Abstract
Keywords
Licochalcone B Liver cancer Signaling pathways DNA repair system Apoptosis
Introduction
Cancer is an important public health concern worldwide that has brought about an increasing number of deaths, and a huge number of patients are analyzed every year (1). Hepatocellular carcinoma (HCC) is one of the world’s most common cancers and causes almost one million deaths yearly (2). HCC is increasingly present all over the world because of the pandemic of obesity (3). The common treatment for HCC incorporates chemotherapy, radiation, and surgical resection; however, these treatments provide little promise for the restoration of well-being on account of poor results and severe side effects. Liver transplantation is thought to be the best option for patients with HCC. However, the low availability of organs limits this option for patients with HCC, and the high risk of tumor recurrence after transplantation further compromises its viability (4). Therefore, the development of more powerful and fewer side effects anti-tumor drugs, including non-synthetic agents, are needed to prevent or slow the process of hepatocarcinogenesis. Apurinic/apyrimidinic endonuclease 1 (APE1), the mammalian ortholog of Escherichia coli XthA (exonuclease III), is an essential protein that plays a crucial role in maintaining genome integrity. APE1 exhibits another vital capacity as redox factor-1 (Ref-1), which reductively activates transcription, factors including c-Jun, activator protein- 1, nuclear factor kappa B (NF-κB), the tumor- suppressor protein p53, hypoxia-inducible element 1α (HIF-1α), and paired box gene 8, which are involved in different cellular processes such as cell survival, cell signaling, and inflammatory (5). Past research has demonstrated the role of APE1/Ref-1 in the pathogenesis of numerous disorders, including liver fibrosis, liver cirrhosis, neuroinflammation, and different cancers (6-9). The proliferation of eukaryotic cells involves a methodical progression through four distinct phases of the cell cycle: G1, S, G2, and M (10). Cyclin-dependent kinases (CDKs) assume a key role in controlling cell-cycle progression and, to a large degree, oversee cell advancement from the developmental stages G1 and G2 into the stages associated with DNA replication (S) and mitosis (M) (11). Tumor initiation, promotion, and progression normally involve changes in programmed cell death. Several studies have demonstrated that dysregulation of programmed cell death is a critical causal variable in the development of HCC (12). Likewise, a growing body of evidence demonstrates that apoptosis induction is important in the chemoprevention of malignancy by dietary components (13). Flavonoids are among the most powerful and multifunctional substances in plants and have been shown to exhibit anti-cancer, anti-inflammatory, and hepatoprotective effects (14). Licochalcone B (LCB), which belongs to the retrochalcone family, is purified from Chinese licorice roots. Although the essential properties of LCB are still being investigated, studies have shown that LCB possesses powerful antioxidant and free radical scavenging properties as well as many other beneficial pharmacological activities, such as anti-inflammatory and cardioprotective effects (15, 16). Nanoparticle drug delivery systems have many interesting properties, including longer dissemination half-lives, enhanced pharmacokinetics, and decreased toxicity, especially at the systemic level (17). When combined with chemotherapeutic agents, nanoparticles can exploit the hyperpermeability and impaired blood flow of tumor vasculatures, thereby enhancing drug accumulation at the tumor site (18). Lately, researchers have investigated various ways to deliver multiple therapeutic agents using a single-medication nanocarrier because chemoresistance, the main cause of failed tumor treatment, may be prevented by using different medications. Tumor cells resist chemotherapy by overexpressing drug efflux pumps, expanding drug inactivation systems, increasing self-repairing capacity, or expressing altered drug targets (19). For some time, combination chemotherapy has been embraced in clinics as an essential cancer treatment regimen to reduce tumor drug resistance and improve treatment outcomes. Fullerene C60 (FnC60) is a nanoparticle that comprises 32 faces, including 12 pentagons and 20 hexagons. The carbon atoms are organized at 60 vertices of a truncated icosahedrons (20). The properties of FnC60 have been investigated extensively over the past decade, and the nanoparticle has been shown to have advantageous activity as a neuroprotectant as well as protective effects against cancer, inflammation, atherogenesis, and radiation (21). The present study aimed to evaluate the synergistic efficacy of using LCB in combination with FnC60 to prevent diethylnitrosamine (DEN)-induced HCC by assessing DNA fragmentation, oxidative DNA damage (8-hydroxy-2′-deoxyguanosine levels), mRNAs expression of different proapoptotic and anti-apoptotic markers, and mRNAs and protein expression of APE1/Ref-1 and CDK-4. The protective mechanism was further studied for related signaling pathways.
Experimental
Chemicals
LCB (purity ≥ 98%) was purchased from Shanghai Li Chen Biotechnology Co., Ltd. (Shanghai, China). DEN and FnC60 were obtained from Sigma Chemical Co. (St. Louis, Missouri, USA). All other reagents were of analytical, high-performance liquid chromatography, or pharmaceutical grade.
Preparation of FnC60
FnC60 with a purity of more than 99.5% (MER Corporation, Tucson, Arizona, USA) was used. A total of 50 mg of FnC60Fn was dissolved in 10 mL of corn oil by mixing for two weeks at ambient temperatures, and the resulting blend was centrifuged at 5000 ×g for 30 min. The supernatant was sifted through a Millipore filter with a pore size of 0.25 mm (22).
Animals and medicines
Fifty male Wistar rats weighing 120–130 g were used in this study. The rats were treated following the Guide for the Care and Use of Laboratory Animals (National Institutes of Health. The rats were housed separately, and a standard laboratory diet and water were given ad libitum. The animal room was maintained at a constant temperature of 25 ºC with a relative humidity of 50% and a light/cycle of 12 h. After one week of the standard diet, animals were randomly assigned to five groups (n = 10). The rats in the DEN + LCB, DEN + FnC60, and DEN + LCB + FnC60 groups were pretreated with LCB and FnC60 (25 and 4 mg· (kg body weight)-1, either alone or in combination, by gastric tube for two weeks, whereas the animals in control and DEN groups received equivalent volumes of corn oil. Next, all the animals except for those in the control group received DEN (10 mg· (kg body weight)-1 orally for 20 consecutive weeks and the rats in the DEN + LCB, DEN + FnC60, and DEN + LCB + FnC60 groups continued to receive LCB or FnC60 either alone or in combination until the end of the experiment. The dose of LCB was based on the results of a previous study by Teng etal. (23), while the dose of FnC60 was based on the results of Baati et al. (22) and the dosage of DEN was based on Zhang et al. study (24). The animals’ were weighed weekly, and the doses were adjusted as needed. Following 140 days of DEN treatment, the rats of all groups were anesthetized 24 h after the last treatment, and liver tissue was extracted and weighed. A portion of the liver was fixed in paraformaldehyde (10%) for histopathological examination, and the remainder was quickly frozen in liquid nitrogen prior to storage at -80 ºC.
Determination of 8-OHdG in the liver
DNA was separated from liver tissue using specialized kits. The levels of 8-OHdG were examined by ELISA. Briefly, an 8-OHdG antibody and sample DNA were added to an ELISA plate that had been pre-coated with 8-OHdG. The 8-OHdG in the sample competes with the 8-OHdG bound to the plate for the 8-OHdG antibody. The mean contents of 8-OHdG per microgram of DNA for each group were calculated for each sample. The percent DNA fragmentation in the liver tissue was measured using the colorimetrical diphenylamine assay.
RNA purification and reverse transcription
Total RNA was extracted from hepatic tissue using TRIzol reagent (Invitrogen Corp., USA) as per the manufacturer’s guidelines. The RNA was then dissolved in water containing diethyl pyrocarbonate. The concentration and purity of the RNA were determined using a Nanodrop spectrophotometer (Nanodrop 2000c, Thermo Scientific, USA). Complementary DNA was synthesized using a RevertAid First Strand cDNA Synthesis Kit as per the manufacturer’s protocol.
Real-time PCR
Gene expression levels in hepatic tissue were determined using real-time PCR. The primers were formulated by Sangon Biotech Co., Ltd. (Shanghai, China) (Table 1). All reactions were carried out using Maxima SYBR Green qPCR Master Mix in a MasterCyclerTM real plex 4 (Eppendorf, Westbury, New York, USA) with the following cycling conditions: initial denaturation at 92 ºC for 12 min, followed by 35 cycles of 10 s at 92 ºC, 30 s at 58 ºC, and 20 s at 70 ºC. The differences in gene expression among groups were calculated using the 2-ΔΔCT method, normalized to GAPDH and expressed as relative mRNA levels compared with the controls (25).
Western blotting
APE1/Ref-1 and CDK-4 protein expression were examined using western blot analysis. Protein extracts from liver tissue were prepared using a lysis buffer (50 mmol·L- 1 Tris-HCl, pH 7.6, 0.5% Triton X-100, and 20% glycerol). The extracts were then subjected to centrifugation (15000 ×g, 15 min at 4 ºC). The supernatant fractions were assayed for protein concentration using Bradford reagent (Bio-Rad, Richmond, California, USA) and were then used for western blot analyses of APE1/Ref-1, CDK-4, and β-actin (Cell Signaling Technology, Beverly, Massachusetts A, USA). Horseradish peroxidase-conjugated IgG (Zymed, South San Francisco, California A, USA) was used as a secondary antibody.
Liver histology
For histopathology, paraffin-embedded sections approximately 5 µm thick were prepared, deparaffinized, rehydrated, stained with hematoxylin and eosin, and examined using an Olympus AX70 microscope (Olympus, Tokyo, Japan).
Statistical analysis
SPSS 13.0 was used for the statistical analysis. A chi-square test was performed to analyze the nodule incidence. Data were expressed as the mean ± SD and analyzed using a one-way ANOVA followed by Tukey’s test for multiple comparisons. Differences were considered significant at P < 0.05.
Results
Changes in body and liver weights
The effects of LCB and FnC60 on the bodyweight changes induced by DEN are summarized in Figure 1. After the first week of DEN administration, the rats began to exhibit slowed growth. In addition, with DEN treatment, the average weight gain gradually decreased. Following 98 days of DEN administration, the body weight in the DEN group began to decrease. After 140 days, the weights of the rats in the control group had increased to approximately three times their initial values, whereas the weights of the rats in the DEN group were only about 1.61- times their initial values. However, the LCB and FnC60 treatments significantly restored the body weight gain of the rats, and the DEN + LBCB + FnC60 group showed body weights closer to those of the control group. Figure 2 shows the liver weights of the different groups of animals. The liver weight in the DEN group was greater than that in the control group (P < 0.05). The administration of LBCB and FnC60 reduced the liver weight: in groups treated with LCB, FnC60, or their combination, the liver weight was lower than that of the DEN group (P < 0.05) and comparable to that of the control group.
LCB and FnC60 inhibited DEN-induced formation of 8-OHdG and DNA fragmentation in the liver
As illustrated in Figure 3, after 140 days of DEN treatment, the 8-OHdG content increased by 2.31-fold (P < 0.01) in relation to the control group’s content. However, treatment with LCB and FnC60 attenuated the increase in 8-OHdG induced by DEN. The 8-OHdG content was significantly (P < 0.05) decreased by about 33.8%, 36.3%, and 53.7% in the DEN + LBCB, DEN + FnC60, and DEN + LBCB + FnC60 groups, respectively, compared with the DEN group, and the level in the DEN + LCB + FnC60 group was not significantly different from that in the control group. Also, the percent DNA fragmentation was significantly increased in the DEN-treated group compared to the control group, while it was significantly decreased in the DEN + LCB, DEN + FnC60, and DEN + LBCB + FnC60 groups compared with the DEN group and were not significantly different between the DEN + LCB + FnC60 group and the control animals (Figure 4).
LCB and FnC60 augmented cell death in the livers of rats with DEN-induced HCC
Because dysregulation of cell death is a crucial factor in the origination of hepatocarcinoma, we further quantified the gene expression of mediators of apoptosis (12). As illustrated in Figure 5, the real-time PCR data revealed upregulation of gene expression of the anti-apoptotic proteins B-cell lymphoma 2 (Bcl-2), B-cell lymphoma xL (Bcl-xL), and β-arrestin 2 in the DEN group compared with the control group (P < 0.05). However, significant decreases in the mRNA expression levels of Bcl-2-associated X protein (Bax) and caspase-3 (CASP-3) were observed in the DEN rats compared with the control animals. Therefore, the Bcl-2/Bax ratio was significantly increased in the DEN rats compared with the control group, Treatment with LCB and FnC60 obviously decreased the expression of Bcl-2 and Bcl-xL and augmented the expression of Bax and CASP-3 compared with levels in the DEN group. The Bcl-2/Bax ratios were significantly decreased in the LCB and FnC60 groups compared with the DEN group.
LCB and FnC60 downregulated APE1/Ref-1 and upregulated p53 mRNA expression in the livers of DEN-treated rats
As illustrated in Figure 6, the quantitative real-time PCR results revealed a significant increase in the mRNA expression levels of APE1/Ref-1 and a significant decrease in the mRNA expression level of p53 in the DEN group compared with the control group (P < 0.05). However, the administration of LCB and FnC60 markedly decreased the expression of APE1/Ref-1 mRNA and increased the expression of p53 mRNA compared with expression levels in the DEN group. The APE1/Ref-1 mRNA expression level in the DEN + LCB + FnC60 group was not significantly different from that of the control groups.
LCB and FnC60 downregulated CDK-4 and retinoblastoma (Rb) mRNA expression in the livers of DEN-treated rats
As shown in Figure 6, the PCR results showed a significant increase in the mRNA expression levels of CDK-4 and Rb in the DEN group compared with the control group (P < 0.05). However, the administration of LCB and FnC60 markedly decreased the expression of CDK-4 and Rb mRNA compared with levels in the DEN group, and levels in the DEN + LCB + FnC60 group were not significantly different from those in the control group.
LCB and FnC60 downregulated APE1/Ref-1 and CDK-4 protein expression in the livers of DEN-treated rats
As shown in Figure 7, the western blot results revealed a significant increase in the protein expression levels of APE1/Ref-1 and CDK-4 in the DEN group compared with the control group (P < 0.05). However, the administration of LCB and FnC60 markedly decreased the expression of APE1/Ref-1 and CDK-4 compared with levels in the DEN group and the control group.
Histological changes in the liver
Representative histological images of the liver for all groups are shown in Figure 8. The hepatic tissue of the control group exhibited normal hepatocyte architectures (Figure 8a). In the rats treated with DEN, the liver sections showed areas of aberrant hepatocellular phenotypes that included nuclear size variations, hyperchromatism, and irregular sinusoids (Figure 8b). Also, abnormal hepatocellular histology with prominent hyperbasophilic preneoplastic focal lesions and the presence of eosinophilic and clear cell foci was also observed (Figure 8c). Finally, examining the livers of rats treated with DEN showed the trabeculae of HCC, including highly pleomorphic tumor cells and degenerated tumor cells. These cells were characterized by eosinophilic cytoplasm, large or binucleated nuclei, and prominent nucleoli. Intercellular bile canaliculi were also present (Figure 8d). These histopathological appearances were obviously lessened in the livers of rats treated with LCB and FnC60, which appeared similar to those of the control animals. Furthermore, histological analysis of the livers of rats treated with LCB and FnC60 revealed significant improvement in the hepatocellular architecture compared with that of the DEN group (Figures 8e-8g).
Discussion
Lately, obvious considerations have been interested in using the antioxidants as possible targets for mitigating diverse maladies due to their specific natural actions and low toxicity (26-36).
In the present study, the combination of LCB and FnC60 reduced histopathological damage, inhibited oxidative DNA damage, and decreased DNA fragmentation induced by DEN. Furthermore, we found that LCB and FnC60 upregulated proapoptotic signals and downregulated anti-apoptotic signals. Interestingly, the tested compounds down-regulated the gene and protein expression of enzymes involved in DNA repair and cell-cycle progression. These results provide strong evidence that LCB and FnC60 act synergistically to prevent the development of DEN-induced HCC. Oxidative DNA damage might be a critical event in the development of malignancy (37). DEN, a potent hepatocarcinogen in rats, may exert its effects by altering DNA structure, particularly by generating alkyl DNA adducts and producing chromosomal abnormalities and micronuclei in the liver (38). To further evaluate the role of LCB and FnC60 in the inhibition of DEN-induced HCC, we determined the contents of 8-OHdG, a DNA lesion induced by reactive oxygen species (ROS) and an important indicator of oxidative DNA injury caused by advanced age, tumors, and chronic diseases (39). The results clearly indicated that treatment with LCB and FnC60 significantly reduced hepatic 8-OHdG content and percent DNA fragmentation in relation to the levels observed in DEN-treated rats, which suggests that LCB and FnC60 prevented DEN-induced DNA damage. Tumor initiation, promotion, and progression usually involve changes in programmed cell death. Studies have shown that dysregulation of cell death is imperative for HCC advancement and that induction of apoptosis is important in the chemoprevention of malignancy by dietary components (12, 13). Thus, we evaluated changes in the mRNA expression level of mediators of apoptosis. The Bcl-2 protein family regulates proapoptotic factors and has a vital role in determining the capacity of cells to experience cell death (40). Bax is normally found in the cytoplasm but translocates to the mitochondria to promote cell death; however, the activity of Bax is neutralized by anti-apoptotic factors such as Bcl-2 (41). Numerous tumor cells avoid apoptosis by upregulating Bcl-2 and Bcl-xL expression (42). In the current work, LCB and FnC60 upregulated Bax expression and downregulated Bcl-2 and Bcl-xL expression, in contrast with the changes observed in rats treated only with DEN. The ratio of Bcl-2 to Bax, rather than the levels of the individual proteins, is thought to be a basic determinant of cell survival or demise (43). A decrease in the Bcl2/Bax ratio will initiate cell death (44). In the current study, the ratio of Bcl-2 to Bax was substantially reduced by treatment with LCB and FnC60, which may have triggered apoptosis amid the hepatocarcinogenesis induced by DEN. A number of studies have demonstrated that an important mechanism by which capsaicin, zerumbone, matrine, and leptin prevent HCC involves reducing Bcl-2/Bax ratios (45). CASP-3 activation is crucial for the execution of apoptosis and is a specific event in both the internal and external apoptotic pathways (46). In the current experiment, DEN exposure reduced CASP-3 expression, while LCB and FnC60 increased CASP-3 expression. Cell death induced by PTX-2 in human HCC is associated with downregulation of Bcl-2 and Bcl-xL, upregulation of Bax, and activation of CASP-3 (47). Thus, LCB and FnC60 may have triggered apoptosis through downregulation of Bcl-2 and Bcl-xL and upregulation of Bax and CASP-3, and these components might be essential for the prevention of HCC. The protein β-arrestin-2 was initially recognized as a terminator of G protein-coupled receptor signaling and has been shown to have novel capacities as a signal transducer in different pathways (48). With these activities, β-arrestin 2 may prevent apoptotic signaling by activating the anti-apoptotic pathway (49). In the current study, β –arrestin 2 transcription was increased in DEN-treated rodents and this may have had an anti-apoptotic effect related to upregulation of Bcl-2 and Bcl-xL and downregulation of Bax and CASP-3. Conversely, the LCB and FnC60 treatments reduced β-arrestin 2 mRNA expression levels, thereby promoting apoptotic signaling through upregulation of Bax and CASP-3. Moreover, β -arrestin-2 has a role in the Wnt signaling pathway, which is frequently imbalanced in HCC (50). Increased ROS generation is a mechanism by which certain exogenous chemicals instigate apoptosis; therefore, antioxidants can suppress apoptosis (51). In addition, certain chemicals prevent tumors by increasing antioxidant activity and causing cell death. For instance, polyphenols, lycopene, and moringa oleifera have noteworthy chemo-prophylactic effects, counteracting disease through their antioxidant activities and induction of cell death (51). Therefore, it is plausible that LCB and FnC60 have powerful antioxidant capacities as well as proapoptotic effects. Pretreatment with LCB and FnC60 reduced ROS generation, which was reflected in decreased 8-OHdG levels, without hindering the proapoptotic activities of LCB and FnC60. This fact indicates that the mechanisms of HCC prevention by LCB and FnC60 has multiple components. The control of CDK action happens at numerous levels, including the addition and removal of phosphate from CDK, cyclin formation and destruction, the transcription, degradation, and accessibility of protein inhibitors, and the subcellular localization of these different components (10). Progression through the G1-/S transition requires the addition of phosphate to Rb by CDK-4 or the highly homologous kinase CDK-6 complexed with its activating subunits, the D type cyclins (D1, D2, and D3) (52, 53). Major phosphorylation of Rb lessens its capacity to quell gene expression through the E2F family of transcription factors and therefore permits the synthesis of many genes, the protein products of which are important for DNA division (54). Accordingly, the activity of CDK-4 or CDK-6 manages a basic checkpoint for the G1-/S transition and cell division (55). More than 90% of human cancers have biochemical and genetic adaptations that allow them to circumvent the mechanisms that control this checkpoint (56). The irregularities include upregulation of CDK-4 enhancement of D-type cyclins; downregulation of p16INK4A , an inhibitor of CDK-4; changes in CDK-4 that inhibit the action of p16INK4A on the enzyme; and degradation or transformation of Rb (57). These distortions can trigger the loss of proliferative controls either by removing the checkpoint completely or by promoting inappropriate or increased CDK-4 activity, which brings about Rb hyperphosphorylation. The recurrence of these changes suggests that annulment of the G1 checkpoint and speeding up of the CDK-4/cyclin D pathway creates favorable circumstances for the multiplication and survival of cancer cells. In view of this, cyclin D–dependent kinases have long been considered prime targets for chemotherapy (58). Preliminary evidence suggests that inhibition of cyclin D–dependent kinase activity may prevent cancer development or at least reverse the altered phenotype. For instance, the reduction of cyclin D expression through missense mutation causes an accompanying decrease in cyclin D–dependent kinase activity and subsequently inhibits cancer development, nullifies tumorigenicity, or causes tumor cell death in specific cases (59). Other studies have demonstrated that upregulation of p16INK4A expression in tumor cells can inhibit proliferation and reduce tumorigenicity (60). These observations bolster the case for using CDK-4/6 as a target for tumor treatment and suggest that a specific inhibitor of these enzymes would produce a meaningful therapeutic response. Our results showed that DEN induces HCC through upregulation of both CDK-4 and Rb, and the administration of LCB and FnC60 downregulates CDK-4 and Rb. These novel discoveries provide new information on the anti-cancer potential of the tested compounds.
APE1/Ref-1 has been associated with several different human ailments, and its dysregulation, post-translational modification, or sequence polymorphisms can influence susceptibility toward these maladies, as observed for several types of cancer (9). In this study, DEN initiated treatment upregulated APE1/Ref-1 expression. Overexpression and increased enzymatic activities of APE1/Ref-1 have been associated with survival and chemoresistance in cancer cells (61). Phytochemicals may alter the repair and redox activities of APE1/Ref-1 (62). Resveratrol (trans-3′,4′,5-trihydroxystilbene), an essential polyphenolic anti-cancer agent present in grapes and other food sources, has shown antiproliferative activity against cancer cells in-vitro (63). Resveratrol also has a vital role in counteracting stress by regulating APE/Ref-1 (8). The antioxidant effect of LCB (23) and FnC60 (64) may be the mechanism by which these compounds reduce the expression of APE-1/Ref-1, which is principally activated by oxidative stress (8). APE1/Ref-1 also activates HIF-1α and p53 using its redox activity. Activation of the tumor-suppressor protein p53 by APE1/Ref-1 adds to the oxidative stress response. Activated p53 translocates to the nucleus to promote the transcription of p53-responsive genes, such as p21, cyclin G, and Bax genes (65). To elucidate the mechanisms underlying the protective effect of LCB and FnC60, we measured the protein expression of APE-1/Ref-1 and CDK-4. Western blot analysis showed that DEN treatment enhanced APE-1/Ref-1 and CDK-4 protein expression. However, LCB either alone or in combination with FnC60 decreased APE1/Ref-1 and CDK-4 protein expression, indicating that regulation occurs at both transcriptional and translational levels.
Changes at the molecular level were confirmed by histopathology. The histopathological images of livers of rats in the DEN group demonstrated a distorted hepatocellular phenotype that included nuclear size variations, hyperchromatism, and sporadic sinusoids with conspicuous hyperbasophilic preneoplastic central lesions and eosinophilic clear cell foci. Additionally, profoundly pleomorphic and degenerated tumor cells were observed. This finding was consistent with the results obtained by Sreepriya and Bali, who reported that the presence of atypical nuclei is a marker of HCC (66). Nevertheless, animals receiving LCB alone or in combination with FnC60 showed entryway regions and hepatic lobules comparable to those of the control animals, which demonstrated liver recovery. These results appear to show that FnC60 had a synergistic effect increasing the penetration and consequently the bioavailability of LCB. Biological membranes play critical roles in the homeostasis of all organisms because they segregate important activities between and within cells and tissues. Small hydrophobic molecules can partition across biological membranes down a concentration gradient, but hydrophilic molecules generally require some sort of selective transport system to cross the lipid bilayer. Previous studies have shown that certain compounds can facilitate the transport of polar molecules across biological membranes (67).
Nucleotide sequences of the primers used in RT-PCR
Gene symbol | GenBank | Primer (5'→3') |
---|---|---|
Bcl-2 | NM_016993.1 | F: CCCCAGAAGAAACTGAACC |
Bcl-XL | XM_017591479.1 | F: CGTGGAAAGCGTAGACAAGG |
β-arrestin-2 | NM_012911.1 | F: CCACGTCACCAACAATTCTG |
Bax | NM_017059.2 | F: GTTGCCCTCTTCTACTTTGC |
Caspase-3 | NM_012922.2 | F: CTGGACTGCGGTATTGAGAC |
APE-1/Ref-1 | NM_024148.1 | F: TGCTGTGTGGGGATCTCAAT |
P53 | NM_030989.3 | F: CTCCTCTCCCCAGCAAAAGA |
Cdk-4 | NM_053593.2 | F: TGATGCGCCAGTTTCTAAGC |
Rb | NM_017045.1 | F: TCACATTCCTCGAAGCCCTT |
GAPDH* | NM_017008.4 | F: TCAAGAAGGTGGTGAAGCAG |
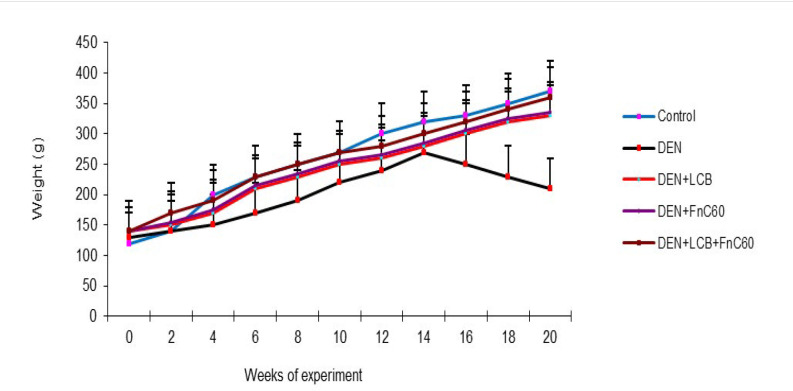
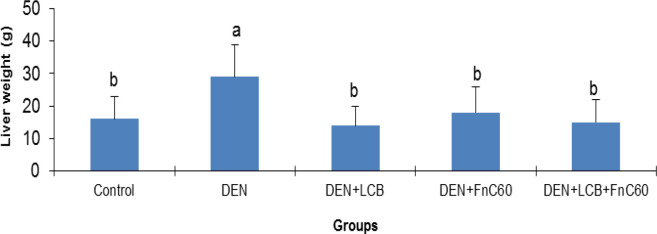
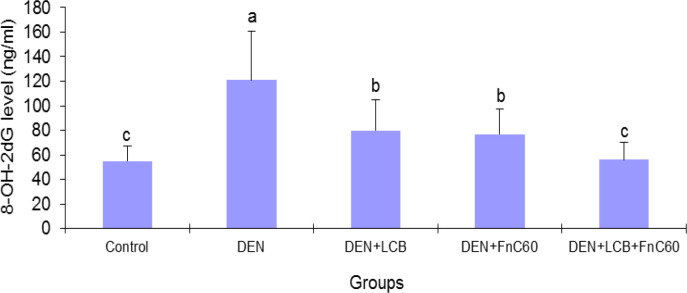
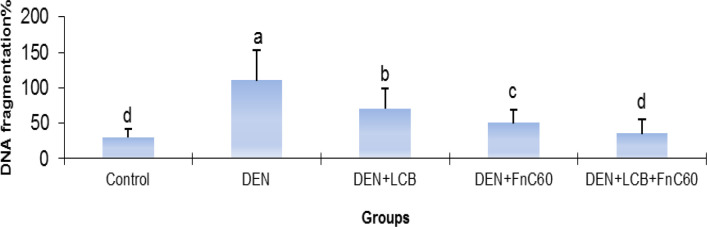
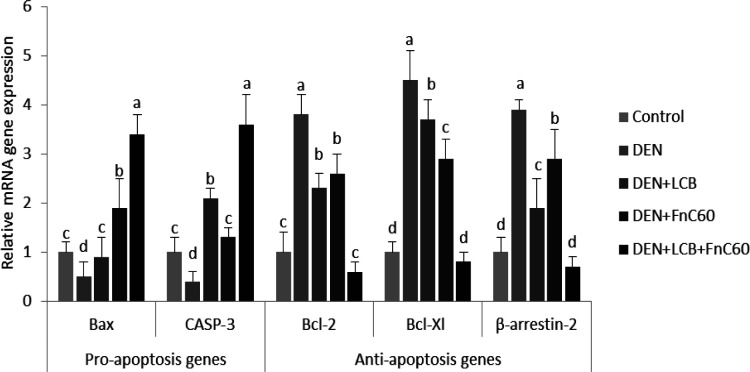
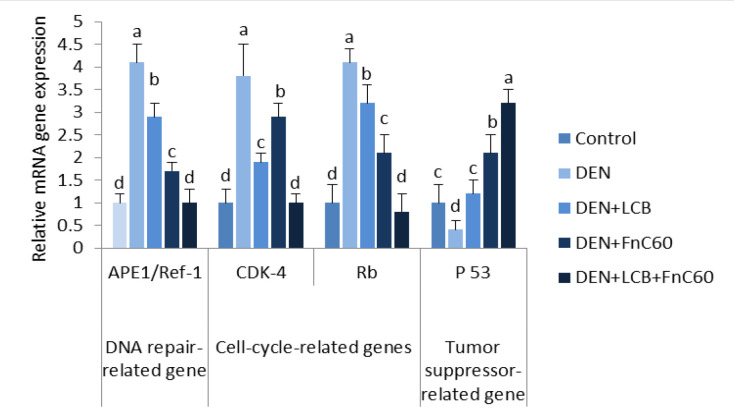
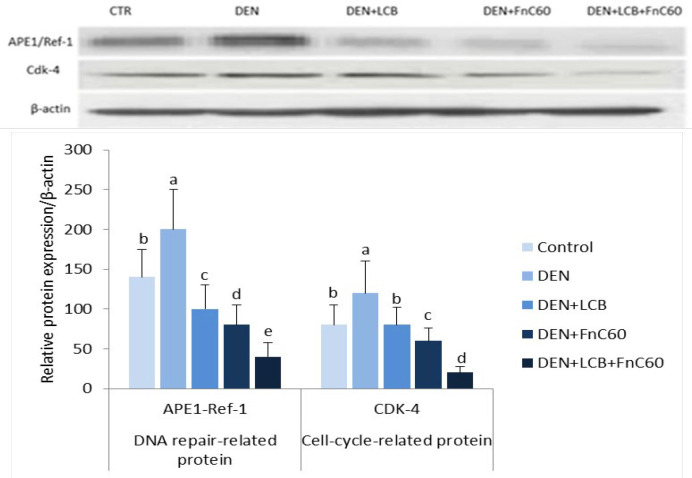
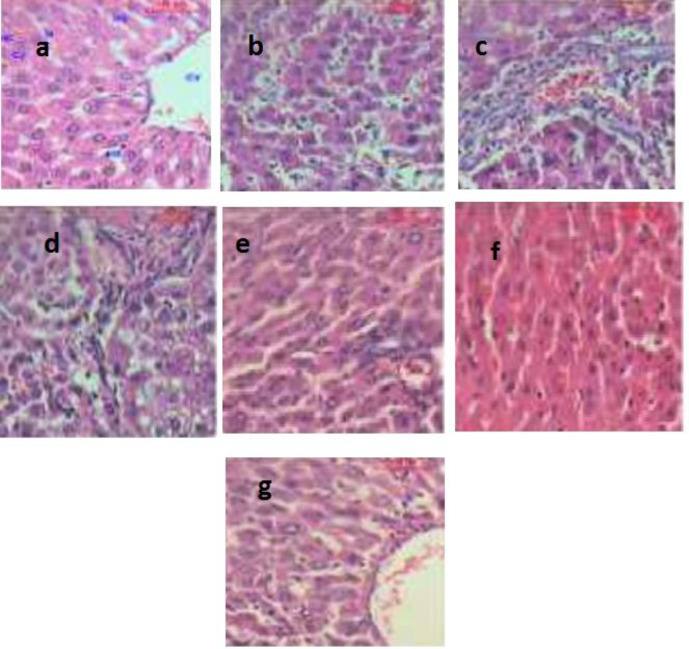
Conclusion
To our knowledge, the findings presented herein are the first to demonstrate the in-vivo synergistic effects of LCB and FnC60 against DEN-induced HCC. The chemo-prophylactic effect of LCB and FnC60 may be accompanied by regulation of the DNA repair system and the cell- cycle at S-phase progression and the induction of apoptosis at the gene and protein expression levels. Effective treatments for HCC are scarce; however, our findings suggest the potential utility of LCB and FnC60 in chemoprevention of HCC with APE1/Ref-1 and CDK-4 as therapeutic targets.
Acknowledgements
References
-
1.
Jemal A, Bray F, Center MM, Ferlay J, Ward E, Forman D. Global cancer statistics. CA Cancer J. Clin. 2011;61:69-90. [PubMed ID: 21296855].
-
2.
Inoue H, Seitz H. Viruses and alcohol in the pathogenesis of primary hepatic carcinoma. Eur. J. Cancer Prev. 2001;10:107-10. [PubMed ID: 11263585].
-
3.
Scalera A, Tarantino G. Could metabolic syndrome lead to hepatocarcinoma via non-alcoholic fatty liver disease? World J. Gastroenterol. 2014;20:9217-28.
-
4.
Tabone M, Pellicano R. Prevention of intrahepatic hepatocarcinoma recurrence in patients with viral cirrhosis: two potential options. Minerva Gastroenterologica e Dietologica. 2006;52:47-52. [PubMed ID: 16554706].
-
5.
Bapat A, Fishel ML, Kelley MR. Going ape as an approach to cancer therapeutics. Antioxid. Redox Signal. 2009;11:651-67. [PubMed ID: 18715143].
-
6.
Bassiouny AR, Zaky A, Kandeel KM. Alteration of AP-endonuclease1 expression in curcumin-treated fibrotic rats. Ann. Hepatol. 2011a;10:516-30. [PubMed ID: 21911894].
-
7.
Bassiouny AR, Zaky AZ, Abdulmalek SA, Kandeel KM, Ismail A, Moftah M. Modulation of AP-endonuclease1 levels associated with hepatic cirrhosis in rat model treated with human umbilical cord blood mononuclear stem cells. Int. J. Clin. Exp. Pathol. 2011b;4:692-707. [PubMed ID: 22076170].
-
8.
Zaky A, Mohammad B, Moftah M, Kandeel KM, Bassiouny AR. Apurinic/apyrimidinic endonuclease 1 is a key modulator of aluminum-induced neuroinflammation. BMC Neurosci. 2013;14:26. [PubMed ID: 23497276].
-
9.
Chen S, Xiong G, Wu S, Mo J. Downregulation of apurinic/apyrimidinic endonuclease 1/redox factor-1 enhances the sensitivity of human pancreatic cancer cells to radiotherapy in-vitro. Cancer Biother. Radio. 2013;28:169-76.
-
10.
Obaya A, Sedivy J. Regulation of cyclin-Cdk activity in mammalian cells. Cell. Mol. Life Sci. 2002;59:126-42. [PubMed ID: 11846025].
-
11.
Chauhan S, Diril MK, Lee JH, Bisteau X, Manoharan V, Adhikari D, Ratnacaram CK, Janela B, Noffke J, Ginhoux F, Coppola V, Liu K, Tessarollo L, Kaldis P. Cdk2 catalytic activity is essential for meiotic cell division in-vivo. Biochem. J. 2016;473:2783-98. [PubMed ID: 27371320].
-
12.
Fabregat I. Dysregulation of apoptosis in hepatocellular carcinoma cells. World J. Gastroenterol. 2009;15:513-20. [PubMed ID: 19195051].
-
13.
Khan N, Adhami VM, Mukhtar H. Apoptosis by dietary agents for prevention and treatment of prostate cancer. Endocr.-Relat. Cancer. 2010;17:R39-R52. [PubMed ID: 19926708].
-
14.
Rezende TP, Corrêa JOdA, Aarestrup BJ, Aarestrup FM, de Sousa OV, da Silva Filho AA. Protective effects of Baccharisdracunculifolia leaves extract against carbon tetrachloride-and acetaminophen-induced hepatotoxicity in experimental animals. Molecules. 2014;19:9257-72. [PubMed ID: 24991758].
-
15.
Fu Y, Chen J, Li YJ, Zheng YF, Li P. Antioxidant and anti-inflammatory activities of six flavonoids separated from licorice. Food Chem. 2013;141:1063-71. [PubMed ID: 23790887].
-
16.
Han J, Wang D, Yu B, Wang Y, Ren H, Zhang B, Wang Y, Zheng Q. Cardioprotection against ischemia/reperfusion by licochalcone B in isolated rat hearts. Oxidative Med. Cell. Longev. 2014;2014:134862.
-
17.
Davis ME, Shin DM. Nanoparticle therapeutics: an emerging treatment modality for cancer. Nat. Rev. Drug Discov. 2008;7:771-82. [PubMed ID: 18758474].
-
18.
Danhier F, Feron O, Preat V. To exploit the tumor microenvironment: Passive and active tumor targeting of nanocarriers for anti-cancer drug delivery. J. Control. Release. 2010;148:135-46. [PubMed ID: 20797419].
-
19.
Gottesman MM. Mechanisms of cancer drug resistance. Ann. Rev. Med. 2002;53:615-27. [PubMed ID: 11818492].
-
20.
Heymann AD, Shapiro Y, Chodick G, Shalev V, Kokia E, Kramer E, Shemer J. Reduced hospitalizations and death associated with influenza vaccination among patients with and without diabetes. Diabetes Care. 2004;27:2581-4. [PubMed ID: 15504989].
-
21.
Tykhomyrov AA, Nedzvetsky VS, Klochkov VK, Andrievsky GV. Nanostructures of hydrated C 60 fullerene (C 60 HyFn) protect rat brain against alcohol impact and attenuate behavioral impairments of alcoholized animals. Toxicology. 2008;246:158-65. [PubMed ID: 18289766].
-
22.
Baati T, Bourasset F, Gharbi N, Njim L, Abderrabba M, Kerkeni A, Szwarc H, Moussa F. The prolongation of the lifespan of rats by repeated oral administration of [60] fullerene. Biomaterials. 2012;33:4936-46. [PubMed ID: 22498298].
-
23.
Teng H, Chen M, Zou A, Jiang H, Han J, Sun L, Feng C, Liu J. Hepatoprotective effects of licochalcone B on carbon tetrachloride-induced liver toxicity in mice. Iran. J. Basic Med. Sci. 2016;19:910-5. [PubMed ID: 27746874].
-
24.
Zhang CL, Zeng T, Zhao XL, Yu LH, Zhu ZP, Xie KQ. Protective effects of garlic oil on hepatocarcinoma induced by N-nitrosodiethylamine in rats. Int. J. Biol. Sci. 2012;8:363-74. [PubMed ID: 22393308].
-
25.
Livak KJ, Schmittgen TD. Analysis of relative gene expression data using real-time quantitative PCR and the (2− ΔΔCT method. Methods. 2001;25:402-8. [PubMed ID: 11846609].
-
26.
Ghanema IIA, Sadek KM. Olive leaves extract restored the antioxidant perturbations in red blood cells hemolysate in streptozotocin induced diabetic rats. World Acad. Sci. Eng. Technol. Int. J. Biol. Biomol. Agric. Food Biotechnol. Eng. 2012;6:124-30.
-
27.
Mohamed Sadek K. Antioxidant and immunostimulant effect of carica papaya linn Aqueous extract in acrylamide intoxicated rats. Acta Inform. Med. 2012;20:180-5. [PubMed ID: 23322975].
-
28.
Sadek KM. Barley phenolic compounds impedes oxidative stress in lead acetate intoxicated rabbits. Oxid. Antioxid. Med. Sci. 2012;1:141-6.
-
29.
Sadek KM. Chemotherapeutic efficacy of an ethanolic Moringa oleifera leaf extract against chromium-induced testicular toxicity in rats. Andrologia. 2014;46:1047-54. [PubMed ID: 24215114].
-
30.
Sadek KM, Abouzed TK, Abouelkhair R, Nasr S. The chemo-prophylactic efficacy of an ethanol Moringa oleifera leaf extract against hepatocellular carcinoma in rats. Pharm. Biol. 2017;55:1458-66. [PubMed ID: 28345375].
-
31.
Sadek KM, Lebda MA, Abouzed TK, Nasr SM, Shoukry M. Neuro- and nephrotoxicity of subchronic cadmium chloride exposure and the potential chemoprotective effects of selenium nanoparticles. Metab. Brain Dis. 2017;32:1659-73. [PubMed ID: 28660360].
-
32.
Sadek KM, Lebda MA, Nasr SM, Shoukry M. Spirulina platensis prevents hyperglycemia in rats by modulating gluconeogenesis and apoptosis via modification of oxidative stress and MAPK-pathways. Biomed. Pharmacother. 2017;92:1085-94. [PubMed ID: 28622709].
-
33.
Sadek KM, Shaheen H. Biochemical efficacy of vitamin D in ameliorating endocrine and metabolic disorders in diabetic rats. Pharm. Biol. 2014;52:591-6. [PubMed ID: 24251869].
-
34.
Taha N, Korshom M, Mandour A, Sadek K. Effects of garlic and acrylamide on some antioxidant enzymes. Global J. Med. Plant Res. 2013;1:190-4.
-
35.
Taha N, Korshom M, Mandour A, Sadek K. Lipoic acid impedes markers of oxidative stress in the liver of streptozotocin-diabetic rats. Global J. Med. Plant Res. 2013;1:195-8.
-
36.
Taha N, Sadek K, Korshom M, Mandour A. The green tea improve the oxidative status in stereptozotocin-induced diabetic rats. Int. J. Curr. Res. 2013;1:79-82.
-
37.
Mandal P. Potential biomarkers associated with oxidative stress for risk assessment of colorectal cancer. Naunyn-Schmiedebergs Arch. Pharmacol. 2017;390:557-65. [PubMed ID: 28229171].
-
38.
Al-Rejaie SS, Aleisa AM, Al-Yahya AA, Bakheet SA, Alsheikh A, Fatani AG, Al-Shabanah OA, Sayed-Ahmed MM. Progression of diethylnitrosamine-induced hepatic carcinogenesis in carnitine-depleted rats. World J. Gastroenterol. 2009;15:1373-80. [PubMed ID: 19294768].
-
39.
Tsubota A, Yoshikawa T, Nariai K, Mitsunaga M, Yumoto Y, Fukushima K, Hoshina S, Fujise K. Bovine lactoferrin potently inhibits liver mitochondrial 8-OHdG levels and retrieves hepatic OGG1 activities in Long-Evans Cinnamon rats. J. Hepatol. 2008;48:486-93. [PubMed ID: 18191270].
-
40.
Tse C, Shoemaker AR, Adickes J, Anderson MG, Chen J, Jin S, Johnson EF, Marsh KC, Mitten MJ, Nimmer P. ABT-263: a potent and orally bioavailable Bcl-2 family inhibitor. Cancer Res. 2008;68:3421-8. [PubMed ID: 18451170].
-
41.
Edlich F, Banerjee S, Suzuki M, Cleland MM, Arnoult D, Wang C, Neutzner A, Tjandra N, Youle RJ. Bcl-x L retrotranslocates Bax from the mitochondria into the cytosol. Cell. 2011;145:104-16. [PubMed ID: 21458670].
-
42.
Danial NN, Korsmeyer SJ. Cell death: critical control points. Cell. 2004;116:205-19. [PubMed ID: 14744432].
-
43.
Andreu-Fernandez V, Sancho M, Genoves A, Lucendo E, Todt F, Lauterwasser J, Funk K, Jahreis G, Perez-Paya E, Mingarro I, Edlich F, Orzaez M. Bax transmembrane domain interacts with prosurvival Bcl-2 proteins in biological membranes. Proc. Natl. Acad. Sci. USA. 2017;114:310-5. [PubMed ID: 28028215].
-
44.
Gardner CR. Anti-cancer drug development based on modulation of the Bcl-2 family core apoptosis mechanism. ExpertRev. AnticancerTher. 2004;4:1157-77.
-
45.
Ma L, Wen S, Zhan Y, He Y, Liu X, Jiang J. Anticancer effects of the Chinese medicine matrine on murine hepatocellular carcinoma cells. Planta Medica. 2008;74:245-51. [PubMed ID: 18283616].
-
46.
Sarada S, Himadri P, Ruma D, Sharma S, Pauline T. Selenium protects the hypoxia induced apoptosis in neuroblastoma cells through upregulation of Bcl-2. Brain Res. 2008;1209:29-39. [PubMed ID: 18405886].
-
47.
Qi F, Li A, Inagaki Y, Xu H, Wang D, Cui X, Zhang L, Kokudo N, Du G, Tang W. Induction of apoptosis by cinobufacini preparation through mitochondria-and Fas-mediated caspase-dependent pathways in human hepatocellular carcinoma cells. Food Chem. Toxicol. 2012;50:295-302. [PubMed ID: 22019693].
-
48.
DeWire SM, Ahn S, Lefkowitz RJ, Shenoy SK. β-arrestins and cell signaling. Annu. Rev. Physiol. 2007;69:483-510. [PubMed ID: 17305471].
-
49.
Khan MS, Devaraj H, Devaraj N. Chrysin abrogates early hepatocarcinogenesis and induces apoptosis in N-nitrosodiethylamine-induced preneoplastic nodules in rats. Toxicol. Appl. Pharmacol. 2011;251:85-94. [PubMed ID: 21167192].
-
50.
Bryja V, Gradl D, Schambony A, Arenas E, Schulte G. β-arrestin is a necessary component of Wnt/β-catenin signaling in-vitro and in-vivo. Proc. Natl. Acad. Sci. 2007;104:6690-5. [PubMed ID: 17426148].
-
51.
Sadek KM, Saleh EA, Nasr SM. Molecular hepatoprotective effects of lipoic acid against carbon tetrachloride-induced liver fibrosis in rats: Hepatoprotection at molecular level. Hum. Exp. Toxicol. 2018;37:142-54. [PubMed ID: 29233029].
-
52.
Gubern A, Joaquin M, Marques M, Maseres P, Garcia-Garcia J, Amat R, Gonzalez-Nunez D, Oliva B, Real FX, de Nadal E, Posas F. The N-Terminal phosphorylation of RB by p38 bypasses its inactivation by CDKs and prevents proliferation in cancer cells. Mol. Cell. 2016;64:25-36. [PubMed ID: 27642049].
-
53.
Chen Q, Lin J, Jinno S, Okayama H. Overexpression of Cdk6–cyclin D3 highly sensitizes cells to physical and chemical transformation. Oncogene. 2003;22:992-1001. [PubMed ID: 12592386].
-
54.
Trimarchi JM, Lees JA. Sibling rivalry in the E2F family. Nat. Rev. Mol. 2002;3:11-20.
-
55.
Ho A, Dowdy SF. Regulation of G 1 cell-cycle progression by oncogenes and tumor suppressor genes. Curr. Opin.Genet.Dev. 2002;12:47-52. [PubMed ID: 11790554].
-
56.
Malumbres M, Barbacid M. Milestones in cell division: to cycle or not to cycle: a critical decision in cancer. Nat. Rev. Cancer. 2001;1:222-31. [PubMed ID: 11902577].
-
57.
Whittaker SR, Mallinger A, Workman P, Clarke PA. Inhibitors of cyclin-dependent kinases as cancer therapeutics. Pharmacol. Ther. 2017;57:3939-65.
-
58.
Fry D, Garrett M. Inhibitors of cyclin-dependent kinases as therapeutic agents for the treatment of cancer. Curr. Opin. Oncol. Endoc. Metab. Invest. 2000;2:33-40.
-
59.
Nomanbhoy TK, Sharma G, Brown H, Wu J, Aban A, Vogeti S, Alemayehu S, Sykes M, Rosenblum JS, Kozarich JW. Chemoproteomic evaluation of target engagement by the cyclin-dependent kinase 4 and 6 inhibitor palbociclib correlates with cancer cell response. Biochemistry. 2016;55:5434-41. [PubMed ID: 27571378].
-
60.
Matsumura K, Nakata S, Taniguchi K, Ii H, Ashihara E, Kageyama S, Kawauchi A, Yoshiki T. Depletion of gamma-glutamylcyclotransferase inhibits breast cancer cell growth via cellular senescence induction mediated by CDK inhibitor upregulation. BMC Cancer. 2016;16:1-9.
-
61.
Sengupta S, Mantha AK, Mitra S, Bhakat KK. Human AP endonuclease (APE1/Ref-1) and its acetylation regulate YB-1-p300 recruitment and RNA polymerase II loading in the drug-induced activation of multidrug resistance gene MDR1. Oncogene. 2011;30:482-93. [PubMed ID: 20856196].
-
62.
Raffoul JJ, Banerjee S, Singh-Gupta V, Knoll ZE, Fite A, Zhang H, Abrams J, Sarkar FH, Hillman GG. Down-regulation of apurinic/apyrimidinic endonuclease 1/redox factor-1 expression by soy isoflavones enhances prostate cancer radiotherapy in-vitro and in-vivo. Cancer Res. 2007;67:2141-9. [PubMed ID: 17332344].
-
63.
Wong DH, Villanueva JA, Cress AB, Sokalska A, Ortega I, Duleba AJ. Resveratrol inhibits the mevalonate pathway and potentiates the antiproliferative effects of simvastatin in rat theca-interstitial cells. Fertil. Steril. 2011;96:1252-8. [PubMed ID: 21907337].
-
64.
Andrievsky GV, Bruskov VI, Tykhomyrov AA, Gudkov SV. Peculiarities of the antioxidant and radioprotective effects of hydrated C 60 fullerene nanostuctures in-vitro and in-vivo. Free Radic. Biol. Med. 2009;47:786-93. [PubMed ID: 19539750].
-
65.
Zhang T, He WH, Feng LL, Huang HG. Effect of doxorubicin-induced ovarian toxicity on mouse ovarian granulosa cells. Regul. Toxicol. Pharmacol. 2017;86:1-10. [PubMed ID: 28219713].
-
66.
Sreepriya M, Bali G. Effects of administration of Embelin and Curcumin on lipid peroxidation, hepatic glutathione antioxidant defense and hematopoietic system during N-nitrosodiethylamine/Phenobarbital-induced hepatocarcinogenesis in Wistar rats. Mol. Cell. Biochem. 2006;284:49-55. [PubMed ID: 16477385].
-
67.
Filipski KJ, Varma MV, El-Kattan AF, Ambler CM, Ruggeri RB, Goosen TC, Cameron KO. Intestinal targeting of drugs: rational design approaches and challenges. Curr. Top. Med. Chem. 2013;13:776-802. [PubMed ID: 23578023].