Abstract
Keywords
Chenopodium botrys Essential Oil Apoptosis HeLa Cell Sesquiterpenes
Introduction
Dysphania botrys (L.) (syn. Chenopodium botrys Linn.), the Jerusalem-oak, belong of Chenopodiaceae family (1), widely grows throughout Western Asia, Europe, and North America(2) . Traditionally, Chenopodium botrys (C. botrys) is used in the treatment of several conditions, such as coughing, pectoral complaints, abdominal pain, and obstruction(3). The essential oil of C. botrys is collected from Southern Serbia and Greece, exhibiting significant bactericidal and fungicidal effects (4). The concentration and composition of C. botrys in its essential may vary between 0.08-2% in different regions(5). Based on previous studies, essential oil of C. botrys is composed of monoterpenes (camphor, δ-3-carene, fenchone, linalool, menthone, nerol, β-pinene, pulegone, terpineol-4, and thujone) and sesquiterpenes (β-elemene, elemol and β-eudesmol) (6). Furthermore, there are evidences that sesquiterpenes as β-eudesmol are anti-cancer natural products (7).
Apoptosis is defined as cellular self-destruction or programmed cell death. Studies have confirmed the association of apoptosis and cancer. Moreover, growing evidence suggests that the process of neoplastic transformation, progression, and metastasis involve changes in normal apoptotic pathways (8).
The main apoptosis signaling pathways are extrinsic (death receptor) and intrinsic pathways (mitochondria). The intrinsic pathway is initiated by stimulants such as anticancer drugs, growth factor withdrawal, and hypoxia or via the induction of oncogenes, which leads to the permeabilization of the external mitochondrial membrane and activates the mitochondrial pathway. The mitochondrial pathway is initiated with the release of apoptogenic agents (e.g., cytochrome C) from the mitochondrial intermembrane space into the cytosol. Release of cytochrome C into the cytosol leads to the activation of caspase-3 gene through the formation of the apoptosome complex (9).
Bcl-2 family proteins that regulate mitochondria-mediated apoptosis include the upstream of caspase activation. In addition, the Bcl-2 family consists of anti-apoptotic proteins (e.g., Bcl-2, Bcl-xL, and Mcl-1) and some pro-apoptotic proteins (e.g., Bax, Bad, and Bim). Overexpression of Bcl-2 anti-apoptotic proteins blocks external mitochondrial membrane permeabilization and inhibits apoptosis (10, 11). It has been suggested that a mediator that could inhibit and/or increase the expression of anti-apoptotic proteins might induce apoptosis in cancer cells.
Human cervical cancer is the second common cause of cancer-related deaths among women worldwide (12). A significant proportion of the patients with cervical cancer are irresponsive to chemotherapy due to the drug resistance of tumors or tremendous drug side-effects (12, 13).
Cancer is characterized by the loss of control over cell growth (14). The tumor suppressor protein p21 (WAF1/Cip1) is a universal cyclin-dependent kinase inhibitor (CDKI), which regulates cell progression through the cell cycle (15). The induction of p21 has been observed to be straightly mediated by p53, causing an arrest in the cell cycle at the G1 phase(16, 17) .
In cancer cells, p21 expression is generally independent of wild-type p53 expression. Increased expression of p21 is associated with cell proliferation arrest and metastatic suppression(18, 19). As a tumor suppressor protein, p53 controls plays numerous physiological roles in cell cycle arrest, DNA repair, apoptosis, translation, and differentiation (17, 18).
There are several experiments on antimicrobial and anti-inflammatory activity of C. botrys essential oil(2, 5). Nevertheless, there are no investigations on anti-cancer effect of this essential oil. According to previous reports on anti-cancer effects of eudesmol compound (7) and presence of this sesquiterpene in C. botrys essential oil (6), the present study aimed to elucidate that C. botrys essential oil could induce apoptosis and arrest the cell cycle in HeLa cells.
Experimental
Materials
Collection of Plants
Aerial parts of C. botrys were collected at the altitude of 1,200 meters in the north of Sabzevar, located in Razavi Khorasan province in the northeast of Iran in June 2017. The samples were identified by Souzani at the Herbarium of School of Pharmacy, Mashhad University of Medical Sciences in Mashhad, Iran where the voucher specimen of the plant was deposited. After the hydro-distillation of C.botrys aerial parts (100 grams) for two hours, the herbal essential oil was prepared using a Clevenger apparatus. Afterwards, the essential oil was passed over anhydrous sodium sulfate in order to obtain a dried, slightly yellow oil. The essential oil was subjected to cytotoxic assay, and the herbal essential oil was subjected to GC-MS analysis.
Cell culture
Human cervial carcinoma cell line (HeLa cells) was purchased from Pasteur Institute of Iran. Dulbecco’s Modified Eagle’s Medium (DMEM GIBCO, England) supplemented with 10% fetal bovine serum (FBS, GIBCO, England) and 1% penicillin/streptomycin (100 U/mL of penicillin G, 100 μg/mL of streptomycin, Biosera, England) was used for the HeLa cells.
Methods
Analysis of C. botrys Essential Oil
The essential oil of C. botrys was analyzed using GC/MS apparatus (GC7890N Agilent MS-5975) to characterize the chemical constituents. The system was equipped with an HP-5MS capillary column (30 m×0.25 mm with film thickness of 0.25 μm). The condition of temperature programming was based on the protocol of helium carrier gas, adjusted to a linear velocity of 1 mL/min, and the column had the split ratio of 50:1. The injector temperature was regulated at 260 °C.
In the next stage, the temperature of the oven column was maintained at 60 °C for four minutes, programmed to 100 °C at 3 °C/min for two minutes, and to 250 °C at 6 °C/min for seven minutes with the electron energy of 70 eV. To observe the positive ions, the analyzer was scanned from the mass of 50 to 550. Additionally, a mixture of homologous series of n-alkanes C9-C23 was used on the HP-5MS column, and the Kovats indices of the chemical constituents were determined. Constituents of the essential oil were characterized by the comparison of their mass spectra with those of the NIST-5 mass spectra library.
Cytotoxicity Assay
In the present study, cytotoxic effects of C. botrys essential oil on HeLa cell proliferation was evaluated using the 3-(4, 5-dimethylthi-azol-2-yl)-2, 5-diphenyltetrazolium bromide (MTT) assay. The cells were evenly distributed (5×103 cells/well) on 96-well plates (Iwaki, Tokyo, Japan) and incubated in a humidified incubator at the temperature of 37 ℃ with 5% CO2 overnight.
In the next stage, after optimizing the concentration range for C. botrys essential oil from 0-1mg/mL, the cells were treated by C. botrys essential oil at the concentrations of zero, 5, 10, 30, 50, 75, 100, 130, 170, and 200 µg/mL (essential oil solved in DMSO and added cell culture medium) and incubated for 24 h. Following that, the medium in each well was replaced with 20 µL MTT (5 mg/mL in phosphate-buffered saline (PBS)) and incubated at the temperature of 37 ˚C for four hours. The purple-blue formazan crystals were dissolved in 100 µL of dimethyl sulfoxide, and the absorbance was measured at the wavelengths of 510 and 630 nm (control wavelengths) on a 96-well plate reader (Thermo Lab Systems, Franklin, MA, USA). Afterwards, the concentrations of the essential oil that achieved 50% mortality (IC50) were calculated. Data analysis was performed using GraphPad Prism (Advanced Graphics Software, Inc., Rancho, Santa Fe, CA, USA).
LDH Assay
Lactate dehydrogenase (LDH) levels were measured as the marker of membrane disintegration and permeability increase in the supernatant of the treated and control cells. Moreover, the supernatants containing LDH released from the treated and untreated HeLa cells in 96-well plates were assessed based on the reduction of NAD+ at the wavelength of 330 nm by the Deutsche Gesellschaft Für Klinische Chemie method using an auto-analyzer (Biotechnica, BT 1500, Rome, Italy) and commercial kit (Pars Azmoon, Tehran, Iran).
Apoptosis Assay
Apoptosis of the treated and untreated HeLa cells was measured by flow cytometry using Annexin V-FITC/PI detection kit (BD, Biosciences Pharmingen) in accordance with the instructions of the manufacturer. HeLa cells (2.5×105 cells/well) were treated with various concentrations of the essential oil of C. botrys (50 and 80 µg/mL as IC50 and 100 µg/mL) in six-well plates for 24 h. Afterwards, the cells were detached using trypsin and collected and washed twice with cold PBS.
The cells were resuspended in Annexin V-binding buffer and incubated with 5 µL of Annexin V-FITC and 5 µl of propidium iodide (PI) for 15 min at room temperature in the dark. Apoptotic and necrotic cell populations were determined on FACSCalibur flow cytometer (BD Biosciences, USA), and the data were analyzed using the CellQuest Pro software package (BD Biosciences, USA). PI-/Annexin V- cells were considered as viable cells, early apoptosis was defined in PI-/Annexin V+ cells, and late apoptosis or necrosis was considered in PI+/Annexin V+ cells.
Cell Cycle Analysis
The HeLa cells with exponential growth were seeded in six-well plates (2.5×105 cells/well) and treated by the essential oil of C. botrys at the concentrations 50, 80, and 100 for 24 h. Subsequently, the cells were collected and incubated with 20 μg/mL of RNase A and 10 μg/mL of PI in the dark for 30 min. After staining, cell cycle phase distribution and hypodiploid DNA were detected by FACSCalibur flow cytometer (BD Biosciences, USA) at the wavelength of 488 nm and analyzed using the FlowJo 3.1 software (FlowJo, Oregon, USA).
Real-time PCR
For gene expression analysis, quantitative real-time polymerase chain reaction (PCR) was performed. HeLa cells (2.5×105 cells/well) were cultured in six-well plates and treated with the essential oil of C. botrys at the concentrations of 50, 80, and 100 μg/mL. Total RNA extraction was performed using AccuZol™ Total RNA Extraction Solution (Bioneer, Korea) obtained from the treated and untreated cells. In addition, cDNA synthesis was conducted using 2 μg RNA (Takara Bio, Shiga, Japan).
SYBR Green PCR Master Mix was applied to determine the expression of Bax, Bcl2, caspase-3, p53, p21, and glyceraldehyde-3-phosphate dehydrogenase (GAPDH) Homo sapiens gene. Moreover, quantitative PCR reactions were performed in a 20-µL volume containing 0.5 µL of each of the forward and reverse primers, 2 µL of cDNA, 10 µL of SYBR Green PCR Master Mix, and 7 µL of Diethyl pyrocarbonate water in accordance with the instructions of the manufacturer.
After the initial 10 min at the temperature of 94 °C as the activation stage, 35 cycles, including 15 seconds of denaturation at 95 °C, 20 seconds of annealing at 60 °C for p21 and p53 and 57 °C for Bax, Bcl2, and caspase-3, and 20 seconds of extension at 72 °C, were carried out using BioRad CFX96 Well Real-Time PCR detection system (Bio-Rad Laboratories, Hercules, CA, USA). Primer sequences and fragments sizes are presented in Table 1.
Statistical analysis
Statistical analysis were done by using the SPSS 16.0 software package (SPSS GmbH Software, Germany). The data were presented as mean ± SEM. Comparisons were made using the one-way ANOVA (followed by the post-hoc LSD test) and Brown-Forsythe analysis (followed by the post-hoc Dunnett T3 test). P-values less than 0.05 were considered as level of significance. Graph Pad Prism5 software package was used (Inc.; San Diego CA, USA, 2003) for drawing the statistical graphs.
Result and Discussion
Chemical Composition of C. botrys Essential Oil
The essential oil of C. botrys was isolated from the dried aerial parts of the plant through a hydro-distillation process with a yield of 0.97% (w/w) based on the dry weight of C. botrys. In total, 37 constituents were detected in the herbal essential oil by GC-MS analysis, as well as their composition and retention index. Accordingly, the essential oil of C. botrys was composed of monoterpenes (0.92%), oxygenated monoterpenes (2.68%), sesquiterpenes (13.71%), oxygenated sesquiterpenes (64.17%), and other components (4.06%). The major constituents of C. botrys essential oil were α-Eudesmol (16.81%), Elemol acetate (13.2%), Elemol (9.0%), and α-Chenopodiol-6-acetate (7.9%).
Cytotoxicity Induction by C. botrys Essential Oil
In the present study, a broad spectrum of the cytotoxic effects C. botrys essential oil on HeLa human cancer cell line was investigated using the MTT assay. Cell viability upon the treatment of cells with increasing concentrations of the herbal essential oil (5-200 µg/mL) was assessed for 24 h. According to the results, the essential oil of C. botrys exhibited concentration-dependent cytotoxic effects on the cell line at 24 h. Furthermore, C. botrys essential oil exerted significant cytotoxic effects on the HeLa cells with the IC50 of 79.62 µg/mL after treatment for 24 h (Figure 1).
Apoptosis Induction by C. botrys Essential Oil in Cervical Cancer Cells
To determine whether the loss of the cell viability of cervical cancer cells induced by C. botrys essential oil was associated with the induction of apoptosis, the HeLa cells were treated by C. botrys essential oil, and the number of the apoptotic cells was determined using the Annexin V-FITC and PI detection kit as previously described.
Apoptotic cells were characterized as early (Annexin V+) and late (Annexin V+/PI+) apoptotic cells. Treatment of the HeLa cells with the essential oil of C. botrys led to a significant dose-dependent increase (p ˂ 0.001) in the number of apoptotic cells from 0 µg/mL to 80 µg/mL and 100 µg/mL, as follows: 0 µg/mL (mean ± SEM: 8.14±0.65), 50 µg/mL (7.53 ± 0.93), 80 µg/mL (68.4 ± 0.91), and 100 µg/mL (87.3 ± 1.95) (Figure 2).
Induction of Cell Cycle Arrest in G1 Phase in Cervical Cancer Cells by C. botrys Essential Oil
Percentage of the cervical cancer cells in various phases of the cell cycle was investigated using the flow cytometry of PI-labeled HeLa cells after 24 h of treatment with C. botrys essential oil. Treatment of the HeLa cells by C. botrys essential oil caused an increase in the G0/G1 phases and a reduction in the G2 and S phases, which indicated cell cycle arrest in the G1 phase (Figure 3).
Effects of C. botrys on the Expression of Bcl-2 Family Members and Caspase-3
To evaluate the status of the intrinsic signaling of apoptosis in mitochondria, mRNA expression levels of the pro-apoptotic (Bax) and anti-apoptotic genes (Bcl2) were measured using real-time PCR. Moreover, we examined caspase involved in C. botrys-induced apoptosis by evaluating the mRNA expression level of caspase-3. The essential oil of C. botrys significantly increased the expression of Bax (p ˂ 0.001, between 0 µg/mL to100 µg/mL) and caspase-3 (p ˂ 0.001). Furthermore, the ratio of Bax/Bcl2 had a five-fold increase from the concentration of 50 to 100 µg/mL (p ˂ 0.001). Bcl2 gene expression had no significant difference between the groups (Figure 4A).
Effects of C. botrys Essential Oil on the Expression of p21 and p53
To assess the status of the HeLa cells in cell growth arrest, we evaluated the expression levels of p21 and p53 genes. According to the findings, the essential oil of C. botrys significantly increased the expression of p21 (p ˂ 0.001) and p53 (p ˂ 0.001) in a concentration-dependent manner, especially in the case of p21 (Figure 4B).
Phytochemicals may affect the signaling pathways within the cells, including those involved in the regulation of cell proliferation and activation of apoptosis(20). Apoptosis, also known as programmed cell death, is a pathway through which cells undergo death to control cell proliferation or react to DNA damage (21). Activation of these pathways is a major mechanism, through which cytotoxic drugs eradicate tumor cells (22). In the current research, we evaluated the apoptosis induced by C. botrys essential oil in HeLa cells and determined the involved molecular mechanisms that occur via the intrinsic pathway.
The results of GC/MS analysis showed 37 compounds representing 85.54% of the total contents of the essential oil (Table 2). Moreover, the results indicated that C. botrys is an abundant source of monoterpenes, especially sesquiterpenes (78.88%). Several studies have demonstrated that the major compounds of C. botrys essential oil are α- and β-eudesmol(23, 24), α- and β-chenopodiol (25), camphor, and elemol (26). However, the main components were noted as α-eudesmol (16.81%), Elemol acetate (13.2%), Elemol (9.0%), and α-Chenopodiol-6-acetate in the current research. In the present study, we investigated the cytotoxic effects of C. botrys essential oil on cervical cancer cells, and the herbal essential oil was considered to be a potential cytotoxic agent in the treatment of HeLa cells by killing the cancer cells in a dose-dependent manner. In particular, treatment of the HeLa cells with 100 µg/mL of C. botrys essential oil for 24 h could reduce the viability of cervical cancer cells by 81.14 ± 6.87% (IC50:79.64 µg/mL), which indicated the potential to eliminate cancer invasiveness.
The anti-tumor effects of the herbal essential oil could be attributed to cytostatic and/or cytotoxic function. Cytostatic substances are able to prevent cell growth and/or induce cell cycle arrest at various cell cycle checkpoints. On the other hand, cytotoxic substances induce cell death through apoptosis or necrosis (29). Therefore, we demonstrated a mild elevation in the leakage of LDH by increasing the concentration of the essential oil. Moreover, LDH leakage had a significant adjustment at the IC50 dosage of C. botrys essential oil.
In the current research, apoptosis was followed by cell cycle arrest in the G1 phase, which increased the cells in the sub-G1 (G0) phase and decreased cells in the G2 and S phases (Figures 2 & 3). This finding is supported by the overexpression of p21 and p53 genes. P21 is known to act as an essential inhibitor of CDK2, which is activated in response to various cellular and environmental signals to improve tumor suppressor events (30).
In the present study, the wild-type tumor suppressor p53 and p21 of the HeLa cells was up-regulated (Figure 4B). Therefore, it could be concluded that the up-regulation of p53 by C. botrys essential oil triggers the accumulation of p21 protein, leading to G0/G1 phase cell cycle arrest and apoptosis in HeLa cells (31-33). It is also notable that p53/p21 is involved in the control of cell cycle, apoptosis, and maintenance of genomic stability (34). Once DNA damage occurs, wild-type p53 is expressed to induce cell cycle arrest at various checkpoints in order to repair the damaged DNA. As a result, unrepaired DNA cells are targeted by apoptosis (32). Furthermore, this is accompanied by an increase in the expression of the Bax and Bax/Bcl2 ratio. The cytosolic section of p53 activates the apoptotic-effector protein Bax to initiate apoptosis (35). The damaged cells could be repaired to maintain the integrity of the cell or be cleared by apoptosis to remove a cancerous cell through G1 phase arrest. Apoptosis is an aspect of mammalian cell behavior, which is of utmost importance in the growth and development in tumor oncogenesis.
Bcl2 and Bax are the critical regulators of cell apoptosis with remarkable anti-apoptotic and pro-apoptotic effects, respectively (36). According to the literature, susceptibility to apoptosis is likely to be determined based on the Bax/Bcl2 ratio as the key regulators of apoptosis located mainly at the outer membrane of the mitochondria and endoplasmic reticulum. In the present study, their regulation of apoptosis was mainly achieved through the mitochondria. Overexpression of Bcl2 leads to resistance to apoptosis, whereas overexpression of Bax results in increased apoptosis (36, 37).
Our findings indicated the adjustment of the expression of Bcl2 and Bax compared to the control group. Bcl2 expression decreased significantly, while Bax expression had a statistically significant increase after treatment with C. botrys essential oil. Moreover, the Bax/Bcl2 ratio and caspase-3 elevated in the HeLa cells after treatment with C. botrys essential oil, which is consistent with the results regarding cell apoptosis.
According to the results of the present study, C. botrys essential oil induced intrinsic apoptosis through adjusting the expression of Bcl2 and Bax to enhance their ratio, followed by the triggering of the caspase cascade. Therefore, it could be concluded that C. botrys essential oil could potentially eliminate cancer cells by conducting apoptosis pathways.
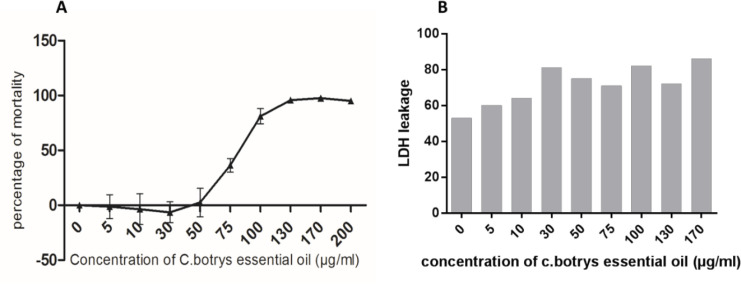
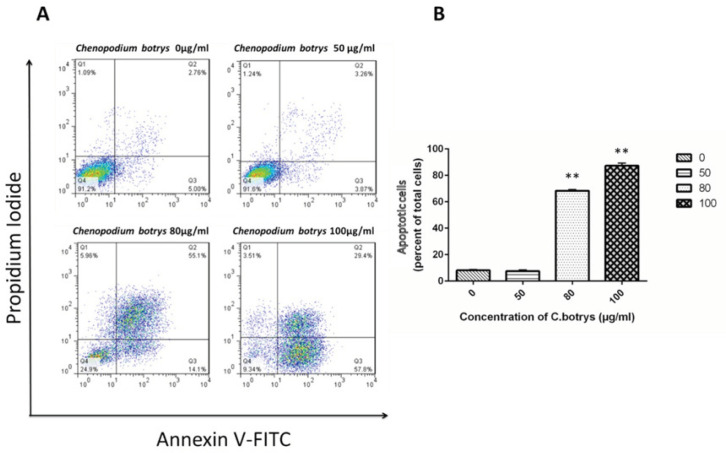
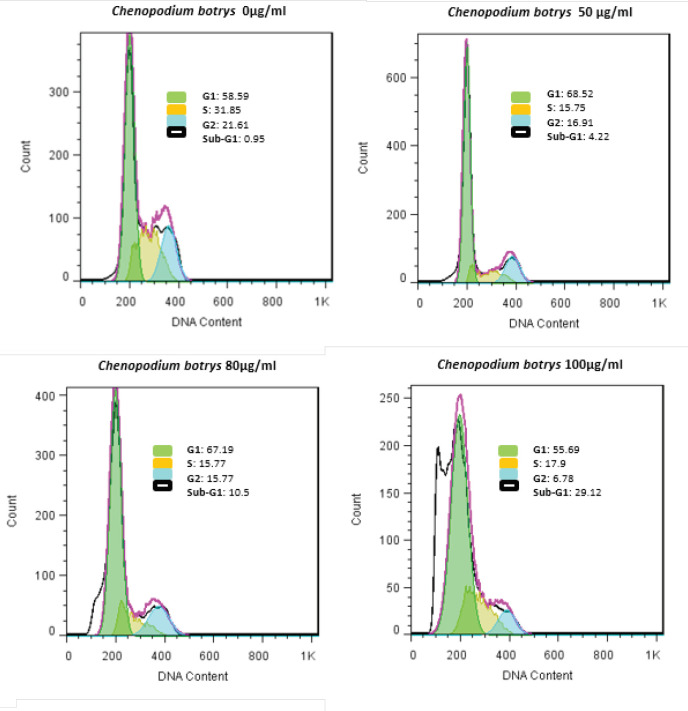
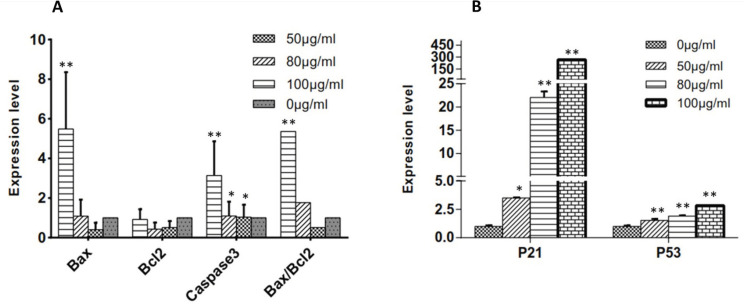
Primer Sequences
Gene | Sequence | bp | |
---|---|---|---|
Bax | Forward | CGCCCTTTTCTACTTTGCCA | 166 |
Reverse | GTGAGGAGGCTTGAGGAGTC | ||
Bcl2 | Forward | GCCTTCTTTGAGTTCGGTGG | 192 |
Reverse | GAAATCAAACAGAGGCCGCA | ||
Caspase-3 | Forward | ACTGGACTGTGGCATTCAGA | 162 |
Reverse | GCACAAAGCGACTGGATGAA | ||
p53 | Forward | CCCCTCCTGGCCCCTGTCATCTTC | 265 |
Reverse | GCAGCGCCTCACAACCTCCGTCAT | ||
p21 | Forward | CGCATGGGTTCTGACGGACATCC | 257 |
Reverse | TGCCGAAGTCAGTTCCTTGTGG | ||
GAPDH | Forward | GGAAGGTGAAGGTCGGAGTCA | 101 |
Reverse | GTCATTGATGGCAACAATATCCACT |
Chemical Composition of C. botrys Essential Oil
Class | RIL3 | RIL2 | RIL1 | Kovats Retention Index (RIa) | % | Rt (min) | Compounds | No. |
---|---|---|---|---|---|---|---|---|
MH | 991 | 990 | 981 | 981.8085 | 0.60 | 4.986 | β-Myrcene | 1 |
MH | 1031 | 1030 | 1014 | 1017.147 | 0.32 | 6.143 | Limonene | 2 |
MO | 1096 | 1073.13 | 0.97 | 8.239 | α-Thujone | 3 | ||
MO | 1145 | 1124 | 1126.591 | 0.22 | 10.377 | p-Menth-2-en-1-ol | 4 | |
MO | 1177 | 1180 | 1169 | 1162.908 | 0.35 | 11.923 | 4-Terpineol | 5 |
MO | 1193 | 1187 | 1177.402 | 0.52 | 12.54 | α-Terpineol | 6 | |
MO | 1285 | 1285 | 1270.805 | 0.36 | 16.582 | Bornyl acetate | 7 | |
MO | 1354 | 1354 | 1354 | 1336.388 | 0.26 | 19.607 | citronellyl acetate | 8 |
SH | 1391 | 1395 | 1382 | 1378.25 | 2.64 | 21.626 | β-elemene | 9 |
SH | 1425 | 1420 | 1398.155 | 1.42 | 22.586 | Trans-caryophyllene | 10 | |
SH | 1460 | 1451 | 1433.083 | 0.28 | 23.955 | α-Humulene | 11 | |
SO | 1454 | 1442.879 | 0.23 | 24.334 | Trans-geranylacetone | 12 | ||
SH | 1470 | 1465.96 | 0.52 | 25.227 | β-Eudesmene | 13 | ||
SH | 1485 | 1484.053 | 1.13 | 25.927 | Aromadendrene | 14 | ||
SH | 1505 | 1494.908 | 1.40 | 26.347 | γ-Cadinene | 15 | ||
SH | 1524 | 1516 | 1505.52 | 0.45 | 26.726 | δ-Cadinene | 16 | |
SO | 1539 | 1517.956 | 0.68 | 27.136 | α-Copaen-11-ol | 17 | ||
SO | 1540 | 1547 | 1537.307 | 9.02 | 27.774 | Elemol | 18 | |
SH | 1556 | 1562 | 1551.319 | 0.85 | 28.236 | Germacrene B | 19 | |
SO | 1583 | 1578 | 1561.541 | 0.95 | 28.573 | Caryophyllene Oxide | 20 | |
SO | 1644 | 1624.721 | 0.27 | 30.571 | γ-Eudesmol | 21 | ||
SO | 1653 | 1641.754 | 16.81 | 31.074 | α-Eudesmol | 22 | ||
SO | 1675 | 1667.931 | 13.17 | 31.847 | Elemol acetate | 23 | ||
SO | 1678 | 1673.383 | 1.40 | 32.008 | botrydiol | 24 | ||
SO | 1691 | 1682 | 1678.835 | 1.13 | 32.169 | juniper camphor | 25 | |
SO | 1729 | 1696.749 | 0.42 | 32.698 | α-Costol | 26 | ||
SO | 1724 | 1736 | 1701.249 | 0.55 | 32.828 | guaiol acetate | 27 | |
SO | 1778 | 1782 | 1770.242 | 5.05 | 34.706 | γ-eudesmol acetate | 28 | |
SO | 1789 | 1785 | 1779.023 | 2.44 | 34.945 | α-eudesmol acetate | 29 | |
SO | 1791 | 1788.758 | 4.53 | 35.21 | β-Eudesmol acetate | 30 | ||
SO | 1830 | 1866.967 | 4.30 | 37.223 | β-Chenopodiol | 31 | ||
SO | 1876 | 1875.52 | 0.38 | 37.441 | α-Chenopodiol | 32 | ||
SO | 1904 | 1883.837 | 0.55 | 37.653 | β-Chenopodiol-6-acetate | 33 | ||
NH | 1928 | 1917.371 | 0.66 | 38.484 | Methyl Palmitate | 34 | ||
SO | 1957 | 1930.473 | 2.15 | 38.8 | Acetoxyeudesman-4-α-ol-11 | 35 | ||
SO | 1977 | 1940.796 | 7.88 | 39.049 | α-Chenopodiol-6-acetate | 36 | ||
NH | 2128 | 2113.458 | 0.68 | 43.065 | Methyl Stearate | 37 | ||
85.54 | Total Identified Chemicals | |||||||
0.92 | Monoterpenes (MH) (%) | |||||||
2.68 | Oxygenated Monoterpenes (MO) (%) | |||||||
13.71 | Sesquiterpenes (SH) (%) | |||||||
64.17 | Oxygenated Sesquiterpenes (SO) (%) | |||||||
1.34 | Non terpene hydrocarbon (NH) |
Acknowledgements
References
-
1.
Mosyakin SL, Clemants SE. Further transfers of glandular-pubescent species from Chenopodium subg Ambrosia to Dysphania (Chenopodiaceae). J. Bot. Res. Inst. Texas. 2008;2:425-31.
-
2.
Maksimovic ZA, Dordevic S, Mraovic M. Antimicrobial activity of Chenopodium botrys essential oil. Fitoterapia. 2005;76:112-4. [PubMed ID: 15664473].
-
3.
Bano A, Ahmad M, Hadda TB, Saboor A, Sultana S, Zafar M, Khan MPZ, Arshad M, Ashraf MA. Quantitative ethnomedicinal study of plants used in the skardu valley at high altitude of Karakoram-Himalayan range, Pakistan. J. Ethnobiol. Ethnomed. 2014;10:43.
-
4.
Tzakou O, Pizzimenti A, Pizzimenti F, Sdrafkakis V, Galati E. Composition and antimicrobial activity of Chenopodium botrys L. Essential oil from Greece. J. Essent. Oil Res. 2007;19:292-4.
-
5.
Morteza-Semnani K. A Review on Chenopodium botrys L: traditional uses, chemical composition and biological activities. Pharm. Biomed. Res. 2015;1:1-9.
-
6.
Buchbauer G, Jirovetz L, Wasicky M, Walter J, Nikiforov A. Headspace Volatiles of Chenopodiumbotrys (Chenopodiaceae). J. Essent. Oil Res. 1995;7:305-8.
-
7.
Li Y, Li T, Miao C, Li J, Xiao W, Ma E. β‐Eudesmol induces JNK‐dependent apoptosis through the mitochondrial pathway in HL60 cells. Phytother Res. 2013;27:338-43. [PubMed ID: 22585533].
-
8.
Bold RJ, Termuhlen PM, McConkey DJ. Apoptosis, cancer and cancer therapy. Surg. Oncol. 1997;6:133-42. [PubMed ID: 9576629].
-
9.
Riedl SJ, Salvesen GS. The apoptosome: signalling platform of cell death. Nat. Rev. Mol. Cell Biol. 2007;8:405. [PubMed ID: 17377525].
-
10.
Gillings AS, Balmanno K, Wiggins CM, Johnson M, Cook SJ. Apoptosis and autophagy: BIM as a mediator of tumour cell death in response to oncogene‐targeted therapeutics. The FEBS J. 2009;276:6050-62. [PubMed ID: 19788418].
-
11.
Tait SW, Green DR. Mitochondria and cell death: outer membrane permeabilization and beyond. Nat. Rev. Mol. Cell Biol. 2010;11:621. [PubMed ID: 20683470].
-
12.
Ferlay J, Soerjomataram I, Dikshit R, Eser S, Mathers C, Rebelo M, Parkin DM, Forman D, Bray F. Cancer incidence and mortality worldwide: sources, methods and major patterns in GLOBOCAN 2012. Int J Cancer. 2015;136:E359-E86. [PubMed ID: 25220842].
-
13.
Xue Y, Toh SY, He P, Lim T, Lim D, Pang CL, Abastado J-P, Thierry F. HPV16-E2 induces prophase arrest and activates the cellular DNA damage response in vitro and in precursor lesions of cervical carcinoma. Oncotarget. 2015;6:34979. [PubMed ID: 26474276].
-
14.
Clarke MF, Fuller M. Stem cells and cancer: two faces of eve. Cell. 2006;124:1111-5. [PubMed ID: 16564000].
-
15.
Xiong Y, Hannon GJ, Zhang H, Casso D, Kobayashi R, Beach D. p21 is a universal inhibitor of cyclin kinases. Nature. 1993;366:701.
-
16.
Harper JW, Adami GR, Wei N, Keyomarsi K, Elledge SJ. The p21 Cdk-interacting protein Cip1 is a potent inhibitor of G1 cyclin-dependent kinases. Cell. 1993;75:805-16. [PubMed ID: 8242751].
-
17.
El-Deiry WS. p21/p53, cellular growth control and genomic integrity.” Cyclin dependent kinase (CDK) inhibitors. Heidelberg; 1998. p. 121-137.
-
18.
Jiang H, Lin J, Su Z, Herlyn M, Kerbel RS, Weissman BE, Welch DR, Fisher PB. The melanoma differentiation-associated gene mda-6, which encodes the cyclin-dependent kinase inhibitor p21, is differentially expressed during growth, differentiation and progression in human melanoma cells. Oncogene. 1995;10:1855-64. [PubMed ID: 7753561].
-
19.
Vidal M, Loganzo JF, Hayward N, Albino A. Mutations and defective expression of the WAF1 p21 tumour-suppressor gene in malignant melanomas. Melanoma Res. 1995;5:243-50. [PubMed ID: 7496159].
-
20.
Srivastava S, Somasagara RR, Hegde M, Nishana M, Tadi SK, Srivastava M, Choudhary B, Raghavan SC. Quercetin, a natural flavonoid interacts with DNA, arrests cell cycle and causes tumor regression by activating mitochondrial pathway of apoptosis. Sci. Rep. 2016;6:24049. [PubMed ID: 27068577].
-
21.
Ghobrial IM, Witzig TE, Adjei AA. Targeting apoptosis pathways in cancer therapy. CA-Cancer J. Clin. 2005;55:178-94. [PubMed ID: 15890640].
-
22.
Debatin K-M. Apoptosis pathways in cancer and cancer therapy. Cancer Immunol. Immunother. 2004;53:153-9. [PubMed ID: 14749900].
-
23.
Kletter C, Kriechbaum M. Tibetan medicinal plants. CRC Press; 2001.
-
24.
El-Sayed AM, Al-Yahya M, Hassan MM. Chemical composition and antimicrobial activity of the essential oil of Chenopodium botrys growing in Saudi Arabia. Int. J. Crude Drug Res. 1989;27:185-8.
-
25.
Bedrossian AG, Beauchamp PS, Bernichi B, Dev V, Kitaw KZ, Rechtshaffen H, Bottini AT, Hope H. Analysis of North American Chenopodium botrys essential oil isolation and structure of two new sesquiterpene alcohols. J. Essent. Oil Res. 2001;13:393-400.
-
26.
Feizbakhsh A, Sedaghat S, Tehrani MS, Rustaiyan A, Masoudi S. Chemical composition of the essential oils of Chenopodium botrys L from two different locations in Iran. J. Essent. Oil Res. 2003;15:193-4.
-
27.
Bojilov D, Dagnon S, Ivanov I. Constituent composition of Chenopodium botrys essential oil. Bulg. Chem. Commun. 2017;49:124-9.
-
28.
Chalabian F, Monfared A, Larijani K, Saldoosi S. Comparison of the Essential Oils of Chenopodium botrys L Ferulago subvelutina Rech F Rosa gallica L and Antimicrobial Activity of the Oils against some Microbes. IJMAPR. 2006;22:146-54.
-
29.
Pachon G, Rasoanaivo H, Azqueta A, Rakotozafy J, Raharisololalao A, De Cerain AL, De Lapuente J, Borras M, Moukha S, Centelles J. Anticancer effect of a new benzophenanthridine isolated from Zanthoxylum madagascariense (Rutaceline). In vivo. 2007;21:417-22. [PubMed ID: 17436597].
-
30.
Abbas T, Dutta A. p21 in cancer: intricate networks and multiple activities. Nat. Rev. Cancer. 2009;9:400. [PubMed ID: 19440234].
-
31.
Xia M, Knezevic D, Vassilev L. p21 does not protect cancer cells from apoptosis induced by nongenotoxic p53 activation. Oncogene. 2011;30:346. [PubMed ID: 20871630].
-
32.
Taylor WR, Grabovich A. Targeting the cell cycle to kill cancer cells. Pharmacology. Elsevier. 2009:429-53.
-
33.
Alkhalaf M, El-Mowafy A. Overexpression of wild-type p53 gene renders MCF-7 breast cancer cells more sensitive to the antiproliferative effect of progesterone. J. Endocrinol. 2003;179:55-62. [PubMed ID: 14529565].
-
34.
Antony ML, Kim S-H, Singh SV. Critical role of p53 upregulated modulator of apoptosis in benzyl isothiocyanate-induced apoptotic cell death. PLoS One. 2012;7:e32267. [PubMed ID: 22359675].
-
35.
Follis AV, Llambi F, Merritt P, Chipuk JE, Green DR, Kriwacki RW. Pin1-induced proline isomerization in cytosolic p53 mediates BAX activation and apoptosis. Mol. Cell. 2015;59:677-84. [PubMed ID: 26236013].
-
36.
Kanthan R, Senger J-LB, Diudea D. Malignant mixed Mullerian tumors of the uterus: histopathological evaluation of cell cycle and apoptotic regulatory proteins. World J. Surg. Oncol. 2010;8:60. [PubMed ID: 20642852].
-
37.
Krajewski S, Krajewska M, Shabaik A, Miyashita T, Wang HG, Reed JC. Immunohistochemical determination of in vivo distribution of Bax, a dominant inhibitor of Bcl-2. Am. J. Pathol. 1994;145:1323.