Abstract
Keywords
Alzheimer’s disease Amygdalin Cholinesterase inhibitors Neuroprotectivity P. armeniaca
Introduction
Alzheimer’s disease (AD) is an age-related progressive neurodegenerative disorder characterized by the deterioration of memory leading to remarkable multilateral cognitive disorders with significant impact on the quality of life (1). It is also known as the most common cause of dementia in old age and according to statistics, the number of patients with dementia was 47 million in 2015 and it will reach 131.5 million by 2050 (2, 3).
The reduction of the level of acetylcholine (ACh) in the cerebrospinal fluid, amyloid beta (βA) aggregation, irregular phosphorylation of tau proteins and subsequent formation of neurofibrils tangles in the hippocampal neurons, and oxidative stress have been involved in the pathogenesis of AD (4-7).
Cholinesterase enzymes (ChEs) including acetylcholinesterase (AChE) and butyrylcholinesterase (BChE) belonging to the alpha/beta-hydrolase fold family have different tissue distribution (8). AChE is known to be found in neurons, whereas BChE mostly exists in plaques and tangles in glial cells (7). At present, the most promising therapeutic strategy is the inhibition of ChEs to prevent ACh neurotransmitter breakdown in neurons and synaptic clefts leading to the increased level of ACh in the basal forebrain (4). In addition, recent study reported by Mueller et al. revealed diminished mortality in patients with AD using AChE inhibitors (9). Furthermore, recent studies confirmed the efficacy of selective BChE inhibitors to promote the level of ACh and improve cognitive function (10). However, non-cholinergic role of ChEs by augmenting the formation of βA plaques has absorbed lots of attention (10-12). Hence, inhibition of ChEs not only improves the cholinergic function but can also be considered as an efficient strategy for preventing βA formation. Also, ChEs inhibitors have been recently considered for the cognitive improvements of dementia resulting from other neurological conditions such as Lewy body disease, Huntington’s disease, multiple sclerosis (MS), subcortical vascular dementia, and Parkinson’s disease (13, 14).
There is no definite treatment for AD and current four FDA-approved drugs including donepezil, rivastigmine, galantamine, memantine only ameliorate symptoms and the first three are AChE inhibitors (AChEIs). However, lack of significant efficacy and different side effects such as gastrointestinal disturbances, insomnia, fatigue or depression led to the conduction of a wide range of research to develop efficient anti-AD agents based on the multifactorial nature of disease (15). In this regard, various medicinal plants have been investigated for their effectiveness in treating AD and memory deficits. It is predicted that herbal agents regulate disease progression apart from symptomatic treatment (16-19).
Prunus armeniaca L. known as apricot is a delicious stone fruit belonging to family Rosaceae, widely cultivated in the temperate regions such as Iran, Turkey, Pakistan, Iraq, Afghanistan, Syria, Russia, USA, Mediterranean countries, and central Asia. Among them, Iran and Turkey are the main producers in the world (20). In Iran, the fruit is grown throughout the country especially north-west where the climate is suitable for cultivating a highly desirable product. Apricot kernels contain various micronutrients and macronutrients such as fatty acids, sterol derivatives, polyphenols, carotenoids, volatile compounds, and cyanogenic glycosides (CNGs) (21). They are divided into two types of sweet and bitter kernels, depending on the amount of CNGs, mainly amygdalin (D-mandelonitrile-β-D-gentiobioside) which cause a bitter taste (22). They also have shown various pharmacological properties such as anticancer, antimicrobial, cardioprotective, hepatoprotective, antioxidant, anti-inflammatory, anti-amyloidogenic, neuroprotective, and neurotrophic activities (21, 23-25). Moreover, apricot kernels were recommended for the treatment of dementia and promotion of the memory in ITM (26).
In this study, according to our previous study on herbal anti-ChE agents and focusing on the efficient biological activity of apricot kernels which were supported by both classic medicine and ITM, anti-ChE and neuroprotective activities of different sweet and bitter apricot kernels’ extracts from north-west of Iran were investigated (27, 28).
Experimental
Plant materials
The fresh fruits (Prunus armeniaca L.) were collected from Ivand, a village in East Azerbaijan with coordinates (38°20′57″N, 46°07′01″E, at 1632.7m altitude above sea level) on 6 July 2016. They were fetched to the laboratory and the stones were removed. Then, they were identified by Prof. G.R. Amin and the voucher specimens PMP-750 and PMP-768 were deposited in the Herbarium of Faculty of Pharmacy, Tehran University of Medical Sciences, Tehran, Iran for sweet and bitter types, respectively.
Extraction
The sweet and bitter apricot kernels were shade-dried and then milled using a mortar and pestle, given codes S and B, respectively.
Petroleum ether extracts SO and BO: to extract fatty oils, 600 g of S and 130 g of B were separately macerated three times, each time with 3100 mL and 1100 mL petroleum ether, respectively for 72 h at room temperature. The solvent was concentrated under vacuum and the resulting oils (SO and BO) were stored at 20 °C. Also, the resulting residue in this step was dried at room temperature to give 342 g and 70 g solids S1 and B1, respectively which were used for further extractions including hydroalcoholic, ethanolic, and aqueous extractions.
Hydroalcoholic extracts HS1 and HB1: 300 g of S1 and 50 g of B1 were separately extracted three times with methanol-water (70:30 (v/v) with total volume (3700 mL) and (970 mL) respectively, by maceration for 72 h at room temperature. The extracts were filtered and centrifuged at 4000 rpm for 6 min (Heraeus Megafuge 1.0, England), concentrated using a rotary evaporator under vacuum (Heidolph, Heizbad Hei-VAP, Germany) at 20 °C, and finally freeze-dried (LTE science LTD, England) at 60 °C/10 μmHg for 24 h to obtain desired extracts HS1 and HB1 in 16.30% and 22.74%, respectively which were stored at 20 °C.
Aqueous extracts AS1 and AB1: 10 g of S1 and 10 g of B1 were separately boiled with 150 mL distilled water in a beaker for 10 minutes. Then, they were cooled and filtered. Each solid residue was re-extracted by 50 mL distilled water. They were filtered, centrifuged at 4000 rpm for 6 min, concentrated using a rotary evaporator under vacuum, freeze-dried, and stored at 20 °C to afford desired extracts of AS1 and AB1 in 27.5% and 24.2%, respectively.
Ethanolic extracts ES1 and EB1: 10 g of S1 and 10 g of B1 were separately extracted three times with 245 mL ethanol (96%) by maceration for 72 h at room temperature. Next, the collected solvents were filtered, centrifuged at 4000 rpm for 6 minutes, concentrated using a rotary evaporator under vacuum, freeze-dried to yield 7.3% and 6.9% ES1 and EB1, respectively which were stored at 20 °C. The same procedure was performed for S and B to afford ethanolic extracts of ES (13.2%) and EB (9.3%).
In-vitro AChE and BChE inhibition assays
Anti-AChE and anti-BChE activity of all extracts as well as amygdalin were investigated using modified Ellman’s method (29).
Electric eel (Torpedo Californica) AChE (E.C. 3.1.1.7, type VI-S, lyophilized powder), BChE (E.C.3.1.1.8, from equine serum), acetylthiocholine iodide, butyrylthiocholine iodide, 5, 5′-dithiobis (2-nitrobenzoic acid) (DTNB), amygdalin and rivastigmine were purchased from Sigma (Steinheim, Germany).
10 mg of aqueous extracts of P. armeniaca was dissolved in 1 mL distilled water and the other extracts were dissolved in a mixture of 50% DMSO and 50% methanol to obtain dilution of stock 10 mg/mL and afterwards four different concentrations of each extract were examined to get enzyme inhibition for AChE/BChE. In the case of amygdalin, the stock solution was prepared by dissolving 1 mg of that compound in 1 mL distilled water. In a 96 well plate, 50 µL phosphate buffer (0.1 M, pH 8.0), 25 µL of the sample solution, DMSO/MeOH or distilled water and 25 µL of enzyme [0.22 U/mL of AChE or BChE] was added. The plate was pre-incubated for 15 min at room temperature. Then, 125 µL of DTNB (3 mM in buffer), as well as 25 µL of substrate (3 mM in water) (acetylthiocholine iodide or butyrylthiocholine iodide) was added to each well. Finally, the change of absorbance was recorded at 405 nm after 20 min using a microplate reader (BioTek ELx808, USA).
Rivastigmine was applied as the positive control, while a mixture including buffer, DMSO, DTNB, and substrate was tested under the same conditions without enzyme as the negative control.
Neuroprotection study assays
Rat pheochromocytoma PC12 cell line was purchased from the Pasteur Institute (Tehran, IRAN) and culture media and supplements were purchased from Gibco (Paisley, UK). The cells were cultivated in DMEM supplemented with 10% fetal calf serum plus antibiotics (100 units/mL penicillin, 100 μg/mL streptomycin). To induce neuronal differentiation, PC12 cells were re-suspended using trypsin/EDTA (0.25%) and seeded in 96 well culture plate (4000cells/well) and cultured for 1 week in differentiation medium (DMEM + 2% h serum + NGF (100 ng/mL) + penicillin and streptomycin). To evaluate the effect of AS1 and AB1 as well as amygdalin on the survival rate of neurons, the culture medium was changed to NGF free medium and different concentrations of the above-mentioned extracts and amygdalin (1, 10, 100 μg/mL) were applied on cells. Quercetin (3 μg/mL) was applied as a positive control. The extracts and amygdalin were diluted in DMEM and added to each well in the volume of 10 μL. Then, after 3 h, induction of ROS mediated apoptosis was initiated by adding the H2O2 (400 μM) to their medium. After 12 h, MTT assay was performed (30). MTT solution (5 mg/mL) was added to each well in a volume of 10 μL, and 3.5 h later, 100 μL of the solubilisation solution (10% SDS in 0.01 M HCl (w/v) was added into each well. The plates were allowed to stand overnight in the incubator in a humidified atmosphere. Absorbance was measured at 570 nm with a reference wavelength of 630 nm using a plate reading spectrophotometer (BioTek ELx808, USA). Each experiment was performed in three replicates.
Determination of total phenols content
The total phenolic content of the aqueous extracts possessing anti-ChE activity was assessed using Folin-Ciocalteu assay (31). Folin-Ciocalteu (Steinheim, Germany) and gallic acid (GAE) (Darmstadt, Germany) were purchased from Sigma. The total phenolic content was expressed in gallic acid equivalents as mg per g extract. The calibration equation was (y = 0.0068x-0.0221) (R² = 0.9911) where y is the absorbance at 765 nm using an UV-Vis Spectrophotometer (Mecasys 2120uv, Korea) and x is the concentration of gallic acid mg/g extract.
Determination of total flavonoids content
Total flavonoid content in the aqueous extracts was determined by aluminum chloride colorimetric assay (32). Aluminum chloride and chatechin (CE) were purchased from Merk (Darmstadt, Germany) and Sigma (Darmstadt, Germany), respectively. Total flavonoid content of aqueous extracts was expressed as mg chatechin equivalents per g extract. The calibration equation was (y = 0.002x + 0.0227) (R² = 0.9975) where y is the absorbance at 510 nm and x is the concentration of chatechin mg/g extract.
Statisticalanalysis
All experiments were accomplished in triplicates. The IC50 values were estimated graphically from log inhibitor the extract concentration versus percent of inhibition by using Microsoft Excel 2010 program. One-way ANOVA was used to assess significant differences among the treatment groups followed by Tukey’s multiple comparisons test that was carried out to determine the level of significance by GraphPad Prism 6 software (San Diego, CA, USA). It means statistically significant when P value was less than 0.05.
Results
In-vitro anti-AChE and anti-BChE activity of different extracts of P. armeniaca
Anti-ChE activity of different extracts was evaluated comparing with rivastigmine as the reference drug (Table 1). Also, ChEI activity of amygdalin as one of the main constituents was investigated to demonstrate whether it is responsible for the corresponding inhibitory activity (33, 34) (Table 1).
As shown in Table1, AB1 illustrated the best anti-AChE activity with IC50 = 134.93 μg/mL whereas HB1, BO, EB1 and EB counterparts depicted no AChEI activity. Our results revealed that AS1 showed moderate inhibitory activity against AChE (IC50 = 356.75 μg/mL) and the corresponding HS1, SO, and ES extracts showed low activity with IC50s = 418.79, 481.42, and 445.65 μg/mL, respectively. It was found that ES1 and amygdaline showed no inhibitory activity against AChE and probably is not responsible for anti-AChE activity of apricot kernels.
It should be noted that all extracts as well as amygdalin showed no anti-BChE activity.
Neuroprotectivity against H2O2-induced cell death in PC12 neurons
Aqueous extracts of AB1 and AS1 were selected for the investigation of in-vitro neuroprotective studies caused by H2O2 in differentiated PC12 neuron cells.
Also, similar assay was conducted for amygdalin whether the neuroprotectivity was caused by amygdalin (Figure 1). As shown in Figure 1, the percentage of cell viabilities was measured at the concentrations of 1, 10, 100 µg/mL of those extracts in comparison to the H2O2-treated group as the control at the concentration of 3 µg/mL.
According to our results, PC12 cells pre-treated with AB1 significantly protected neurons against H2O2 at 10 and 100 µg/mL (cell viability = 70.49 and 81.47% with P-value < 0.0001). However, it depicted moderate neuroprotectivity against cell death at 1 µg/mL (cell viability = 60.54% with P-value < 0.01). It was found that aqueous extract of AS1 indicated remarkable neuroprotectivity at 100 µg/mL (cell viability = 70.71% with P-value < 0.0001). It exhibited no significant productivity at 1 and 10 µg/mL. Similar in-vitro evaluation of amygdalin at concentration of 1, 10, and 100 µg/mL showed no neuroprotectivity.
Determination of total phenolic and flavonoid content
Total phenolic and flavonoid content of aqueous extracts was reported in Table 2. As can be seen in Table 2, the extract of AS1 contained more total phenolic and flavonoids content than AB1 suggesting that AChEI activity is independent of phenolic and flavonoid compounds.
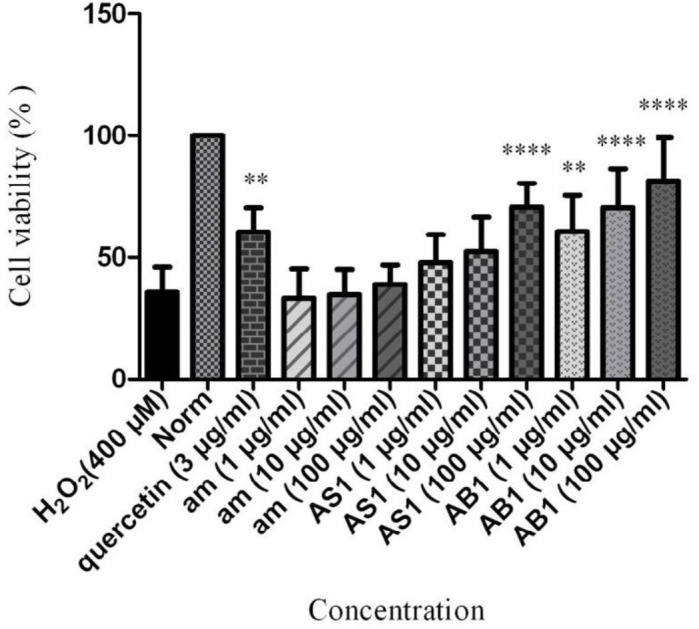
The IC50 values of different extracts of P. armeniaca toward AChE and BChE
Samples | AChEI [IC50 (μg)/mL] | BChEI [IC50 (μg)/mL] |
---|---|---|
AS1 | 356.75 ± 0.171 | > 500 |
HS1 | 418.79 ± 0.365 | > 500 |
SO | 481.42 ± 0.447 | > 500 |
ES | 445.65 ± 8.42 | > 500 |
ES1 | > 500 | > 500 |
AB1 | 134.93± 2.88 | > 500 |
HB1 | > 500 | > 500 |
BO | > 500 | > 500 |
EB | > 500 | > 500 |
EB1 | > 500 | > 500 |
Amygdalin | > 500 | > 500 |
Rivastigmine | 2.77 ± 0.09 | 1.93 ± 0.03 |
Total phenols and flavonoids content from aqueous extracts of P. armeniaca
Sample | Total phenols | Flavonoids | Flavonoids/ |
---|---|---|---|
AS1 | 21.33±0.06 | 16.07 ± 0.03 | 0.75 |
AB1 | 18.61±0.51 | 7.32 ± 0.17 | 0.39 |
Discussion
AD is one of the most common types of dementia. In this respect, various synthetic and plant-derived ChEIs are usually used for the management and amelioration of the disease (15). In this study, P. armeniaca kernels were candidate to be examined for their anti-AD activities via ChEI and neuroprotective properties. The selection of kernels comes back to the efficacy of apricot kernels recommended for the prevention and treatment of memory loss in Iranian traditional medicine (ITM). For this purpose, a wide range of plants have been introduced in ITM which have been thoroughly investigated in the recent studies (27). Also, the presence of glycosides in the kernels may found to be suitable as they are one of the major classes of those compounds documented to possess ChEI activity (35, 36).
It seems that the plants belonging to Rosaceae family containing CNGs have depicted anti-ChE activity (37, 38). According to the literature available, the concentration of constituents and biological properties of apricot kernels not only are affected by the kinds of apricot kernels (sweet or bitter) and solvent extraction, but also are affected by the variety, geographic region, and collection time (34, 39, 40). Hence, anti-ChE and neuroprotective activities of aqueous, hydroalcoholic, petroleum ether, and ethanolic extracts of both sweet and bitter kernels of apricot cultivated in north-western Iran were investigated. In addition, similar studies were conducted for amygdalin as the main constituent of kernels whether it is responsible for the desired activities.
Our results revealed that aqueous extract of bitter apricot kernels showed potent in-vitro acetylcholinesterase inhibitory and neuroprotective effects. However, regarding to results reported in Table 1, amygdalin demonstrated no biological effects. Therefore, the observed effects probably are associated to the synergistic effects of several compounds. It is worth mentioning that AChEI activity of some plants from family Rosaceae including Agrimonia eupatoria, Amygdalus scoparia, Coussapoa microcarpa, Cotoneaster nummularia, Rosa canina, and Rosa damascene depicted low inhibitory activity and P. armeniaca was found much more potent than those reported in the literature (41).
Another instructive point relates to the study reported by Cheng et al., which revealed that amygdalin has improved nerve growth factor (NGF)-induced neuritogenesis and reduced 6-hydroxydopamine (6-OHDA)-induced neurotoxicity in rat dopaminergic PC12 cells (24). On the other side, prunasin known as CNGs which is ubiquitous in the apricot kernels has shown neuroprotective activity in NG108-15 hybridoma cells (42). The neuroprotectivity of bitter kernels may be related to the presence of flavonoids since these compounds have been found as effective neuroprotective agents (43, 44). For example, it has been revealed that the consumption of green tea led to the reduction of risk of Parkinson’s disease (43, 45).
In a study reported by Tohda et al., a kampo formula composed of P. armeniaca L., which is used for the improvement of learning impairment and synaptic loss in the patients with AD, was studied for its neuroprotective abilities in mice (46). It was found that synergistic effects played an important role in directly activating neurons.
There are various reports confirming that cyclooxygenase2 (COX-2) overexpression causes alteration of neuronal cell cycle in a murine model of AD and COX-2 inhibitors inhibited the cell-cycle in the early phase of βAP toxicity (47). Also, in a study reported by Yang et al., amygdalin which was extracted from the seeds of Prunus armeniaca L. suppressed COX-2 and inducible nitric oxide synthase (iNOS) expressions in mouse BV2 microglial cells. It can be considered as an auxiliary role for the cognitive enhancement (48). However, it seems that further investigations are required to determine the exact mechanism of action of apricot kernels as an anti-AD agent.
Conclusion
In conclusion, in-vitro ChE inhibitory and neuroprotective activities of various extractions of bitter and sweet apricot kernels (P. armeniaca L.) were investigated. Our results demonstrated that the best anti-AChE activity and neuroprotectivity against H2O2-induced cell death in PC12 neurons were obtained by the aqueous extract of bitter type. To gain insight into more effective constituent responsible for the desired biological activities, amygdalin as the most abundant compound in the apricot kernels was similarly evaluated. As amygdalin showed neither ChE inhibitory nor neuroprotective activities the synergistic effects of multiple compounds are probably involved in inducing desired biological activities. Also, it is suggested that inhibitory activity of kernels extracts should be evaluated against different factors involved in the creation of AD such as βA aggregation, phosphorylation of tau proteins, and beta secretase (BACE1) to find the possible mechanism of action of apricot kernels for the treatment of dementia and improvement of memory to develop plant-mediated neurodegenerative drug discovery.
Acknowledgements
References
-
1.
Alzheimer’s A. 2015 Alzheimer’s disease facts and figures. Alzheimers Dement. 2015;11:332-84. [PubMed ID: 25984581].
-
2.
Amaducci LA, Rocca WA, Schoenberg BS. Origin of the distinction between Alzheimer’s disease and senile dementia How history can clarify nosology. Neurology. 1986;36:1497-9. [PubMed ID: 3531918].
-
3.
Prince M, Comas-Herrera A, Knapp M, Guerchet M, Karagiannidou M. World Alzheimer report 2016: improving healthcare for people living with dementia: coverage, quality and costs now and in the future. London, UK: Alzheimer’s Disease International; 2016.
-
4.
Muñoz-Torrero D. Acetylcholinesterase inhibitors as disease-modifying therapies for Alzheimer’s disease. Curr. Med. Chem. 2008;15:2433-55. [PubMed ID: 18855672].
-
5.
LaFerla FM, Green KN, Oddo S. Intracellular amyloid-β in Alzheimer’s disease. Nat. Rev. Neurosci. 2007;8:499-509. [PubMed ID: 17551515].
-
6.
Ballatore C, Lee VM-Y, Trojanowski JQ. Tau-mediated neurodegeneration in Alzheimer’s disease and related disorders. Nat. Rev. Neurosci. 2007;8:663-72. [PubMed ID: 17684513].
-
7.
Cheignon C, Tomas M, Bonnefont-Rousselot D, Faller P, Hureau C, Collin F. Oxidative stress and the amyloid beta peptide in Alzheimer’s disease. Redox. Biol. 2018;14:450-64. [PubMed ID: 29080524].
-
8.
Darvesh S, Hopkins DA, Geula C. Neurobiology of butyrylcholinesterase. Nat. Rev. Neurosci. 2003;4:131-8. [PubMed ID: 12563284].
-
9.
Mueller C, Perera G, Hayes RD, Shetty H, Stewart R. Associations of acetylcholinesterase inhibitor treatment with reduced mortality in Alzheimer’s disease: a retrospective survival analysis. Age Ageing. 2017;47:88-94.
-
10.
Greig NH, Utsuki T, Ingram DK, Wang Y, Pepeu G, Scali C, Yu Q-S, Mamczarz J, Holloway HW, Giordano T. Selective butyrylcholinesterase inhibition elevates brain acetylcholine, augments learning and lowers Alzheimer β-amyloid peptide in rodent. Proc. Natl. Acad. Sci. U.S.A. 2005;102:17213-8. [PubMed ID: 16275899].
-
11.
Small DH, Moir RD, Fuller SJ, Michaelson S, Bush AI, Li QX, Milward E, Hilbich C, Weidemann A. A protease activity associated with acetylcholinesterase releases the membrane-bound form of the amyloid protein precursor of Alzheimer’s disease. Biochemistry. 1991;30:10795-9. [PubMed ID: 1718425].
-
12.
Guillozet A, Mesulam MM, Smiley J, Mash D. Butyrylcholinesterase in the life cycle of amyloid plaques. Ann. Neurol. 1997;42:909-18. [PubMed ID: 9403484].
-
13.
Li Y, Hai S, Zhou Y, Dong BR. Cholinesterase inhibitors for rarer dementias associated with neurological conditions. Syst. Rev. 2015:Cd009444.
-
14.
Šešok S, Bolle N, Kobal J, Bucik V, B Vodušek D. Cognitive function in early clinical phase huntington disease after rivastigmine treatment. Psychiatr. Danub. 2014;26:239-48. [PubMed ID: 25191771].
-
15.
Wszelaki N, Kuciun A, Kiss A. Screening of traditional European herbal medicines for acetylcholinesterase and butyrylcholinesterase inhibitory activity. Acta Pharm. 2010;60:119-28. [PubMed ID: 20228046].
-
16.
Adams M, Gmünder F, Hamburger M. Plants traditionally used in age related brain disorders—A survey of ethnobotanical literature. J. Ethnopharmacol. 2007;113:363-81. [PubMed ID: 17720341].
-
17.
Akram M, Nawaz A. Effects of medicinal plants on Alzheimer’s disease and memory deficits. Neural Regen. Res. 2017;12:660-70. [PubMed ID: 28553349].
-
18.
Su Y, Wang Q, Wang C, Chan K, Sun Y, Kuang H. The treatment of Alzheimer’s disease using Chinese medicinal plants: from disease models to potential clinical applications. J. Ethnopharmacol. 2014;152:403-23. [PubMed ID: 24412377].
-
19.
Kurt BZ, Gazioğlu I, Sevgi E, Sönmez F. Anticholinesterase, Antioxidant, Antiaflatoxigenic Activities of Ten Edible Wild Plants from Ordu Area, Turkey. Iran J. Pharm. Res. 2018;17:1047-56. [PubMed ID: 30127827].
-
20.
Raj V, Jain A, Chaudhary J. Prunus armeniaca (Apricot): an overview. J. Pharm. Res. 2012;5:3964-6.
-
21.
Rai I, Bachheti R, Saini C, Joshi A, Satyan R. A review on phytochemical, biological screening and importance of Wild Apricot (Prunus armeniaca ). Orient. Pharm. Exp. Med. 2016;16:1-15.
-
22.
Karsavuran N, Charehsaz M, Celik H, Asma BM, Yakıncı C, Aydın A. Amygdalin in bitter and sweet seeds of apricots. Environ. Toxicol. Chem. 2014;96:1564-70.
-
23.
Katayama S, Ogawa H, Nakamura S. Apricot carotenoids possess potent anti-amyloidogenic activity in-vitro. J. Agric. Food Chem. 2011;59:12691-6. [PubMed ID: 22043804].
-
24.
Cheng Y, Yang C, Zhao J, Tse HF, Rong J. Proteomic identification of calcium-binding chaperone calreticulin as a potential mediator for the neuroprotective and neuritogenic activities of fruit-derived glycoside amygdalin. J. Nutr. Biochem. 2015;26:146-54. [PubMed ID: 25465157].
-
25.
Yang C, Zhao J, Cheng Y, Li X, Rong J. Bioactivity-guided fractionation identifies amygdalin as a potent neurotrophic agent from herbal medicine Semen Persicae extract. Biomed. Res. Int. 2014;2014:306857. [PubMed ID: 25050339].
-
26.
Jorjani SI. Mohaghegh, M, editor. Yadegar on Medicine and Pharmacology. 44. Tehran: Iran: Institute of Islamic Studies in Tehran; 2003. 31 p.
-
27.
Saeedi M, Babaie K, Karimpour-Razkenari E, Vazirian M, Akbarzadeh T, Khanavi M, Hajimahmoodi M, Shams Ardekani MR. In-vitro cholinesterase inhibitory activity of some plants used in Iranian traditional medicine. Nat. Prod. Res. 2017;31:2690-4. [PubMed ID: 28278615].
-
28.
Saeedi M, Vahedi-Mazdabadi Y, Rastegari A, Soleimani M, Eftekhari M, Akbarzadeh T, Khanavi M. Evaluation of Asarum europaeum Rhizome for the Biological Activities Related to Alzheimer’s Disease. Res. J. Pharmacogn. 2020;7:25-33.
-
29.
Saeedi M, Safavi M, Karimpour-Razkenari E, Mahdavi M, Edraki N, Moghadam FH, Khanavi M, Akbarzadeh T. Synthesis of novel chromenones linked to 1, 2, 3-triazole ring system: Investigation of biological activities against Alzheimer’s disease. Bioorg. Chem. 2017;70:86-93. [PubMed ID: 27914694].
-
30.
Gerlier D, Thomasset N. Use of MTT colorimetric assay to measure cell activation. J. Immunol. Methods. 1986;94:57-63. [PubMed ID: 3782817].
-
31.
Singleton VL, Orthofer R, Lamuela-Raventós RM. Analysis of total phenols and other oxidation substrates and antioxidants by means of folin-ciocalteu reagent. Meth. Enzymol. 1999;299:152-78.
-
32.
Marinova D, Ribarova F, Atanassova M. Total phenolics and total flavonoids in Bulgarian fruits and vegetables. J. Univ. Chem. Technol. Metallurgy. 2005;40:255-60.
-
33.
Savic IM, Nikolic VD, Savic-Gajic IM, Nikolic LB, Ibric SR, Gajic DG. Optimization of technological procedure for amygdalin isolation from plum seeds (Pruni domesticae semen). Front. Plant Sci. 2015;6:276. [PubMed ID: 25972881].
-
34.
Lv W-F, Ding M-Y, Zheng R. Isolation and quantitation of amygdalin in Apricot-kernel and Prunus Tomentosa Thunb by HPLC with solid-phase extraction. J. Chromatogr. Sci. 2005;43:383-7. [PubMed ID: 16176653].
-
35.
Suchaichit N, Kanokmedhakul S, Kanokmedhakul K, Moosophon P, Boonyarat C, Plekratoke K, Tearavarich R, Suchaichit NP. Phytochemical investigation and acetylcholinesterase inhibitory activity of bark of Hymenodictyon orixense. Nat. Prod. Res. 2018;32:0.2936. [PubMed ID: 29047314].
-
36.
Nugroho A, Choi JS, Hong J-P, Park H-J. Anti-acetylcholinesterase activity of the aglycones of phenolic glycosides isolated from Leonurus japonicus. Asian Pac. J. Trop. Biomed. 2017;7:849-54.
-
37.
Mukherjee PK, Kumar V, Mal M, Houghton PJ. Acetylcholinesterase inhibitors from plants. Phytomedicine. 2007;14:289-300. [PubMed ID: 17346955].
-
38.
Murray AP, Faraoni MB, Castro MJ, Alza NP, Cavallaro V. Natural AChE inhibitors from plants and their contribution to Alzheimer’s disease therapy. Curr. Neuropharmacol. 2013;11:388-413. [PubMed ID: 24381530].
-
39.
Yıldırım FA, Yıldırım AN, Şan B, Aşkın MA, Polat M. Variability of phenolics and mineral composition in kernels of several bitter and sweet apricot (Prunus armeniaca Batsch. ) cultivars.J. Food Agri. Environ. 2010;8:179-84.
-
40.
Stoewsand G, Anderson J, Lamb R. Cyanide content of apricot kernels. J. Food Sci. 1975;40:1107.
-
41.
Esmaeili S, Ara L, Hajimehdipoor H, Kolivand H, Mohammadi Motamed S. Acetylcholinesterase inhibitory effects of some plants from Rosaceae. Res. J. Pharmacogn. 2015;2:33-7.
-
42.
Tan HP, Wong DZH, Ling SK, Chuah CH, Kadir HA. Neuroprotective activity of galloylated cyanogenic glucosides and hydrolysable tannins isolated from leaves of Phyllagathis rotundifolia. Fitoterapia. 2012;83:223-9. [PubMed ID: 22093753].
-
43.
Vauzour D, Vafeiadou K, Rodriguez-Mateos A, Rendeiro C, Spencer JP. The neuroprotective potential of flavonoids: a multiplicity of effects. Genes Nutr. 2008;3:115-26. [PubMed ID: 18937002].
-
44.
Dajas F, Rivera F, Blasina F, Arredondo F, Echeverry C, Lafon L, Morquio A, Heizen H. Cell culture protection and in-vivo neuroprotective capacity of flavonoids. Neurotox. Res. 2003;5:425-32. [PubMed ID: 14715446].
-
45.
Checkoway H, Powers K, Smith-Weller T, Franklin GM, Longstreth Jr WT, Swanson PD. Parkinson’s disease risks associated with cigarette smoking, alcohol consumption, and caffeine intake. Am. J. Epidemiol. 2002;155:732-8. [PubMed ID: 11943691].
-
46.
Tohda C, Tamura T, Komatsu K. Repair of amyloid β (25–35)-induced memory impairment and synaptic loss by a Kampo formula, Zokumei-to. Brain Res. 2003;990:141-7. [PubMed ID: 14568338].
-
47.
Xiang Z, Ho L, Valdellon J, Borchelt D, Kelley K, Spielman L, Aisen PS, Pasinetti GM. Cyclooxygenase (COX)-2 and cell cycle activity in a transgenic mouse model of Alzheimer’s disease neuropathology. Neurobiol. Aging. 2002;23:327-34. [PubMed ID: 11959394].
-
48.
Yang H-Y, Chang H-K, Lee J-W, Kim Y-S, Kim H, Lee M-H, Shin M-S, Ham D-H, Park H-K, Lee H. Amygdalin suppresses lipopolysaccharide-induced expressions of cyclooxygenase-2 and inducible nitric oxide synthase in mouse BV2 microglial cells. Neurol. Res. 2007;29:59-64.