Abstract
Keywords
Atorvastatin Radiosensitizing Apoptosis Ionizing radiation Radiosensitizer
Introduction
Radiotherapy is one of the most effective strategies for cancer treatment. In this treatment protocol, ionizing radiation (IR) is passing through cells and produces free radical and reactive oxygen species (ROS) in cellular environment. These toxic substances could attack to critical macromolecule such as DNA that cause damage. The extensive DNA damage results in cell death (1). However, IR is causing cell death; cancer cells activate endogenous radioresistance signaling pathways in response to killing effect of IR (2, 3). Radioresistance response in tumor cells is the major challenge in cancer treatment. To make overcoming resistance of the cancer cells to IR, there are several approaches as increasing dose of IR or use of radiosensitizers (4). The use of high dose of IR induces side effects on normal tissue that is leading mismanagement of patient during treatment. The administration of a radioprotective agent reduces side effects induced by IR on normal cells and tissues (5, 6). Radiosensitizer is able to sensitize cancer cells to IR (4). Therefore, blocking the cellular repair using radiosensitizer can enhance the efficacy of cancer treatment (7). Atorvastatin (ATV) is a member of statin family that is inhibiting HMG-CoA reductase activity and is a cholesterol-loweing drug. It exhibits anti-cancer activity in several human cancer cells (8-10). ATV is able to induce autophagy in cancer cells leads to cell death (11, 12). However, ATV has exhibited anti-cancer effects, several studies demonstrated the radioprotective effect of ATV on normal tissues. Recently, we demonstrated that ATV reduced side effects of IR on lymphocyte, testis, and kidney (13-15). Anti-inflammatory, enhanced endogenous antioxidant, inhibition of lipid peroxidation, and caspase-3 are main mechanisms involved in radioprotective effect of ATV on normal cells and tissues. However, the protective effects of ATV on normal cells and tissues have been well studied. There are limited studies about the effect of co-treatment of ATV and IR on cancer cells. In a clinical trial, we demonstrated that topical administration of ATV reduced skin toxicity induced by IR in patients with breast cancer (16). Thus, the present study attempts to investigate the sensitizing effect of ATV on IR-induced apoptosis and cell death in breast cancer (MDA-MB-231) and non-small cell lung cancer (A-549) cells. These results demonstrate ATV is potentially radiation sensitizer for the treatment of human breast and lung cancers.
Experimental
MDA-MB-231 and A-549 cell lines were obtained from the Pasteur Institute (Iran) and cultured at 37 °C and 5% CO2 in Dulbecco’s Modified Eagle Medium (DMEM) (Gibco, Paisley, UK) supplemented with 10% fetal bovine serum (FBS) (Gibco, UK) and 100 μg/mL penicillin–streptomycin (Gibco, UK). Annexin-V-FLUOS Staining Kit was obtained from eBioscienc (USA). Atorvastatin (from Sobhan daru Pharmaceutical Company, Iran) was freshly prepared in DMSO as stock solution and diluted in culture medium at concentration dose 10 µM. This ATV concentration was chosen based on our previous study that ATV exhibited in-vitro the highest radioprotective effects on human normal lymphocyte without any cellular toxicity as compared to the other concentrations (13).
Apoptosis determination by flow cytometry
Apoptotic cells were analysed in cancer cells at 24 h after exposure of cells to IR. MDA-MB-231 and A-549 cells (3 × 105) were treated with ATV at concentration 10 μM for four hours in 12-well plates and then were exposed to IR at dose 4 Gy. The cells were irradiated with 4 MV X-ray produced by a radiotherapy machine (Linear accelerator, Siemens, Primus, Germany) at a 1.96 Gy/min dose rate and source to sample distance (SSD) of 60 cm. The cells were cultured in DMEM medium for 24 h. The apoptotic cells were determined using an ‘Annexin-V-FLUOS Staining Kit’ according to the manufacturer’s instruction (eBioscience, USA). Briefly, the cells were washed with PBS and incubated with Annexin-V FLUOS labeling solution (containing 2 μL Annexin-V-FLUOS labelling reagent and 2 μL propidium iodide (PI) solution in 100 μL incubation buffer for each sample) at room temperature in the dark for 15 min. Following incubation, the analysis was performed with a Partec flow cytometer system and Flomax software (Partec, Germany). Unstained cells were considered as negative control for background determination. For each sample, a minimum of 10000 events were counted and analysed.
Antiproliferation assay
MDA-MB-231 and A-549 cells (10,000 cells) were plated in each well of a 96-well plate and were incubated and allowed to attach for 24 h in a humidified atmosphere of 5% CO2 in air at 37 °C (Incubator-Biotek-NB 203L Korea). After incubation, the cells were treated with ATV (10 µM) and incubated for 4 h before exposure to X-ray at dose 4 Gy. The radiation dose was selected according to our preliminary study. The cells were incubated for 72 h in a humidified atmosphere of 5% CO2 in air at 37 °C. The culture medium was removed and MTT solution (5 mg/mL PBS) was then added and the plate was located in optimal atmosphere at 37 °C. The metabolically active cells reduced MTT to blue formazan crystals. After incubating for 4 h, the formazan crystals in each well were dissolved in DMSO. The absorbance of each well was read with an ELISA Reader at 570/630 nm (Bioteck, USA). The cells without any treatment were used as control for comparison of absorbance and cell viability (17).
ROS determination
The intracellular accumulation of ROS was assayed using DCFH-DA, an uncharged, cell permeate fluorescent probe. Inside the cells, DCFH-DA is cleaved by nonspecific esterases forming DCFH, which is the non-fluorescein form and is oxidized to the fluorescent compound DCF in the presence of ROS. MDA-MB-231 and lung cancer cells were seeded in 96 well-plates at a density of 2 × 104 cells per well overnight. The cells were treated with ATV (10 μM) for 4 h. Afterwards the cells were washed with PBS and loaded with 30 µM DCFH-DA in PBS for 30 min. DCFH-DA was removed and each well was loaded with PBS. The plate was irradiated with X-ray at a single dose of 4 Gy. Since the ROS oxidize intracellular DCFH to the fluorescent DCF dye, the DCF fluorescence intensity is taken as being directly proportional to the ROS concentration. The intensity of fluorescence was recorded by a fluorescence spectrophotometer, with an excitation filter of 490 nm and an emission filter of 520 nm. The ROS level was calculated as the mean intensity of the treated cells.
Statistical analysis
The data values are presented as means ± standard deviation (SD). Statistical analysis was performed using one-way analysis of variance (ANOVA), as well as Tukey’s multiple comparison and Dunnett multiple comparison tests. P-value < 0.05 was considered as significant and highly significant (Prism 7 Software, 2016, USA).
Results
Apoptosis in MDA-MB-231 cells treated with ATV and radiation
The flow cytometry was used for measurement of apoptosis in breast cancer cells which were treated with ATV and exposed to IR (Figure 1).
As shown in Figure 2, compared to the control group (8.0% ± 2.1), the apoptosis rate of MDA-MB-231 cells was increased in ATV-alone group (13.9% ± 2) and IR-alone group (15.4% ± 3.1) (P < 0.01). A non-significant difference was observed between ATV and IR groups in induction of apoptosis in MDA-MB-231 cells was observed. Furthermore, the apoptosis rate was significantly increased in MDA-MB-231 + IR group (28.4% ± 3.3) compared to the IR alone and ATV alone groups (P < 0.001).
Apoptosis in A-549 cells treated with ATV and radiation
The flow cytometry was used for measurement of apoptosis in lung cancer cells which were treated with ATV and exposed to IR (Figure 3). As shown in Figure 4, compared to the control group (25.9% ± 1.9), the apoptosis rate of A-549 cells was increased in ATV-alone group (37.6% ± 3.9) and IR-alone group (38.3% ± 4.5) (P < 0.05). A non-significant difference was observed between ATV and IR groups in induction of apoptosis in A-549 cells was observed. Furthermore, the apoptosis rate was significantly increased in A-549 + IR group (54.2% ± 7.2) compared to the IR alone and ATV alone groups (P < 0.01).
Effect of ATV and ionizing radiation on cell proliferation in MDA-MB-231
The effect of ATV on proliferation of breast cancer cell was determined by MTT assay. Breast cancer cells proliferation was significantly inhibited by ATV at 10 µM concentration after 72 h incubation (P < 0.01) (Figure 5). ATV exhibited a survival rate of 71% ± 5 in MDA-MB-231 cells. IR exhibited a survival rate of 78% ± 5 in MDA-MB-231 cells at dose 4 Gy (P < 0.01). The proliferation of breast cancer cells was significantly reduced by ATV in combination with IR. This additive effect was 59% ± 1 in proliferation of breast cancer cells. A significant difference was observed between ATV+ IR and ATV or IR alone (P < 0.05) was observed. This result indicates that ATV has additive effect with X-ray on inhibition of cell growth in breast cancer cell. A radiosensitizing effect of ATV on the breast cancer cells was observed.
Effect of ATV and ionizing radiation on cell proliferation in A-549 cells
The effect of ATV on proliferation of lung cancer cell was determined by MTT assay. Non-small cell lung cancer cells proliferation was significantly inhibited by ATV at 10 µM concentration after 72 h incubation (P < 0.01) (Figure 6). ATV exhibited a survival rate of 85% ± 7 in A-549 cells. IR exhibited a survival rate of 83% ± 4 in A-549 cells at dose 4 Gy (P < 0.01). The proliferation of lung cancer cells was significantly reduced by ATV in combination with IR. This additive effect was 73% ± 4 in proliferation of lung cancer cells. A non-significant difference was observed between ATV+IR and ATV or IR alone (P < 0.05) was observed. This result indicates that ATV has additive effect with X-ray on inhibition of cell growth in the lung cancer cell. A radiosensitizing effect of ATV on the lung cancer cells was observed.
ROS levels
Ionizing radiation produces ROS and oxidative stress result in cellular toxicity. Irradiated MDA-MB-231 and A-549 cells showed a significant increase in DCF fluorescence after 4 Gy irradiation (P < 0.001) (Figure 7). Treatment cells with 10 µM ATV significantly increased ROS levels in irradiated cells (P < 0.05) (Figure 8). Thus, pre-treatment with ATV showed an additive effect on radiation-induced ROS generation in the irradiated breast and lung cancer cells.
Discussion
In this study we demonstrated that ATV sensitized MDA-MB-231 breast and A-549 lung cancer cells to IR. ATV increased apoptosis and cell deaths induced by IR on the breast and lung cancer cells. The resistance of the cancer cells to IR is a challenge in radiotherapy regimen in the patients. The cancer cells activate various signalling pathways resulting in resistance to radiation-induced cell death (3). In cancer treatment, it is very benefit to find a medication which enhances killing effect of IR on cancer cells. The administration of a safe radiosensitizer by the patient during radiotherapy results in the improved cancer treatment, also dose of radiation for cancer treatment could be reduced in the patients (3, 4). It is noticed that a radiosensitizer should be ineffective or has radioprotective effect on the normal cells, otherwise radiosensitizer enhances radiation-induced toxicity on the normal cells. ATV is widely used as a medicine for lowering cholesterol in the patients. Recently we showed ATV protected normal human lymphocytes to IR. ATV treatment reduced genotoxicity induced by IR on lymphocytes that micronuclei assay was performed for evaluation of its radioprotective effect. The maximum radioprotection was observed with in-vitro ATV treatment at dose 10 µM on irradiated normal human lymphocytes with an efficacy of 68% protection. Genotoxicity was not observed with ATV alone at concentration 10 µM on normal human lymphocytes (13). It is interesting that ATV exhibited a radiosensitizing effect on the breast and lung cancer cells at concentration of 10 µM while ATV exhibited radioprotective effect on normal cells at this dose. IR causes free radicals in cellular environment that these toxic substances have crucial role in cell death. The dose 4 Gy of IR was chosen based on previous studies that this dose was able to increase apoptosis in cancer cells (18-20). In this study, we showed that exposure of cancer cells with IR at dose 4 Gy significantly increased ROS production, and an additive ROS production was observed in cancer cell pretreated with ATV. Findings showed that enhanced ROS production is a mechanism involved in cancer cell deaths that were exposed to IR. Previous studies showed that ATV has anticancer effect through different mechanisms. ATV induces apoptosis and cell deaths in breast, prostate, and bladder cancer cells through autophagy (9, 21 and 22) and inducing chromosome breaks (23). ATV effectively induced apoptosis in ovarian cancer cells through activation of Jun N-terminal kinases (JNK) (24). ATV is able to induce oxidative stress in breast cancer cell results in apoptosis and cell deaths (25). Several mechanisms have been demonstrated for radiosensitizing effects of drugs such as AKT/mTOR signalling pathways and cox-2 inhibition (by celecoxib) (26), activated checkpoint kinase 2 (CHK2) ( by valproic acid) (27), ROS production (by mefenamic acid) (19), reduction of intracellular GSH content and HIF-1α expression (by oleanolic acid) (28), downregulated endogenous STAT1 expression (by zoledronic acid) (29), cell cycle G2/M arrest (by albendazole) (30), and autophagy inducing ( by vitamin D or vitamin D analogs) (31). Recently Jones et al. showed that anti-proliferative activity of ATV in the ovarian cancer cell lines was associated with induction of apoptosis, autophagy, cellular stress, and cell cycle G1 arrest via inhibition of AKT/mTOR and activation of the MAPK pathways. ATV inhibited cell adhesion and invasion, and finally ATV exhibited anti-metastasis (32). Metastasis is a challenge of cancer disease in patients, and ATV could diminish metastasis in patients with cancers. In our study, ATV alone exhibited anti-proliferation and apoptosis in breast and lung cancer cells, that is in accordance with previous studies. In this study, an additive and synergistic effect of ATV and IR were observed on the irradiated breast and lung cancer cells. Recently we showed that ATV protected testis and kidneys against toxicity induced by IR in mice. The antioxidant, anti-inflammatory, and anti-caspase-3 activities were main mechanisms of ATV in radioprotection (14, 15).
The dual capabilities of ATV to act as an oxidant and radiosensitizing effect in breast and lung cancer cells, yet radioprotective effect in normal cells, supports the role of ATV as a promising agent for radiotherapy that could significantly improve the cancer treatment.
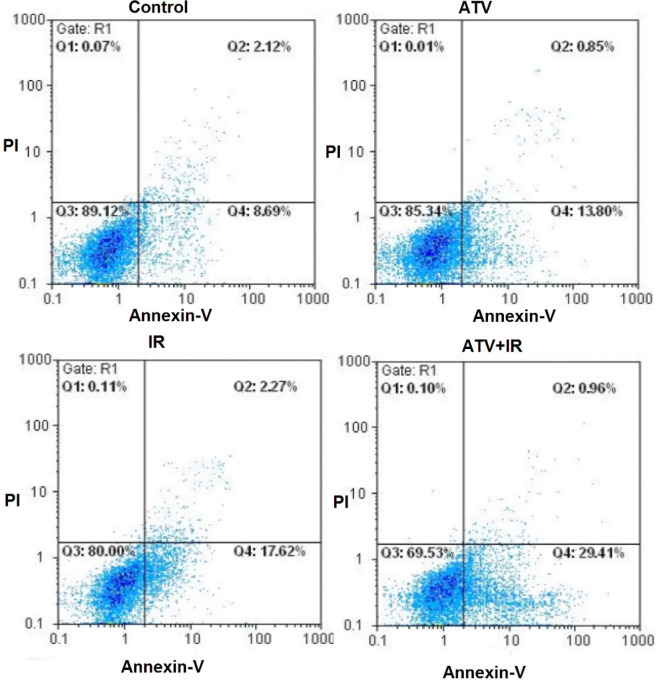
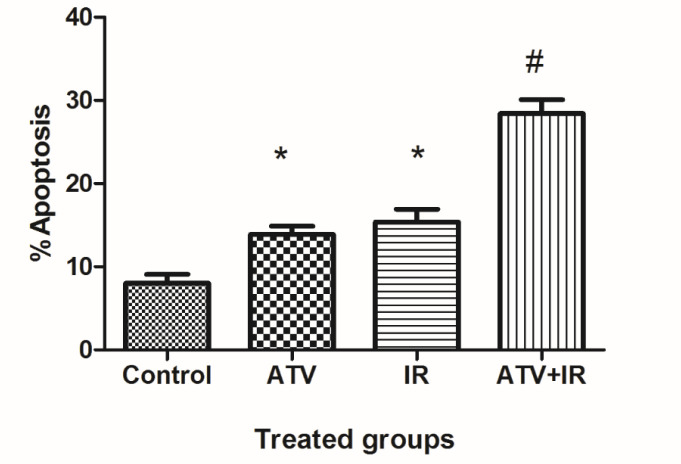
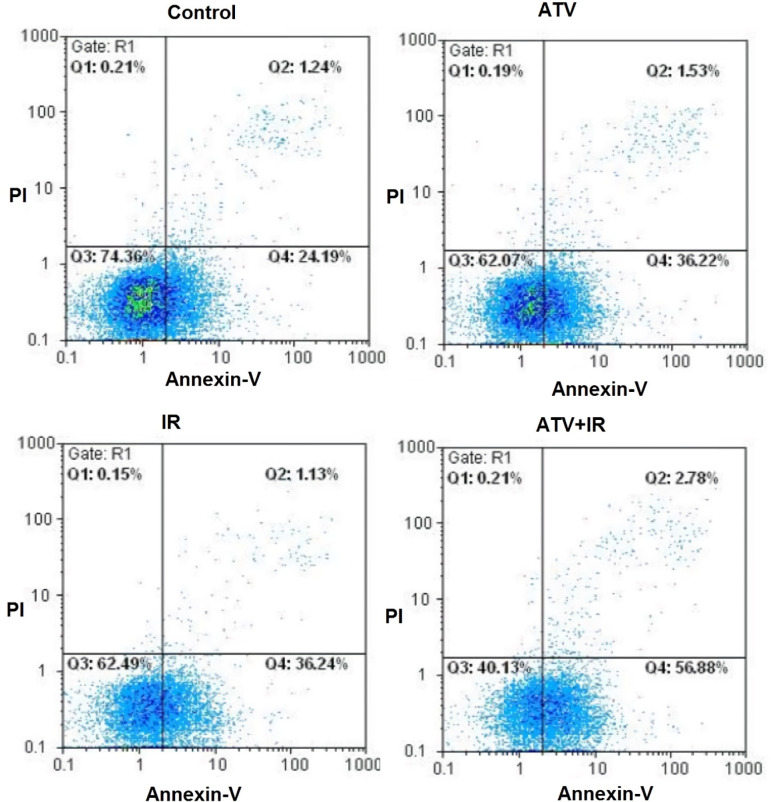
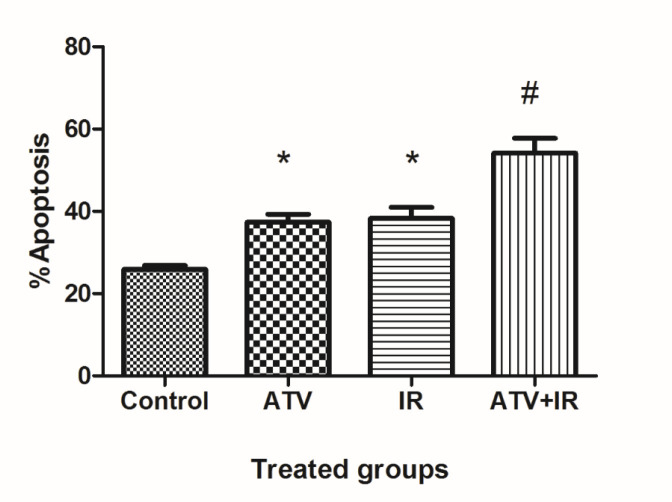
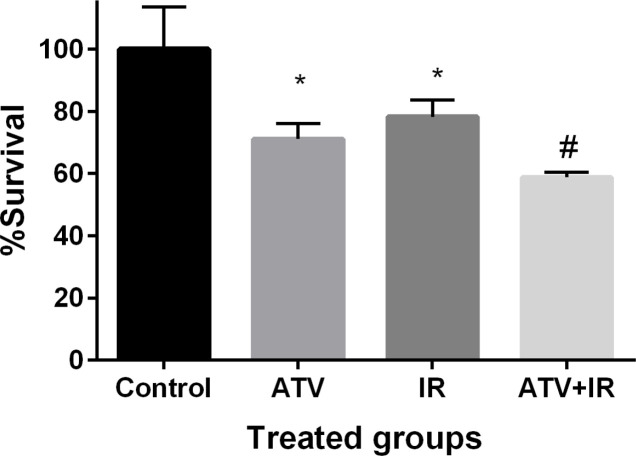
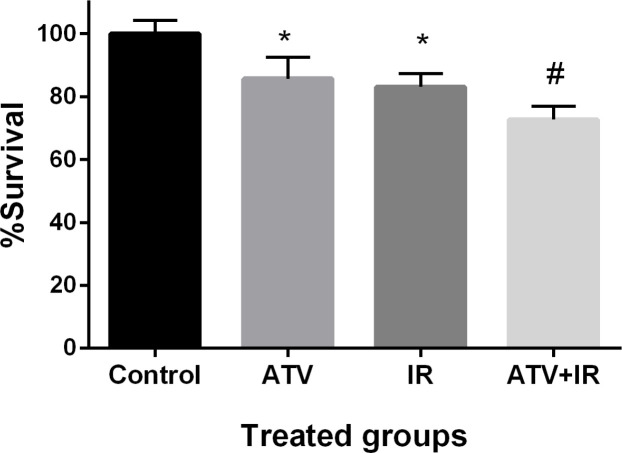
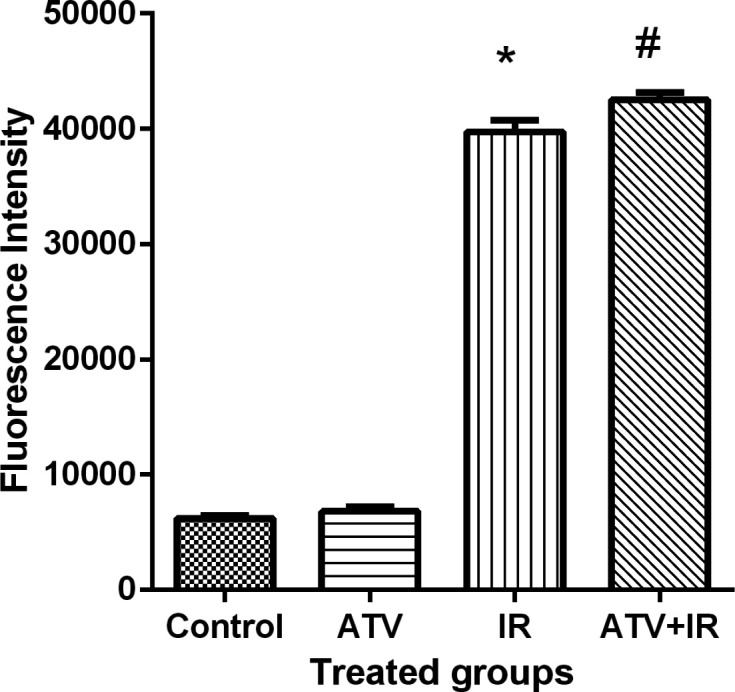
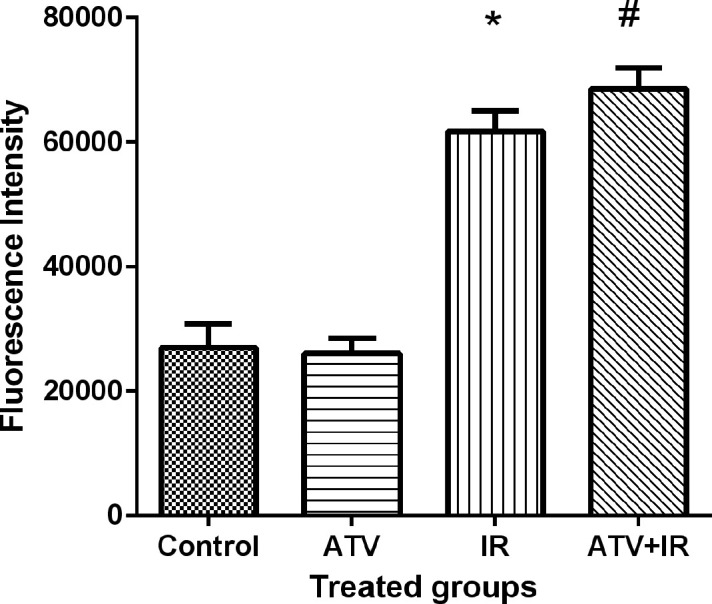
Conclusion
We demonstrated that the ATV sensitized human breast and lung cancer cells to radiation-induced apoptosis and death. Since, previously the radioprotective effects of ATV on normal cells and tissues were observed, this result provides a new indication of ATV for sensitizing the cancer cells to radiation therapy in the patients.
Acknowledgements
References
-
1.
Hosseinimehr SJ. Flavonoids and genomic instability induced by ionizing radiation. Drug Discov. Today. 2010;15:907-18. [PubMed ID: 20933097].
-
2.
Hosseinimehr SJ. The use of angiotensin II receptor antagonists to increase the efficacy of radiotherapy in cancer treatment. Future Oncol. 2014;10:2381-90. [PubMed ID: 25525846].
-
3.
Salehifar E, Hosseinimehr SJ. The use of cyclooxygenase-2 inhibitors for improvement of efficacy of radiotherapy in cancers. Drug Discov. Today. 2016;21:654-62. [PubMed ID: 26955911].
-
4.
Linam J, Yang LX. Recent developments in radiosensitization. Anticancer Res. 2015;35:2479-85. [PubMed ID: 25964520].
-
5.
Hosseinimehr SJ, Ahmadi A, Beiki D, Habibi E, Mahmoudzadeh A. Protective effects of hesperidin against genotoxicity induced by (99m)Tc-MIBI in human cultured lymphocyte cells. Nucl. Med. Biol. 2009;36:863-7. [PubMed ID: 19720298].
-
6.
Hosseinimehr SJ, Zakaryaee V, Froughizadeh M. Oral oxymetholone reduces mortality induced by gamma irradiation in mice through stimulation of hematopoietic cells. Mol. Cell. Biochem. 2006;287:193-9. [PubMed ID: 16532255].
-
7.
Srivastava M, Raghavan SC. DNA double-strand break repair inhibitors as cancer therapeutics. Chem. Biol. 2015;22:17-29. [PubMed ID: 25579208].
-
8.
Bjarnadottir O, Kimbung S, Johansson I, Veerla S, Jonsson M, Bendahl PO, Grabau D, Hedenfalk I, Borgquist S. Global transcriptional changes following statin treatment in breast cancer. Clin. Cancer Res. 2015;21:3402-11. [PubMed ID: 25840970].
-
9.
Kang M, Jeong CW, Ku JH, Kwak C, Kim HH. Inhibition of autophagy potentiates atorvastatin-induced apoptotic cell death in human bladder cancer cells in-vitro. Int. J. Mol.Sci. 2014;15:8106-21. [PubMed ID: 24815071].
-
10.
Wilke M, Gobel A, Rauner M, Benad-Mehner P, Schutze N, Fussel S, Hadji P, Hofbauer LC and Rachner TD. Zoledronic acid and atorvastatin inhibit alphavbeta3-mediated adhesion of breast cancer cells. J. Bone Oncol. 2014;3:10-7. [PubMed ID: 26909293].
-
11.
Oliveira KA, Dal-Cim T, Lopes FG, Ludka FK, Nedel CB, Tasca CI. Atorvastatin promotes cytotoxicity and reduces migration and proliferation of human A172 glioma cells. Mol. Neurobiol. 2018;55:1509-23. [PubMed ID: 28181188].
-
12.
Toepfer N, Childress C, Parikh A, Rukstalis D, Yang W. Atorvastatin induces autophagy in prostate cancer PC3 cells through activation of LC3 transcription. Cancer Biol. Ther. 2011;12:691-9. [PubMed ID: 21768780].
-
13.
Hosseinimehr SJ, Izakmehri M, Ghasemi A. In-vitro protective effect of atorvastatin against ionizing radiation induced genotoxicity in human lymphocytes. Cell. Mol. Biol.(Noisy-le-grand. 2015;61:68-71.
-
14.
Naeimi RA, Talebpour Amiri F, Khalatbary AR, Ghasemi A, Zargari M, Ghesemi M, Hosseinimehr SJ. Atorvastatin mitigates testicular injuries induced by ionizing radiation in mice. Reprod. Toxicol. 2017;72:115-21. [PubMed ID: 28668617].
-
15.
Talebpour Amiri F, Hamzeh M, Naeimi RA, Ghasemi A, Hosseinimehr SJ. Radioprotective effect of atorvastatin against ionizing radiation-induced nephrotoxicity in mice. Int. J. Radiat. Biol. 2018;94:106-113. [PubMed ID: 29268056].
-
16.
Ghasemi A, Ghashghai Z, Akbari J, Yazdani-Charati J, Salehifar E, Hosseinimehr SJ. Topical atorvastatin 1% for prevention of skin toxicity in patients receiving radiation therapy for breast cancer: a randomized, double-blind, placebo-controlled trial. Eur. J. Clin. Pharmacol. 2019;75:171-8. [PubMed ID: 30291370].
-
17.
Yazdannejat H, Hosseinimehr SJ, Ghasemi A, Pourfallah TA, Rafiei A. Losartan sensitizes selectively prostate cancer cell to ionizing radiation. Cell. Mol. Biol. 2016;62:30-3. [PubMed ID: 26828983].
-
18.
Fei Z, Gu W, Xie R, Su H, Jiang Y. Artesunate enhances radiosensitivity of esophageal cancer cells by inhibiting the repair of DNA damage. J. Pharmacol. Sci. 2018;138:131-7. [PubMed ID: 30337244].
-
19.
Hosseinimehr SJ, Safavi Z, Kangarani Farahani S, Noaparst Z, Ghasemi A, Asgarian-Omran H. The synergistic effect of mefenamic acid with ionizing radiation in colon cancer. J. Bioenerg. Biomembr. 2019;51:249-57. [PubMed ID: 30847692].
-
20.
Ji K, Sun X, Liu Y, Du L, Wang Y, He N, Wang J, Wu C, Liu Q. Regulation of apoptosis and radiation sensitization in lung cancer cells via the Sirt1/NF-kappaB/Smac pathway. Cell. Physiol. Biochem. 2018;48:304-16. [PubMed ID: 30016782].
-
21.
He Z, Yuan J, Qi P, Zhang L, Wang Z. Atorvastatin induces autophagic cell death in prostate cancer cells in-vitro. Mol. Med. Rep. 2015;11:4403-8. [PubMed ID: 25672364].
-
22.
Martinez TA, Zeybek ND, Muftuoglu S. Evaluation of the cytotoxic and autophagic effects of atorvastatin on Mcf-7 breast cancer cells. Balkan Med. J. 2018;35:256-62. [PubMed ID: 29485098].
-
23.
Li J, Liu J, Liang Z, He F, Yang L, Li P, Jiang Y, Wang B, Zhou C, Wang Y, Ren Y, Yang J, Zhang J, Luo Z, Vaziri C and Liu P. Simvastatin and atorvastatin inhibit DNA replication licensing factor MCM7 and effectively suppress RB-deficient tumors growth. Cell Death Dis. 2017;8:e2673.
-
24.
Liu H, Liang SL, Kumar S, Weyman CM, Liu W, Zhou A. Statins induce apoptosis in ovarian cancer cells through activation of JNK and enhancement of Bim expression. Cancer Chemother. Pharmacol. 2009;63:997-1005. [PubMed ID: 18766339].
-
25.
Sanchez CA, Rodriguez E, Varela E, Zapata E, Páez A, Masso F, Montaño LF, López-Marure R. Statin-induced inhibition of MCF-7 breast cancer cell proliferation is related to cell cycle arrest and apoptotic and necrotic cell death mediated by an enhanced oxidative stress. Cancer Invest. 2008;26:698-707. [PubMed ID: 18608208].
-
26.
Zhang P, He D, Song E, Jiang M, Song Y. Celecoxib enhances the sensitivity of non-small-cell lung cancer cells to radiation-induced apoptosis through downregulation of the Akt/mTOR signaling pathway and COX-2 expression. PLoS One. 2019;14:e0223760. [PubMed ID: 31613929].
-
27.
Choo DW, Goh SH, Cho YW, Baek HJ, Park EJ, Motoyama N, Kim TH, Kim JY, Kim SS. CHK2 is involved in the p53-independent radiosensitizing effects of valproic acid. Oncol. Lett. 2017;13:2591-8. [PubMed ID: 28454438].
-
28.
Qi R, Jin W, Wang J, Yi Q, Yu M, Xu S, Jin W. Oleanolic acid enhances the radiosensitivity of tumor cells under mimetic hypoxia through the reduction in intracellular GSH content and HIF-1alpha expression. Oncol. Rep. 2014;31:2399-406. [PubMed ID: 24604140].
-
29.
Kijima T, Koga F, Fujii Y, Yoshida S, Tatokoro M, Kihara K. Zoledronic acid sensitizes renal cell carcinoma cells to radiation by downregulating STAT1. PLoS One. 2013;8:e64615. [PubMed ID: 23741352].
-
30.
Patel K, Doudican NA, Schiff PB, Orlow SJ. Albendazole sensitizes cancer cells to ionizing radiation. Radiat. Oncol. 2011;6:160. [PubMed ID: 22094106].
-
31.
Gewirtz DA, Hilliker ML, Wilson EN. Promotion of autophagy as a mechanism for radiation sensitization of breast tumor cells. Radiother. Oncol. 2009;92:323-8. [PubMed ID: 19541381].
-
32.
Jones HM, Fang Z, Sun W, Clark LH, Stine JE, Tran AQ, Sullivan SA, Gilliam TP, Zhou C, Bae-Jump VL. Atorvastatin exhibits anti-tumorigenic and anti-metastatic effects in ovarian cancer in-vitro. Am. J. Cancer Res. 2017;7:2478-90. [PubMed ID: 29312801].