease in the ratio of alpha-amylase to cortisol. It is logical to ascribe these variations to the NFB sessions, as no other interventions were administered to the participants during the course of the experiment. While it is acknowledged that other factors may have influenced the group differences, it is important to note that the experimental group actively engaged in training and received regular supervision from the researcher(s) during the neurofeedback sessions, whereas the control group did not experience these same conditions.
In terms of interpretability, the study results were positive. Neurofeedback training at C4 not only impacted EEG bands but also had a significant influence on all visible outcomes and physiological biomarkers that were previously presented as impaired by the participant. Specifically, anxiety levels, as assessed by the DASS-21, cortisol, and alpha-amylase, exhibited significant reductions. These findings align with previous reports (13, 19, 25, 33, 41) regarding the efficacy of NFB training in anxiety reduction.
Furthermore, the findings of this study support the neurobiological foundation of emotional regulation (17, 25, 42) and provide justification for the application and effectiveness of the theta/beta1 protocol not only for patients with attention deficit disorder/attention deficit hyperactivity disorder (ADD/ADHD), as suggested by existing literature but also for the treatment of anxiety (13, 25) Previous studies that included control samples demonstrated an inhibition-regulation mechanism exerted by the cortex on extensive brain structures. The authors recommended that training in the beta1 band may serve to increase cortical excitation in under-aroused students (8, 14, 43).
Furthermore, individuals with mood and anxiety symptoms tend to exhibit hypoactivity in the rostral cingulate during tasks involving emotional processing or distraction, particularly when facing extensive interference from emotional distractors (26). Notably, our findings propose that the education of the aforementioned EEG bands can be beneficial in reducing anxiety in patients. Prior authors (44) presented that people suffering from chronic anxiety, with abnormally low levels of beta and alpha activity and abnormally high levels of beta2 (12.5 - 30 Hertz), demonstrated significant improvements in attention following NFB. Clearly, the enhancement of the beta1 (12.5 - 16 Hertz) band and the inhibition of theta activity emerged as significant positive differences in executive activity and decreased negative mood. Consequently, it can be inferred that the low power of fast brain waves may indicate diminished frontal cortical functions and damaged inhibitory function, which are acceptable to be affected in anxiety disorders (45).
The findings of the current study suggest similar effects. Along with the psychological tools and EEG variations, the reverse relationship presented in the present study between beta1 amplitude and cortisol levels demonstrates extensive reasons for the association between this hormone and cortical activity. The results of the current study further support the association between beta1 amplitude and cortisol levels, as evidenced by an inverse correlation. Indeed, lower beta1 amplitude was found to be associated with higher cortisol levels (46). This finding may be indicative of elevated levels of anxiety. However, this particular association could not be firmly established in the present trial, perhaps due to the precise measurements employed.
To summarize, our results provide additional support for previous evidence suggesting that NFB training targeting fast-wave activity can lead to improvements at neuroendocrine, behavioral, and executive levels. It is postulated that this training exerts a regulatory effect on overactive subcortical regions that are related to anxiety and emotional vulnerability. Nevertheless, further exploration of this mechanism is warranted in later studies, particularly by assessing cortisol amounts in hair, which would provide information on cortisol concentration and the participants' status over the preceding three months (47).
As far as we remember, this is the first clinical trial to assess the effect of NFB on psychological and hormonal variables. However, it is essential to acknowledge that the study had some limitations, including the absence of a no-treatment concurrent control group and the lack of blinding due to subjects and trainers being aware of subject allocation to either the experimental or control group. Despite these limitations, the study employed objective outcome measures and ensured the blinding of the researchers conducting assessments and analyzing the data. There is a clear need for more focused neuroscientific research to explore the clinical efficacy of NFB interventions in stress management, with a particular focus on the detailed characterization of stress levels ranging from mild to moderate to severe.
One significant limitation of this article lies in the high dropout rate among participants, which can be attributed to the nature of the study and the measurement of salivary cortisol levels. Also, the researchers should be cautious about assuming causation based solely on correlation. It is worth noting that the sample size was carefully selected to minimize the potential impact on the obtained results, considering this issue. Furthermore, further research is warranted to investigate other NFB protocols that could potentially become established as safe and effective interventions. Though the current findings must be interpreted with caution due to potential errors, it is important to highlight the need for larger sample sizes to allow for a more robust statistical analysis of the effects.
References
-
1.
Nooripour R, Hosseinian S, Ghanbari N, Haghighat S, Matacotta JJ, Gasparri ML. Validation of the Persian version of the satisfaction with life scale (SWLS) in Iranian women with breast Cancer. Curr Psychol. 2021;42(4):2993-3000. https://doi.org/10.1007/s12144-021-01662-2.
-
2.
Nooripour R, Hosseinian S, Hussain AJ, Annabestani M, Maadal A, Radwin L, et al. Correction to: How Resiliency and Hope Can Predict Stress of Covid-19 by Mediating Role of Spiritual Well-being Based on Machine Learning. J Relig Health. 2021;60(4):2322-3. [PubMed ID: 33464433]. [PubMed Central ID: PMC7814856]. https://doi.org/10.1007/s10943-021-01180-2.
-
3.
Nooripour R, Bass CK, Apsche J. Effectiveness of quality of life therapy aimed at improving sexual self-efficacy and marital satisfaction in addict couples of treatment period. Int J Behav Consult Ther. 2013;8(2):26-9. https://doi.org/10.1037/h0100973.
-
4.
Carneiro D, Castillo JC, Novais P, Fernández-Caballero A, Neves J. Multimodal behavioral analysis for non-invasive stress detection. Expert Syst Appl. 2012;39(18):13376-89. https://doi.org/10.1016/j.eswa.2012.05.065.
-
5.
Gartner M, Rohde-Liebenau L, Grimm S, Bajbouj M. Working memory-related frontal theta activity is decreased under acute stress. Psychoneuroendocrinology. 2014;43:105-13. [PubMed ID: 24703176]. https://doi.org/10.1016/j.psyneuen.2014.02.009.
-
6.
Morgado P, Cerqueira JJ. Editorial: The Impact of Stress on Cognition and Motivation. Front Behav Neurosci. 2018;12:326. [PubMed ID: 30622462]. [PubMed Central ID: PMC6308156]. https://doi.org/10.3389/fnbeh.2018.00326.
-
7.
Yadav S, Waldeck-Weiermair M, Spyropoulos F, Bronson R, Pandey AK, Das AA, et al. Sensory ataxia and cardiac hypertrophy caused by neurovascular oxidative stress in chemogenetic transgenic mouse lines. Nat Commun. 2023;14(1):3094. [PubMed ID: 37248315]. [PubMed Central ID: PMC10227029]. https://doi.org/10.1038/s41467-023-38961-0.
-
8.
Gadea M, Alino M, Hidalgo V, Espert R, Salvador A. Effects of a single session of SMR neurofeedback training on anxiety and cortisol levels. Neurophysiol Clin. 2020;50(3):167-73. [PubMed ID: 32279927]. https://doi.org/10.1016/j.neucli.2020.03.001.
-
9.
Goldin PR, McRae K, Ramel W, Gross JJ. The neural bases of emotion regulation: reappraisal and suppression of negative emotion. Biol Psychiatry. 2008;63(6):577-86. [PubMed ID: 17888411]. [PubMed Central ID: PMC2483789]. https://doi.org/10.1016/j.biopsych.2007.05.031.
-
10.
Johnson R, Vishwakarma K, Hossen MS, Kumar V, Shackira AM, Puthur JT, et al. Potassium in plants: Growth regulation, signaling, and environmental stress tolerance. Plant Physiol Biochem. 2022;172:56-69. [PubMed ID: 35032888]. https://doi.org/10.1016/j.plaphy.2022.01.001.
-
11.
Faria D, Moll-Bernardes R, Testa L, Moniz CMV, Rodrigues EC, Mota JM, et al. Neurovascular and hemodynamic responses to mental stress and exercise in severe COVID-19 survivors. Am J Physiol Regul Integr Comp Physiol. 2023;325(3):R269-79. [PubMed ID: 37449870]. [PubMed Central ID: PMC10625836]. https://doi.org/10.1152/ajpregu.00111.2023.
-
12.
Youwakim J, Vallerand D, Girouard H. Neurovascular Coupling in Hypertension Is Impaired by IL-17A through Oxidative Stress. Int J Mol Sci. 2023;24(4). [PubMed ID: 36835372]. [PubMed Central ID: PMC9967204]. https://doi.org/10.3390/ijms24043959.
-
13.
Arnsten AF. Stress weakens prefrontal networks: molecular insults to higher cognition. Nat Neurosci. 2015;18(10):1376-85. [PubMed ID: 26404712]. [PubMed Central ID: PMC4816215]. https://doi.org/10.1038/nn.4087.
-
14.
Zimmer C, Woods HA, Martin LB. Information theory in vertebrate stress physiology. Trends Endocrinol Metab. 2022;33(1):8-17. [PubMed ID: 34750063]. https://doi.org/10.1016/j.tem.2021.10.001.
-
15.
Gruzelier JH. Differential effects on mood of 12-15 (SMR) and 15-18 (beta1) Hz neurofeedback. Int J Psychophysiol. 2014;93(1):112-5. [PubMed ID: 23357178]. https://doi.org/10.1016/j.ijpsycho.2012.11.007.
-
16.
Cantave CY, Ouellet-Morin I, Giguere CE, Lupien SJ, Juster RP, Geoffrion S, et al. The Association of Childhood Maltreatment, Sex, and Hair Cortisol Concentrations With Trajectories of Depressive and Anxious Symptoms Among Adult Psychiatric Inpatients. Psychosom Med. 2022;84(1):20-8. [PubMed ID: 34596058]. https://doi.org/10.1097/PSY.0000000000001016.
-
17.
Knyazev GG. Motivation, emotion, and their inhibitory control mirrored in brain oscillations. Neurosci Biobehav Rev. 2007;31(3):377-95. [PubMed ID: 17145079]. https://doi.org/10.1016/j.neubiorev.2006.10.004.
-
18.
Micoulaud-Franchi JA, McGonigal A, Lopez R, Daudet C, Kotwas I, Bartolomei F. Electroencephalographic neurofeedback: Level of evidence in mental and brain disorders and suggestions for good clinical practice. Neurophysiol Clin. 2015;45(6):423-33. [PubMed ID: 26553293]. https://doi.org/10.1016/j.neucli.2015.10.077.
-
19.
Banerjee S, Argaez C. Neurofeedback and Biofeedback for Mood and Anxiety Disorders: A Review of Clinical Effectiveness and Guidelines. Ottawa (ON): Canadian Agency for Drugs and Technologies in Health; 2017. eng.
-
20.
Taheri Z, Tanha Z, Amraee K, Hassanvandi S. [Determining the Causal Relationships between the Brain-behavioral System and Psychological Vulnerability in Female Patients with Diabetes Based on the Mediator Role of Anxiety Sensitivity]. J Nurs Educ. 2023;12(4):44-55. Persian.
-
21.
Rahmani M, Mahvelati A, Farajinia AH, Shahyad S, Khaksarian M, Nooripour R, et al. Comparison of Vitamin D, Neurofeedback, and Neurofeedback Combined with Vitamin D Supplementation in Children with Attention-Deficit/Hyperactivity Disorder. Arch Iran Med. 2022;25(5):285-393. [PubMed ID: 35943003]. https://doi.org/10.34172/aim.2022.47.
-
22.
Soroush-Vala A, Rahmanian M, Jadidi M, Hassanvandi S. Application of Neurofeedback in Treating Epilepsy: A Systematic Review and Meta-Analysis. Int. J Body Mind Cult. 2023;10(2). https://doi.org/10.22122/ijbmc.v10i2.506.
-
23.
Hasslinger J, Jonsson U, Bolte S. Immediate and Sustained Effects of Neurofeedback and Working Memory Training on Cognitive Functions in Children and Adolescents with ADHD: A Multi-Arm Pragmatic Randomized Controlled Trial. J Atten Disord. 2022;26(11):1492-506. [PubMed ID: 35034510]. [PubMed Central ID: PMC9277335]. https://doi.org/10.1177/10870547211063645.
-
24.
Herrmann CS, Struber D, Helfrich RF, Engel AK. EEG oscillations: From correlation to causality. Int J Psychophysiol. 2016;103:12-21. [PubMed ID: 25659527]. https://doi.org/10.1016/j.ijpsycho.2015.02.003.
-
25.
Aliño Costa M, Gadea M, Hidalgo V, Pérez V, Sanjuán J. An Effective Neurofeedback Training, with Cortisol Correlates, in a Clinical Case of Anxiety. Univ Psychol. 2017;15(5). https://doi.org/10.11144/Javeriana.upsy15-5.entc.
-
26.
Etkin A, Egner T, Peraza DM, Kandel ER, Hirsch J. Resolving emotional conflict: a role for the rostral anterior cingulate cortex in modulating activity in the amygdala. Neuron. 2006;51(6):871-82. [PubMed ID: 16982430]. https://doi.org/10.1016/j.neuron.2006.07.029.
-
27.
Seo JS, Wei J, Qin L, Kim Y, Yan Z, Greengard P. Cellular and molecular basis for stress-induced depression. Mol Psychiatry. 2017;22(10):1440-7. [PubMed ID: 27457815]. [PubMed Central ID: PMC5269558]. https://doi.org/10.1038/mp.2016.118.
-
28.
Luo Q, Chen J, Li Y, Wu Z, Lin X, Yao J, et al. Altered regional brain activity and functional connectivity patterns in major depressive disorder: A function of childhood trauma or diagnosis? J Psychiatr Res. 2022;147:237-47. [PubMed ID: 35066292]. https://doi.org/10.1016/j.jpsychires.2022.01.038.
-
29.
Moradi A, Pouladi F, Pishva N, Rezaei B, Torshabi M, Mehrjerdi ZA. Treatment of Anxiety Disorder with Neurofeedback: Case Study. Procedia Soc Behav Sci. 2011;30:103-7. https://doi.org/10.1016/j.sbspro.2011.10.021.
-
30.
Schmitt AP. Resting-state functional connectivity in adolescents experiencing subclinical and clinical symptoms of depression: a mini-review of recent evidence. J Neurophysiol. 2022;127(1):146-9. [PubMed ID: 34879212]. https://doi.org/10.1152/jn.00327.2021.
-
31.
Rahmani E, Mahvelati A, Alizadeh A, Mokhayeri Y, Rahmani M, Zarabi H, et al. Is neurofeedback effective in children with ADHD? A systematic review and meta-analysis. Neurocase. 2022;28(1):84-95. [PubMed ID: 35068368]. https://doi.org/10.1080/13554794.2022.2027456.
-
32.
Hassanvandi S, Mohammadi MT, Shahyad S. Predicting the Severity of COVID-19 Anxiety Based on Sleep Quality and Mental Health in Health Care Workers. Novel Clin Med. 2022;1(4):184-91. https://doi.org/10.22034/NCM.2022.344007.1048.
-
33.
Allen AP, Kennedy PJ, Cryan JF, Dinan TG, Clarke G. Biological and psychological markers of stress in humans: focus on the Trier Social Stress Test. Neurosci Biobehav Rev. 2014;38:94-124. [PubMed ID: 24239854]. https://doi.org/10.1016/j.neubiorev.2013.11.005.
-
34.
Nooripour R, Hosseinian S, Sobhaninia M, Ghanbari N, Hassanvandi S, Ilanloo H, et al. Predicting Fear of COVID-19 Based on Spiritual Well-being and Self-efficacy in Iranian University Students by Emphasizing the Mediating Role of Mindfulness. Pract Clin Psychol. 2022;10(1):1-10. https://doi.org/10.32598/jpcp.10.1.288.6.
-
35.
Bennett CN, Gupta RK, Prabhakar P, Christopher R, Sampath S, Thennarasu K, et al. Clinical and Biochemical Outcomes Following EEG Neurofeedback Training in Traumatic Brain Injury in the Context of Spontaneous Recovery. Clin EEG Neurosci. 2018;49(6):433-40. [PubMed ID: 29268620]. https://doi.org/10.1177/1550059417744899.
-
36.
Rahmani E, Rahmanian M, Mansouri K, Mokhayeri Y, Jamalpour Y, Hassanvandi S. Are There any Possible Side Effects of Neurofeedback? A Systematic Literature Review and Meta-analysis. Iran J Psychiatry Behav Sci. 2023;17(3). e138064. https://doi.org/10.5812/ijpbs-138064.
-
37.
Bokma WA, Zhutovsky P, Giltay EJ, Schoevers RA, Penninx B, van Balkom A, et al. Predicting the naturalistic course in anxiety disorders using clinical and biological markers: a machine learning approach. Psychol Med. 2022;52(1):57-67. [PubMed ID: 32524918]. [PubMed Central ID: PMC8711102]. https://doi.org/10.1017/S0033291720001658.
-
38.
Putman P, van Peer J, Maimari I, van der Werff S. EEG theta/beta ratio in relation to fear-modulated response-inhibition, attentional control, and affective traits. Biol Psychol. 2010;83(2):73-8. [PubMed ID: 19897008]. https://doi.org/10.1016/j.biopsycho.2009.10.008.
-
39.
Le MTH, Tran TD, Holton S, Nguyen HT, Wolfe R, Fisher J. Reliability, convergent validity and factor structure of the DASS-21 in a sample of Vietnamese adolescents. PLoS One. 2017;12(7). e0180557. [PubMed ID: 28723909]. [PubMed Central ID: PMC5516980]. https://doi.org/10.1371/journal.pone.0180557.
-
40.
Kvamme TL, Sarmanlu M, Bailey C, Overgaard M. Neurofeedback Modulation of the Sound-induced Flash Illusion Using Parietal Cortex Alpha Oscillations Reveals Dependency on Prior Multisensory Congruency. Neuroscience. 2022;482:1-17. [PubMed ID: 34838934]. https://doi.org/10.1016/j.neuroscience.2021.11.028.
-
41.
Chattarji S, Tomar A, Suvrathan A, Ghosh S, Rahman MM. Neighborhood matters: divergent patterns of stress-induced plasticity across the brain. Nat Neurosci. 2015;18(10):1364-75. [PubMed ID: 26404711]. https://doi.org/10.1038/nn.4115.
-
42.
Qi M, Gao H, Guan L, Liu G, Yang J. Subjective Stress, Salivary Cortisol, and Electrophysiological Responses to Psychological Stress. Front Psychol. 2016;7:229. [PubMed ID: 26925026]. [PubMed Central ID: PMC4757705]. https://doi.org/10.3389/fpsyg.2016.00229.
-
43.
Schutter DJ, Knyazev GG. Cross-frequency coupling of brain oscillations in studying motivation and emotion. Motiv Emot. 2012;36(1):46-54. [PubMed ID: 22448078]. [PubMed Central ID: PMC3294206]. https://doi.org/10.1007/s11031-011-9237-6.
-
44.
Tinaz S, Kamel S, Aravala SS, Elfil M, Bayoumi A, Patel A, et al. Neurofeedback-guided kinesthetic motor imagery training in Parkinson’s disease: Randomized trial. medRxiv. 2022;Preprint. https://doi.org/10.1101/2022.01.05.22268816.
-
45.
Saul MA, He X, Black S, Charles F. A Two-Person Neuroscience Approach for Social Anxiety: A Paradigm With Interbrain Synchrony and Neurofeedback. Front Psychol. 2021;12:568921. [PubMed ID: 35095625]. [PubMed Central ID: PMC8796854]. https://doi.org/10.3389/fpsyg.2021.568921.
-
46.
Kivimaki M, Steptoe A. Effects of stress on the development and progression of cardiovascular disease. Nat Rev Cardiol. 2018;15(4):215-29. [PubMed ID: 29213140]. https://doi.org/10.1038/nrcardio.2017.189.
-
47.
Rogala J, Jurewicz K, Paluch K, Kublik E, Cetnarski R, Wrobel A. The Do's and Don'ts of Neurofeedback Training: A Review of the Controlled Studies Using Healthy Adults. Front Hum Neurosci. 2016;10:301. [PubMed ID: 27378892]. [PubMed Central ID: PMC4911408]. https://doi.org/10.3389/fnhum.2016.00301.