Abstract
Keywords
Prostate cancer Alkaloids Delphinium cytotoxicity Apoptosis mitochondrial pathway
Introduction
Prostate gland cancer is considered one of the common cancers with a high mortality rate in men (1, 2). A large number of patients suffer from drug resistance or resistance to their primary hormonal treatment (2). Therefore, there is always a necessity to investigate new methods for its treatment or to discover new herbal medications for alleviating cancer symptoms. In recent years, Taxus brevifolia, Catharanthusroseus and Combretumcaffrum were successfully studied to discover biomolecules in the treatment of anti-cancer drugs (3). These plants showed prominent anti-tumor properties mediated by different cellular pathways to induce cancer cell apoptosis or proliferation arrest (3, 4).
The genus Delphinium sp. (larkspur) belongs to the family Ranunculaceae which is rich in complex diterpene or norditerpene alkaloids with a variant range of toxicity (5). Plant of this genus has limited application as herbal medicines in the treatment of inflammatory disorders like jaundice, rheumatism, toothache, neuralgia (5), and cancer treatment (6). In recent years, a different class of compounds including flavonols, anthocyanins, and alkaloids were isolated from these species from which diterpene alkaloids were more interesting to discover anti-cancer agents (5, 7-9). Interestingly, several reports on the antiproliferative properties of the diterpenoid alkaloids isolated from Delphinium species against cancer cells have appeared in recent years. Progress in this field was started in 2010 by Gao`s group, which reported significant cytotoxic activity of Atisine-type alkaloids from D. chrysotrichum against the A549 cell line (10). In 2011, C-20 alkaloids isolated from D. honanense were reported with potent cytotoxic activity against the breast cancer cells (11). In 2014, hetisine-type C20-diterpenoid alkaloids isolated from D. Trichophorum showed prominent cytotoxic activities against A549 cancer cells (12). Therefore, in continuation of recent studies in cancer drug discovery on Delphinium species, we selected D. semibarbatum to check the cytotoxicity and apoptosis properties of alkaloid fractions of this plant against prostate cancer cells. In Iran, D. semibarbatum knowns as yellow larkspur grows wild in the northeast part of the country. D. semibarbatum was previously known as a synonym of D. zalil, but Sharifnia et al. introduced it as a new species (13). Although anticancer and pharmacological properties of a number of the delphinium species are reported, there is no study on the anticancer effects of D. semibarbatum alkaloid contents.
Experimental
Materials
We purchased JC-1 dye from Sigma Aldrich (St. Louis, MO, USA), an apoptosis kit from Abcam (Cambridge, United Kingdom), and a fluorescent ROS detection kit (Marker GeneTM live cell) from Marker Gene Technologies (Marker Gene Technologies, Inc. Eugene, USA). A colorimetric assay kit for analyses of the caspase-3 enzyme was purchased from an R&D systems corporation (Minneapolis, USA). Sc‐7480 (anti‐Bax), sc‐7382 (anti‐Bcl2), and sc‐47724 as GADPH antibodies were provided from Santa Cruz Biotechnology (CA, USA). DMEM and FBS were purchased from Gibco Invitrogen (Grand Island, NY, USA).
Plant Material
D. semibarbatum flowering aerial parts were collected in the proximity of Mozdooran Mountains, located between Mashhad and Sarakhs cities, North Khorasan Province, Iran. It was determined by Mohammad Reza Jouharchi, Mashhad University of Sciences, and the voucher specimen (SAM-3392) was stored in the Samsam Shariat Herbarium, Isfahan University of Medical Science.
Extraction and Isolation
Plant material (5000 g) was air-dried at room temperature, powdered, and extracted by ethanol 95% containing 1% trifluoroacetic acid (TFA) with constant shaking for three days and by three times. The acidified ethanol extract was filtered, concentrated by a rotary evaporator, and stored in the refrigerator. To remove fats and chlorophylls, the concentrated extract was filtered through a C-18 cartridge, using ethanol: water: TFA (70:29:1) as solvent. The defatted extract was dried and submitted on a silica gel column using chloroform: methanol (100:0; 95:5; 90:10: 80:20; 70:30; 60:40) as the eluting solvent. Fractions were checked on TLC for alkaloid content using Dragendorff’s reagent. Alkaloid fractions (DSAFs) labeled as Da, Ea, and Eb, and were selected for biological tests against prostate cancer cells.
Cell Culture
We prepared DU‐145 and LNCaP (C428 and C439) human prostate cancer cells from the Pasteur Institute of Iran. Cells were cultured at DMEM medium contained 10% FBS, penicillin + streptomycin (100 U/mL+ 100 μg/mL), and maintained in the incubator (37 °C, 5% CO2, and 95% humidity).
Cell Viability Assay
DU‐145 and LNCaP prostate cancer cells (5000 cells in each well) were seeded in a 96-well plate. After overnight incubation at 37 °C, they were treated with alkaloid fractions in different concentrations of 0.1, 1, 10, 100, 250, 500, and 1000 µg/mL, while the vehicle for each concentration was used as the negative control. After 48 h, MTT solution (0.5 mg/mL, 20 µL) was added to the wells, shacked, and were incubated again at 37 °C, for 4 more h. Then, the supernatants were discarded, DMSO (100 µL) was added, and the optical density was determined at 570 nm by the BioTek microplate reader (Winooski, VT, USA) (14, 15).
Flow Cytometry Assay with an Apoptosis Kit
Flow cytometry assay was done as mentioned previously (16). Briefly, we seeded DU‐145 and LNCaP cells (5000 cells/well) in a 6-well plate and incubated them overnight. It was treated with DSAFs (10, 100, and 1000 µg/ml) for 48 h, stained with annexin V-FITC (5 µL) and PI (50 ng/mL, 1 µL), and incubated for 15 min in the dark. Finally, plates were analyzed by Bioscience FACS Calibur flow cytometer (Franklin Lakes, NJ, USA).
Western Blot Analysis of Apoptotic Related Proteins
The expression of Bax and Bcl-2 was detected by western blot analysis as reported previously (16). DU‐145 and LNCaP cancer cells were seeded (5000 cells in each well) into the 6-well plates and treated with alkaloid fractions (10, 100, and 1000 µg/ml) for two days. After 48 h, we lysed cells with R0278 RIPA buffer containing P7626 PMSF (0.5 mM) and 0.5% P8340 protease inhibitor cocktails (Sigma Aldrich, MO, USA). Then, protein contents of tested samples were determined by the Bradford reagent and equal amounts of proteins (30–50 μg) were resolved by 12% SDS-PAGE and transferred to PVDF membranes (Amersham Pharmacia Biotech). The membranes were incubated with indicated primary antibodies at 4 °C overnight (Bcl-2, Bax, and GADPH, each 1:1000 dilution) followed by another incubation with horseradish peroxidase HRP-conjugated antibodies at room temperature for 2 h. Protein blots were developed by an ECL detection reagent (Amersham Pharmacia Biotech). Finally, each of the western blot bands was analyzed by image j and normalized based on the GAPDH.
Caspase Activity Assay
Caspase activity was done as it was reported before (16). Briefly, cells were cultured overnight and treated with DSAFs concentrations (1-1000 µg/mL) for 48 h. Then, we lysed the treated cells, collected the supernatants, and checked them for protease activity. Protease activity was checked by the caspase-specific substrate peptide conjugated with p-nitroanaline as the color reporter. After 1-hour incubation at 37 °C, the amount of released p-nitroaniline was determined at 405 nm.
Mitochondrial Membrane Potential Assay
The potential of the mitochondrial membrane was detected using the JC-1 fluorescent probe, which can enter the mitochondria matrix based on the level of ΔΨm. We treated the cells (5 × 103 cells/well) with DSAFs at the concentrations of 1, 10, 100, 500, and 1000 µg/mL. After 48 h, supernatants were discarded and the media were replaced with HEPES buffer (40 mM, pH 7.4) containing JC-1 (2.5 mM) for 30 min at 37 °C. In the end, the fluorescence intensity of the wells was detected at two levels of excitation (490/540 nm)/emission (540/590 nm) wavelengths using BioTek fluorescence Microplate Reader (VT, USA). The difference between 590 and 540 nm fluorescence readings was reported as ΔΨm (17).
Intracellular ROS Generation Assay
Generation of reactive oxygen species was analyzed with dichlorofluorescein diacetates (DCFH-DA) as fluorescent probes, which were described previously (17). DU‐145 and LNCaP cells were seeded in a black 384-well plate and treated with DSAFs at concentrations of 1, 10, 100, 500, and 1000 µg/mL for 48 h. Subsequently, they were incubated with DCFH-DA (20 µM) in HEPES (N-2-hydroxyethylpiperazine-N’-2-ethanesulfonic acid) buffer (40 mmol/L, pH 7.4) for 30 minutes at 37 °C in the dark place, and washed with HEPES buffer. The fluorescence intensity was determined at both the excitation and the emission wavelengths of 485 and 528 nm.
Statistical Analyses
Results were expressed as mean ± SD. Statistical analysis was done by one-way ANOVA analysis using graph pad software followed by Dunnett’s post-hoc test and P-value < 0.05 was considered a statistically significant difference.
Results
DSAFs Inhibit Cell Proliferation of Prostate Cancer Cell Lines
The inhibitory effect of D.semibarbatum alkaloid fractions on the growth of prostate cancer cells was primarily evaluated by MTT assay. As shown in Figure 1, DSAFs treatment for 48 h potently suppressed prostate cancer cell growth, both in DU‐145 and LNCaP cells (P < 0.05). All the tested fractions inhibited the growth of DU‐145/LNCaP cells in a dose-dependent manner with IC50 values of Da: 62.33 ± 2.52/82.50 ± 3.53; Ea: 173.33 ± 15.27/75.80 ± 13.54; Eb: 98.33 ± 15.57/89.6 ± 6.22 varied from 62.33 –173.33/75.80 - 98.33 μg/mL, respectively. In comparison between fractions, Da exhibited higher cytotoxic effects in DU 145, and Ea in LNCaP treated cells (Figure 1).
DSAFs Induce Apoptosisin Prostate Cancer Cell Lines
We stained the treated cells by annexin V/PI and analyzed them by flow cytometry to determine the type of cell death. Staining was conducted to classify cells following treatment with DSAFs into three groups: viable (double negative stained; Q1), apoptotic (Annexin V-FITC+; Q2+Q3), and necrotic (Annexin V-FITC-/PI+; Q4) cells. As it can be seen in Figure 2, alkaloid fractions significantly increased the number of apoptotic cells, compared to the untreated cells in a dose-dependent manner (P < 0.05). Specifically, cell apoptosis increased in the range of 4.95 – 11.46% (Da), 8.01 – 67% (Ea), and 11.84 – 27.43% (Eb) for DU‐145 cells (Figure 2A) and 5.65 – 34.2% (Da), 20.05 – 32.36% (Ea), and 4.64 – 39.83% (Eb) for LNCaP cells (Figure 2B). At a higher concentration (1000 μg/mL), the highest apoptogenic activity was observed for fraction Ea against DU‐145 (67%). Other than apoptosis, necrotic death was also seen; but, the predominating cell death by DSAFs was through the increased population of apoptotic cells (Annexin V-positive) in a dose-dependent manner, especially in DU 145 cells.
Caspase-3 Activity in DSAFs Treated with DU‐145 and LNCaP Cells
To examine the downstream effectors in the apoptotic signaling pathway, activation of caspase-3 was examined by a colorimetric assay using specific chromophores. As shown in Figure 3, D.semibarbatum alkaloid fractions significantly, and dose-dependently enhanced caspase-3 activity in both cell lines (P < 0.05). According to these results, the Ea fraction showed more activity on caspase-3 than others. These data indicated that DSAFs induced apoptosis through the caspase pathway in both prostate cancer cells.
DSAF (Ea) Induces Depolarization of Mitochondrial Membrane Potential (ΔΨm)
As it is known, loss of ΔΨm is an important event in cell apoptosis. For further assessment on how apoptosis occurs in DSAFs treated cells, the mitochondrial membrane potential was analyzed by DSAF (Ea) as a selected fraction by employing a JC-1 fluorescent probe. As shown in Figure 4, a significant loss of ΔΨm occurred after treatment with various concentrations of Ea (1-1000 µg/mL) in a dose-dependent manner in DU‐145 and LNCaP cells (P < 0.05). These results confirmed that DSAF (Ea) induced apoptosis on DU‐145 and LNCaP cells after 48 h through the disruption of the mitochondrial membrane.
DSAF (Ea) Increases ROS Generation
To better characterize the pathway through which D.semibarbatum fractions exert apoptosis in prostate cancer cells, the impact of one of the fractions: Ea of D.semibarbatum on intracellular ROS generation was assessed with the specific DCFH-DA probe. After treatment with Ea for 48 h, a significant increase in ROS levels was observed in a dose‐dependent manner, starting from 50 µg/mL (P < 0.05, Figure 5). These data indicated that ROS overproduction is involved in induced apoptosis by fraction Ea.
Western Blot Analysis of the Anti-apoptotic and Pro-Apoptotic Proteins in DU‐145 and LNCaP Cells
Western blot analysis was employed to determine the effect of fraction Ea from D.semibarbatum on the expression of proteins involved in the mitochondria-mediated apoptosis. As shown in Figure 6, after 48 hof Ea treatment, the expression of Bcl-2 was downregulated, while the Bax was upregulated (P < 0.05). These results confirmed that Ea treatment induces apoptosis in DU‐145 and LNCaP cells via the activation of the mitochondrial pathway.
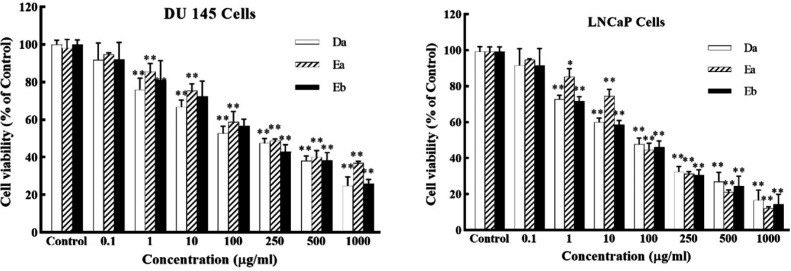
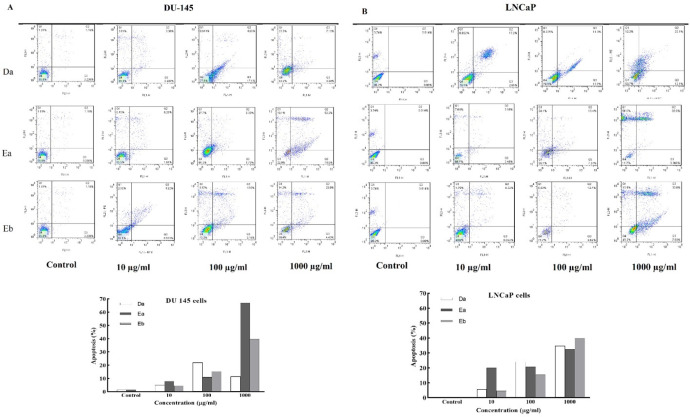
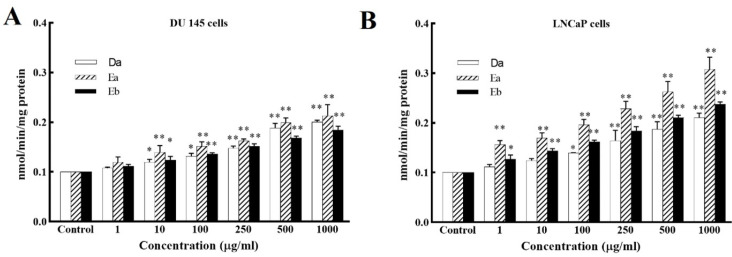
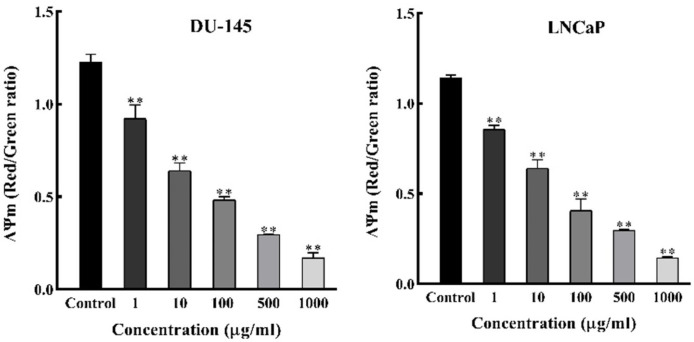
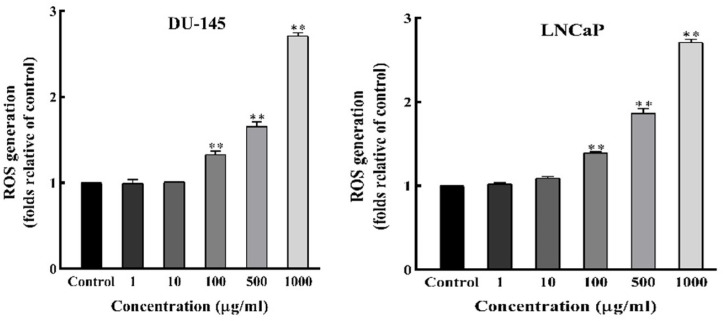
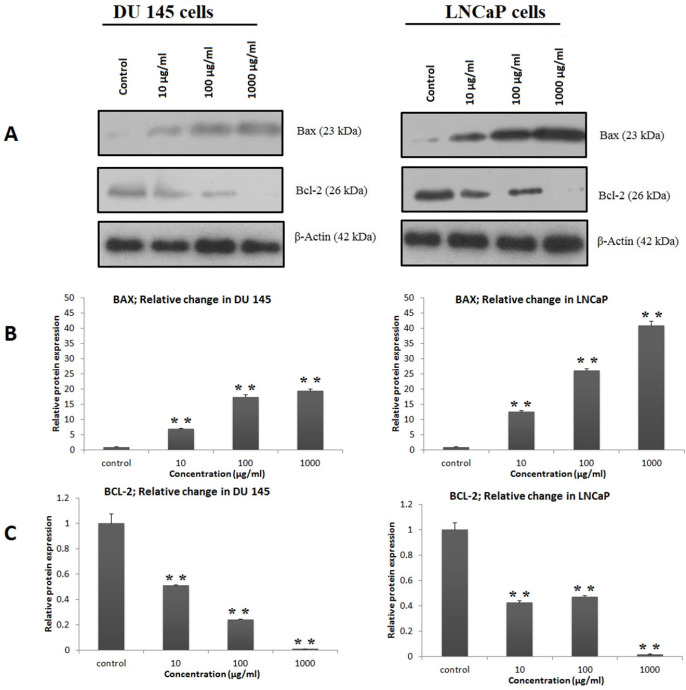
Discussion
This study examined the antiproliferative effect of D. semibarbatum against prostate cancer cells. The MTT assay showed significant antiproliferative effects against LNCaP and DU‐145 cells with higher suppressive activity against LNCaP cells.
Many of the anti-cancer agents can induce apoptosis (18). Since apoptosis is the most potent defense in multicellular organisms against cancer phenomena, the selective induction of apoptosis in tumor cells is a promising therapy approach (19, 20). Here, we demonstrated that compared to the untreated cells, DSAFs decreased cell viability through apoptosis in both LNCaP and DU‐145 prostate cancer cells. Apoptosis was confirmed by an increased population of the annexin positive stained cells in flow cytometry analysis using annexin V/PI. Our results indicated that DSAFs increased both early and late-stage apoptosis in a dose-dependent manner, with high apoptogenic activity at the higher concentrations.
Mitochondria’s role is important in regulating the pathways leading to apoptosis (21). Figure 5 clearly showed that DSAFs induced apoptosis concomitant with loss of ΔΨm in both cell lines. It indicated that anti-cancer activity is exerted by mitochondrial membrane depolarization, and possibly is related to the elevation of ROS levels (22). The inhibitory effects of some anti-tumor drugs are dependent on ROS generation (23). In the mitochondrial-dependent cell apoptosis, excessive ROS production can distract the mitochondria membrane, release apoptosis-inducing factors, and start the caspase cascades (22). Consistent with these results, and intracellular ROS overproduction, we proposed that DSAFs induce apoptosis via the mitochondria-dependent pathway by an increase in intracellular ROS and loss of ΔΨm, which consequently leads to a cascade of events driving to apoptosis (21).
In the mitochondrial pathway of apoptosis, relevant studies confirmed that a high Bax to Bcl-2 ratio is associated with the ΔΨm decrease following cytochrome c release (21). The facilitated release of cytochrome c from mitochondria into the cytosol, as mentioned before, induces caspase cascades which led to apoptosis (24). Therefore, we analyzed the expression of the Bax/ Bcl-2 ratio by western blot analysis and showed that DSAFs treatment shifts the Bax/Bcl-2 ratio in favor of apoptosis by a significant decrease in the anti-apoptotic Bcl-2, and increase of the pro-apoptotic Bax protein (Figure 4). The excess activation of the Bax/Bcl-2 ratio increases permeabilization of the mitochondrial outer membrane and consequently causes the release of other pro-apoptotic molecules, which induce apoptosis through caspase cascades (24). It was consistent with the caspase-3 activity results significantly increased by DSAFs in a dose-dependent manner (Figure 3).
Phytochemical analysis of DSAF (Ea) led to the isolation of one unprecedented C-20 hetisine type diterpene alkaloid as the major component which is reported elsewhere. In this agreement, Spiramine C–D from the hetisine-type diterpenoid alkaloids showed apoptosis activity in MCF-7 cells (25). Xiaoxia Liang et al. reported similar diterpene alkaloids with anti-cancer activities (26). Hazawa et al. reported that six hetisine-type derivatives showed significant cytotoxicity against the Raji and A549 cells (27). In another research surveyed by Xiangting Gao, aconitine with (C-19 diterpene alkaloid) was tested against H9c2 cardiac cells. Aconitine induced intrinsic apoptosis by upregulation of cytochrome c, Bax/Bcl-2 ratio, and caspase-3 activity (28).
Conclusion
Conclusively, the present study demonstrated that all the three alkaloidal fractions of D. semibarbatum exert cytotoxic activities, through apoptosis against LNCaP, and DU 145 cancer cells. Although other than apoptosis, necrotic death was also seen; but, the predominating cell death by DSAFs was through the increased population of apoptotic cells, especially in DU 145 cells. The apoptosis mechanism was investigated to be through the intrinsic pathway. The induction of apoptosis was found to be associated with increased ROS generation, disruption of mitochondrial membrane potential (MMP), and activation of caspase-3. Western blot analysis confirmed that treatment with DASF (Ea) in DU‐145 and LNCaP cells increases the Bax/Bcl-2 ratio through the release of BAX pro-apoptotic, and decreasing Bcl-2 anti-apoptotic proteins.
Acknowledgements
References
-
1.
Ferlay J, Soerjomataram I, Dikshit R, Eser S, Mathers C, Rebelo M, Parkin DM, Forman D, Bray F. Cancer incidence and mortality worldwide: sources, methods and major patterns in GLOBOCAN 2012. Int. J. Cancer. 2015;136:359-86.
-
2.
Siegel RL, Miller KD, Jemal A. Cancer statistics, 2016. CA: Cancer J. Clin. 2016;66:7-30. [PubMed ID: 26742998].
-
3.
Safarzadeh E, Sandoghchian Shotorbani S, Baradaran B. Herbal medicine as inducers of apoptosis in cancer treatment. Adv. Pharm. Bull. 2014;4:421-7. [PubMed ID: 25364657].
-
4.
Wang CZ, Calway T, Yuan CS. Herbal medicines as adjuvants for cancer therapeutics. Am. J. Chin. Med. 2012;40:657-69. [PubMed ID: 22809022].
-
5.
Hao DC, Xiao PG, Ma HY, Peng Y, He CN. Mining chemodiversity from biodiversity: pharmacophylogeny of medicinal plants of Ranunculaceae. Chin. J. Nat. Med. 2015;13:507-20. [PubMed ID: 26233841].
-
6.
Bussa NF, Belayneh A. Traditional medicinal plants used to treat cancer, tumors and inflammatory ailments in Harari Region, Eastern Ethiopia. S. Afr. J. Bot. 2019;122:360-8.
-
7.
Fang S, Benn M. Norditerpenoid alkaloids from seeds of Delphinium zalil. Phytochemistry. 1992;31:3247-50.
-
8.
Kolak U, Ozturk M, Ozgokce F, Ulubelen A. Norditerpene alkaloids from Delphinium linearilobum and antioxidant activity. Phytochemistry. 2006;67:2170-5. [PubMed ID: 16860354].
-
9.
Lin CZ, Zhao ZX, Xie SM, Mao JH, Zhu CC, Li XH, Zeren-dawa B, Suolang-qimei K, Zhu D, Xiong TQ, Wu AZ. Diterpenoid alkaloids and flavonoids from Delphinium trichophorum. Phytochemistry. 2014;97:88-95. [PubMed ID: 24256579].
-
10.
He YQ, Ma ZY, Wei XM, Du BZ, Jing ZX, Yao BH, Gao LM. Chemical constituents from Delphinium chrysotrichum and their biological activity. Fitoterapia. 2010;81:929-31. [PubMed ID: 20550959].
-
11.
He YQ, Ma ZY, Wei XM, Liu DJ, Du BZ, Yao BH, Gao LM. Honatisine, a novel diterpenoid alkaloid, and six known alkaloids from Delphinium honanense and their cytotoxic activity. Chem. Biodivers. 2011;8:2104-9. [PubMed ID: 22083922].
-
12.
Lin CZ, Zhao ZX, Xie SM, Mao JH, Zhu CC, Li XH, Zeren-dawa B, Suolang-qimei K, Zhu D, Xiong TQ, Wu AZ. Diterpenoid alkaloids and flavonoids from Delphinium trichophorum. Phytochemistry. 2014;97:88-95. [PubMed ID: 24256579].
-
13.
Sharifnia F, Barani M, Assadi M. Notes on some species of the genus Delphinium (Ranunculaceae) in Iran. Iran J. Bot. 2013;19:202-10.
-
14.
Aghaei M, Karami-Tehrani F, Panjehpour M, Salami S, Fallahian F. Adenosine induces cell-cycle arrest and apoptosis in androgen-dependent and -independent prostate cancer cell lines, LNcap-FGC-10, DU-145, and PC3. Prostate. 2012;72:361-75. [PubMed ID: 21656837].
-
15.
Tehranian N, Sepehri H, Mehdipour P, Biramijamal F, Hossein-Nezhad A, Sarrafnejad A, Hajizadeh E. Combination effect of PectaSol and Doxorubicin on viability, cell cycle arrest and apoptosis in DU-145 and LNCaP prostate cancer cell lines. Cell Biol. Int. 2012;36:601-10. [PubMed ID: 22214228].
-
16.
Keyvanloo Shahrestanaki M, Bagheri M, Ghanadian M, Aghaei M, Jafari SM. Centaurea cyanus extracted 13-O-acetylsolstitialin A decrease Bax/Bcl-2 ratio and expression of cyclin D1/Cdk-4 to induce apoptosis and cell cycle arrest in MCF-7 and MDA-MB-231 breast cancer cell lines. J. Cell Biochem. 2019;120:18309-19. [PubMed ID: 31161672].
-
17.
Mohammadi Pour P, Yegdaneh A, Aghaei M, Ali Z, Khan IA, Ghanadian M. Novel 16,17-epoxy-23-methylergostane derivative from Sinularia variabilis, a soft coral from the Persian Gulf, with apoptotic activities against breast cancer cell lines. Nat. Prod. Res. 2021;188:71-8.
-
18.
Frankfurt OS, Krishan A. Apoptosis-based drug screening and detection of selective toxicity to cancer cells. Anticancer Drugs. 2003;14:555-61. [PubMed ID: 12960740].
-
19.
Fulda S. Modulation of Apoptosis by Natural Products for Cancer Therapy. Planta. Med. 2010;76:1075-9. [PubMed ID: 20486070].
-
20.
Hamzeloo-Moghadam M, Aghaei M, Fallahian F, Jafari SM, Dolati M, Abdolmohammadi MH, Hajiahmadi S, Esmaeili S. Britannin, a sesquiterpene lactone, inhibits proliferation and induces apoptosis through the mitochondrial signaling pathway in human breast cancer cells. Tumor Biology. 2015;36:1191-8. [PubMed ID: 25342596].
-
21.
Poupel F, Aghaei M, Movahedian A, Jafari SM, Shahrestanaki MK. Dihydroartemisinin Induces Apoptosis in Human Bladder Cancer Cell Lines Through Reactive Oxygen Species, Mitochondrial Membrane Potential, and Cytochrome C Pathway. Int. J. Prev. Med. 2017;8:78.
-
22.
Wu CC, Bratton SB. Regulation of the intrinsic apoptosis pathway by reactive oxygen species. Antioxid Redox Signal. 2013;19:546-58. [PubMed ID: 22978471].
-
23.
Teppo HR, Soini Y, Karihtala P. Reactive Oxygen Species-Mediated Mechanisms of Action of Targeted Cancer Therapy. Oxid Med. Cell Longev. 2017;2017:1485283. [PubMed ID: 28698765].
-
24.
Wang B, Liu J, Gong Z. Resveratrol induces apoptosis in K562 cells via the regulation of mitochondrial signaling pathways. Int. J. Clin. Exp. Med. 2015;8:16926-33. [PubMed ID: 26629245].
-
25.
Yan C, Huang L, Liu HC, Chen DZ, Liu HY, Li XH, Zhang Y, Geng MY, Chen Q, Hao XY. Spiramine derivatives induce apoptosis of Bax−/−/Bak−/− cell and cancer cells. Bioorganic Med. Chem. Lett. 2014;24:1884-8.
-
26.
Liang X, Gao Y, Luan S. Two decades of advances in diterpenoid alkaloids with cytotoxicity activities. RSC Advances. 2018;8:23937-46.
-
27.
Hazawa M, Wada K, Takahashi K, Mori T, Kawahara N, Kashiwakura I. Suppressive effects of novel derivatives prepared from Aconitum alkaloids on tumor growth. Invest. New Drugs. 2008;27:111. [PubMed ID: 18551247].
-
28.
Gao X, Zhang X, Hu J, Xu X, Zuo Y, Wang Y, Ding J, Xu H, Zhu S. Aconitine induces apoptosis in H9c2 cardiac cells via mitochondriamediated pathway. Mol. Med. Rep. 2018;17:284-92. [PubMed ID: 29115599].