Abstract
Keywords
Noscapine NO Oxygen-glucose deprivation Cortical culture Sigma receptor Intracellular calcium
Introduction
Excitotoxicity and excessive intracellular calcium play central roles in causing neurodegenerative disease such as stroke and traumatic brain injury, amyotrophic lateral sclerosis, Alzheimer’s disease, and Parkinson’s disease (1, 2). Although in excitotoxicity process, glutamate has an important role in cerebral injury subsequent to stroke, sigma receptor agonists are also effective in reducing in-vitro ischemic process by diminishing the glutamate release and blocking the excessive intracellular calcium (2–4).
Initially it was believed that the sigma receptors were a subtype of opioid receptor; however, further studies have shown that these receptors are different, independent classes of proteins and have a distinct pharmacological profile consisting of two subtypes termed sigma-1 and sigma-2 (5–8). Both subtypes of receptors are widely distributed in different parts of the CNS, e.g. prefrontal cortex, hippocampus, striatum, and several other tissues, including the immune and endocrine systems (2, 6 and 9).
These receptors have many physiological and pathophysiological roles in learning and memory, movement disorders, drug addiction, amnesia, pain, depression, Alzheimer’s disease, stroke, retinal neuroprotection, HIV infection, and cancer. So, they are candidate as a therapeutic target for various diseases (3, 10). In this context, especially sigma-1 receptors have an important role in modul-ating several physiological event such as the regulation of inositol 1,4,5-triphosphate receptors and calcium mobilization from endoplasmic reticulum stores, modulation of calcium entry through ion channels at the plasma membrane including Kv1.4 channels, N-methyl-d-aspartate (NMDA) receptors, and voltage-dependent calcium channels. Simi-larly, in in-vitro and in-vivo studies, sigma-1 receptor activation inhibited ischemic injury by decreasing NO production via suppressing such as decrease glutamate and NMDA-induced nitric oxide synthase (NOS) activation. Therefore, the sigma-1 receptor could be a novel target in treatment of many diseases such as stroke (6, 11–14). For example, it has been shown that activation of sigma-1 receptor agonists has protective effect in focal cerebral ischemia injury by suppression neuronal NO production (11). Also, Katnik et al. (3) have shown that regulating intracellular calcium by activation of sigma receptors in cortical neurons exposed to ischemia is one of the mechanisms by which these receptors may possibly exert their neuroprotective properties in this condition. They also demonstrated that the sigma-1 receptor antagonist, BD1047 blocked this neuroprotective effect.
Noscapine is a benzylisoquinoline alkaloid from the opium poppy Papaver somniferum and unlike many other alkaloids from the opium latex does not have any notable secondary sedative, hypnotic, analgesic, or euphoric properties (15–17). Ye et al. (18) showed that noscapine could inhibit murine lymphoid tumors, probably by suppressing microtubules assembly. Also, this agent could cause cell cycle arrest in several human and murine neoplasms including lymphoma, thymoma, melanoma, and breast cancer and induced apoptosis in both in-vitro and in-vivo study (15, 19). Kamei (20) showed that pretreatment with rimcazole, a specific antagonist of sigma-receptors decreased antitussive effects of noscapine considerably. Ebrahimi (21) showed that the effect of noscapine on cough suppression was probably via interference with the actions of bradykinin. In addition, Mahmoudian et al. (22) demonstrated that noscapine decreased inflammatory damage during ischemia in rat pups model of ischemic brain injury. Khanmoradi et al. (23) showed that Noscapine protected renal tissue in Wistar rats against ischemia-reperfusion injury by down regulation of the inflammatory mediators. Another study in our lab demonstrated that noscapine significantly increased neuronal cell viability after oxygen-glucose deprivation for 30, 60, and 240 min in murine primary cortical culture probably by re-establishment of calcium homeostasis following a decrease in NO production (24).
In this work, we aim to determine whether sigma receptors are involved in protective effects of noscapine after oxygen–glucose deprivation/recovery (OGD/R). In addition, we want to show if the protective effects of noscapine are dependent on modulation of intracellular calcium and/or NO production.
Experimental
Preparation of primary cell cultures
Timed pregnant mouse was purchased from Iran University of medical science. Under anaesthesia using chloroform, the pregnant mouse was killed by cervical dislocation and mouse embryos (E15–18) were euthanized and decapitated. Fetal cerebral cortex was used for primary mice cortical neuron isolation and cultured as described previously (25, 26).
The resulting homogenate was then centrifuged at 300 ×g for 5 min at 20 °C and were seeded in 12-well plates (at a density of 6 × 104) and glass coverslips pre-treated with poly-D-lysine (10 µg/mL; Sigma–Aldrich) for viability evaluation via OGD and chemical OGD, respectively. The cultures were maintained at 37 °C in 100% humidity and in a 95% air and 5% CO2 atmosphere. The plating medium consisted of RPMI-1640 supplemented with 10% FBS, 5% horse serum, and 100 IU/mL penicillin and100 µg/ml streptomycin (Sigma-Aldrich, Saint Louis, MS, USA).
After 24–48 h, to eliminate the very small number of non-neuronal cells, 10 µM cytosine arabinoside (Sigma, St. Louis, MO, USA) was added to medium culture for 3 days. The experiments were performed after 11-16 days in-vitro. All experiments were carried out by the Institute of Animal Care Committee at Iran University of Medical Sciences.
Oxygen–glucose deprivation and drug exposure
After 11-16 days in culture, noscapine was exposed to Oxygen–glucose deprivation as described previously (27).
The cells were exposed with 5 µM BD1047, the selective sigma-1 antagonist (Sigma-Aldrich, St. Louis, MO, USA) with or without 0.5-2 µM noscapine (Sigma-Aldrich, St. Louis, MO, USA), 24 h before OGD. The current concentration of noscapine was chosen according to our previous research works.
Briefly, to achieve OGD, the cells were treated to OGD medium containing glucose/glutamine-free DMEM without FBS and transferred in an anaerobic chamber with 95% (v/v) N2 and 5% (v/v) CO2. The anaerobic chamber was kept sealed at 37 °C. After 60 min in OGD, the chamber was opened and the cultures were removed from the anaerobic chamber and returned to a normoxic environment in RPMI-1640 under the standard cell incubator containing 5% CO2, 95% air balance, and 98% humidity at 37 °C for 24 h.
Analysis of neuronal cell viability
For quantitative measurement of neuronal viability, 3-(4, 5-dimethylthiazol-2-yl)-2, 5-diphenyltetrazolium bromide (MTT) assay was added at a final concentration of 0.5 mg/mL for 4 h as previously described by (28).
After the cell incubation with MTT, the insoluble formazan crystals in the living cells was dissolved in 200 μL DMSO for 15 min. The optical density (OD) was measured at 570 nm with Dynex MMX microplate reader (Dynex, Richfield, MN, USA). Survival of the control group was defined as 100%. OGD control group: the plates exposed to OGD conditions without addition of any drugs. Control group: the plates not exposed to OGD and without addition any drugs. BD1047 group: the plates exposed to OGD conditions with addition of 5 µM BD1047 (the selective antagonist of sigma-one receptors). BD1047 and noscapine group: the plates exposed to OGD conditions with addition of 5 µM BD1047 and different concentrations of noscapine (0.5-2 µM).
Nitrite analysis
Detecting NO2– in culture supernatants was assessed by using modified Griess reaction (Sigma Chemical Co. St. Louis, MO, USA). The Griess reagent system is based on the chemical reaction which reacts with sulfanilic acid to produce the diazonium ion. A Nitrite Standard reference curve must be prepared for each assay for accurate quantitation of NO2– levels in experimental samples.
After 24 h OGD, the medium in each well was removed and centrifuged at 10,000 ×g for 10 min at 20 °C. Nitrite was determined by mixing 100 µL supernatant with an equal volume of Griess reagent at room temperature. After 10 min, the absorbance at 540 nm was determined on a microplate reader.
Measurement of intracellular free calcium
To describe the intracellular calcium levels during ischemia, 4 mM NaN3 (Sigma-Aldrich, Saint Louis, MS, USA), was used for induction of chemical OGD in the presence of noscapine with or without BD 1047 and continued throughout the entire experiment.
After 30 min chemical OGD, cortical cell cultures grown on coverslips were washed three times with salt solution containing (in mM): 140 NaCl, 3 KCl, 2.5 CaCl2, 1.2 MgCl2, 7.7 glucose and 10 HEPES, pH 7.4 and incubated with 4 µM fura 2/AM at room temperature for 30 min in a dark incubator.
We used an Olympus IX-71 inverted microscope and a cooled charge-couple device (CCD) camera equipped with an automated fluorescence filter changer for recording and subsequent [Ca2+]i calculation. Fluorescence emission images of intracellular Fura-2 at 510 nm and change its peak excitation from 340 nm to 380 nm in response to calcium binding. Ratio metric analyzes were carried out by using ImageJ software.
Statistical analysis
All values were expressed as the mean ± standard error mean (SEM). statistical analysis of triplicate measurements represented at least three independent experiments to obtain comparable results for statistical analysis. Significant differences among multiple groups were examined using One-way analysis of variance (ANOVA) followed with Dunnett test. Statistical significance was defined as P-values less than 0.05 (P < 0.05). the data were analyzed using Graph Pad Prism 6.0 software.
Results
Involvement of sigma-1 receptors in pro-tection after OGD/R
To clarify whether the protective effect of noscapine against 60 min OGD/R-induced cell death was associated with sigma receptors, we examined the effects BD1047, the sigma-1 selective antagonist in the presence or absence of different concentrations of noscapine. Cell survival following OGD-induced cell damage in the presence of BD1047 and different concentrations of noscapine was determined.
As shown in Figure 1 the decrease in cell viability induced by 5 µM BD1047 (27.05% ± 0.32) was statistically significant when compared to the OGD control group (61.92% ± 1.2).
Our results showed that pretreatment of neuronal cells with BD1047 followed by noscapine (0.5-2 µmol) after 60 min oxygen–glucose deprivation/24 h recovery decreased cell viability compared with OGD control group.
However, although noscapine at its highest concentration was unable to exert a complete protective effect (compared to OGD/R control), it did have a concentration dependent protective effect.
Involvement of sigma-1 receptors in changing NO production after OGD/R
We examined the effects of 5 µM BD1047 on NO production in primary cortical neurons exposed to 30 min OGD/R in the presence of different concentrations of noscapine. As shown in Figure 2, the pre-treatment of neuronal cells with BD1047 (1.70 ± 0.03) after 30 min OGD/R, produced significantly more nitrite compared to OGD control group (1.11 ± 0.04). Pre-treating neurons with BD1047 and noscapine (0.5-2 µM) together after 30 min OGD/R induced a decrease in NO production as compared with BD1047 alone. The effects of noscapine in the presence of BD1047 on NO production were concentration dependent; however even at the highest noscapine concentration the nitrite levels did not return to OGD/R control group levels.
The effects of L-NAME (100 µM) on NO production were also examined. The results indicated that NO production induced by 30 min of oxygen– glucose deprivation was reduced by L-NAME, a NOS inhibitor (Figure 2).
Involvement of sigma-1 receptors in modulating intracellular calcium levels after OGD/R
Four millimolar NaN3 was able to induce a significant increase in [Ca2+]i levels in cortical neurons bathed in Ca2+-containing medium (146.49% ± 1.75) (Figures 3A-3B). Exposure of cortical neurons to NaN3 and 2 µM noscapine after 30 min, decreased [Ca2+]i levels by 127.73% ± 1.75. However, the chemical OGD-evoked increase in [Ca2+]i levels was by 204.98 ± 2.04 in the presence of 5 µM BD1047. Nevertheless, when 2 µM noscapine was used in the presence of 5 µM BD1047 after 30 min, the level of intracellular of calcium declined (185.15% ± 31.48) (Figures 4A-4B).
Discussion
Research by other workers has demonstrated that glutamate is a key neurotransmitter in the ischemia induced excitotoxicity process. An excess of glutamate is thought to play an important role in the progress of cerebral infarction via NMDA receptor activation that leads to intracellular calcium overload which causes in turn toxic reaction and ultimately leads to cell damage and death (29–31). Although blocking these receptors could help to attenuate neuronal damage, inhibition of other positive effects of NMDA receptors put a limit on usefulness of this treatment. Therefore, other strategies had to be used to treat hypoxic-ischemic brain damage for moderating NMDA receptor function or interaction in the cascade of toxic reactions that led to excitotoxicity.
According to the previous investigations, sigma receptors could act as putative therapeutic targets for reduction of excitotoxic and neurodegenerative damage (29, 32). Sigma receptors are important because they can greatly affect function of glutamate receptors during excitotoxicity (33). Ligands of both types of sigma receptor subtypes possibly synergistic influenced by several aspects of neurodegeneration and made it possible to modulator effect on neuronal degeneration process far more than traditional drugs (29). More recently a greater role has been attributed to the sigma-1 receptor subtype in some pathological and physiological processes (2). Sigma-1 receptors are located mostly on the endoplasmic reticulum (ER), but when cells are exposed to prolonged stress or their sigma- one agonists, these receptors could translocate to the plasma membrane and modulate different ion channels activities such as voltage-gated and ligand-gated Ca2+, K+, Na+ and Cl- (34). Therefore, based on these observations it has been suggested that sigma-1 receptor ligands hold promise as protective agents for the treatment of cerebral ischemia (34–36).
Many studies have shown that sigma receptor ligands have a wide variety of actions including protecting of neuronal cells and treatment of cerebral ischemia in several cells such as retinal ganglion cells, cerebral cortex, primary neuronal cultures, and cells used in ischemic stroke models (29, 33–35 and 37). For example, it has been revealed that the potent sigma-1 receptor ligand 4-phenyl-1-(4-phenylbutyl) piperidine (PPBP) attenuated neuronal injury of primary cortical neuronal cultures when exposed to 120 min OGD or 100 µM glutamate (37). Yang et al. (35) have demonstrated that therapeutic use of sigma-1 receptor ligand PPBP protected striatal neurons in newborn piglets model of neonatal global hypoxia-ischemia devoid of showing adverse effects associated with completely blocking NMDA receptors in the developing brain. Similarly, PPBP used in an in-vitro model of hypoxia/hypoglycemia in rat primary neuronal cultures and could decrease neurotoxicity caused by cerebral ischemia (32). However, the exact mechanism of this effect is unclear.
In previous studies it has been shown that noscapine could induce the protective effects in variety of ischemic conditions. Khanmoradi et al. (23) showed that noscapine exerted renoprotective effects via down regulation of the inflammatory mediators such as tumor necrosis factor-α (TNF-α) and monocyte chemoattractant protein1 (MCP-1) in renal ischemia–recovery injury in rat models. Mahmoudian et al. (22) demonstrated that noscapine as a bradykinin antagonist reduced inflammation in a rat model of prenatal hypoxic-ischemic brain edema. Vahabzadeh et al. (24) showed that noscapine decreased NO levels and modulation intracellular calcium levels in primary murine fetal cortical. Kamei et al. (38) showed that the antitussive effect of noscapine was dose dependently reduced by pre-treatment with rimcazole, a specific antagonist of sigma sites in mice. This is appearing that noscapine has a protective role in ischemic conditions. Therefore, it could be postulated that this protective effect afforded by noscapine were to some extent dependent upon its action on sigma-one receptors.
As such, the aim of this research is to investigate the effects of noscapine in the presence of 5 µM BD1047, a sigma-1 receptor antagonist in primary cortical culture on 60 min oxygen-glucose deprivation/24 h recovery and show that if different concentrations of noscapine could protect neuronal cells against sigma-1 antagonist in this condition and which mechanisms were involved.
Lack of oxygen and/or glucose is the main reason for ischemic brain injury (39–41). Thus, models have been developed to simulate, in-vitro conditions of low or no oxygen/glucose to mimic the pathologic process of ischemic stroke (42,43).
Goldberg and Choi (44) subjected primary neuronal cultures to no oxygen/ no glucose conditions followed by normal oxygen and glucose exposure. He provided evidence that this model in-vitro produced cell damage similar to neurodegeneration that occurs in cerebral ischemic events in man. Therefore, the Goldberg OGD/R model was adopted in the present work.
MTT assay is widely used to measure cell viability and is a good indicator of cellular metabolic activity (45). In this experiment we used MTT assay to measure the number of living cells and were able to show that 5 µM BD1047 did not have neuroprotective effects on primary cortical culture after 60 min OGD, when used alone. However, when cells incubated in 0.5-2 µM noscapine together with 5 µM BD1047 after 60 min OGD/R, the neuroprotective effects of noscapine were increased in a dose-dependent manner compared to BD1047alone but this effect was not complete.
It can be concluded that the neuroprotective effect of noscapine was at least, partially reversed by pretreating cells with BD1047, a selective sigma-1 antagonist. This suggests that activation of sigma-1 receptors is probably involved in the neuroprotective effects of noscapine against OGD-induced cell injury.
During the period of oxygen and/or glucose deprivation, or in the process of nerve damage, amounts of cellular and neuronal energy is diminished; this change results in increase in glutamate and aspartate concentration. Thus, NMDA receptor stimulation, followed by intracellular Ca2+ change and Ca2+-CaM pathway activation ensue. It is known that excessive calcium in neuron and endothelial cells can activate the Ca2+-dependent enzyme cascades such as neuronal and endothelial isoforms of NOS (nNOS and eNOS). Therefore, increased intracellular NO levels after OGD/R, is expected. (30, 46 and 47). In the target cells, NO is converted to the cytotoxic molecule peroxynitrite which causes damage to DNA and therefore leads to cell injury or death (30, 46). In several in-vitro and in-vivo animal ischemic models, neurotoxicity of NO has been demonstrated. It is also known that under certain pathological conditions, NO production increases e.g. during ischemia. This can lead to cell injury and apoptotic cells death by induction of glutamate (11, 35, 46 and 47).
In this work, we were able to show that OGD/R caused an increase in the amount of intracellular NO. Also, our data show that BD1047 enhanced the NO generation due to OGD/R treat. In addition, noscapine was able to partially restore NO levels in the presence of BD1047.
Many studies have demonstrated that in the presence of ischemia, the generation of NO increases (11, 30, 35, 41 and 48). For example, Goyagi et al. (11) showed that PPBP, the potent sigma-1 agonist, had neuroprotective effects and decreased NO production after in-vivo striatal tissue damage due to 90 min middle cerebral artery occlusion (MCAO) in Wistar rats. Also, these effects of PPBP disappeared when nNOS was absent or was inhibited. In another research, Yang et al. (35) demonstrated that in striatal neurons of newborn piglets exposed to global hypoxia-ischemia, PPBP protected neurons by inhibiting NOS activity that was linked to the decreased coupling of nNOS to postsynaptic density-95 (PSD-95).
Therefore, the data obtained in this work is consistent with other investigations in so far as it shows the importance of sigma-one receptors in lowering the NO induced by OGD/R treatment. In addition, we were able to show that NO-attenuating effects of noscapine were also dependent, at least partially, on sigma-one receptor function.
Change in intracellular calcium ion concentration induced by ischemic condition, result to cell death. Increasing intracellular calcium via stimulation of calcium-sensitive ion channels led to disestablishment of plasma membrane function and finally boosted processes such as proteolysis, lipolysis, and the production of reactive oxygen species that caused cell injury and death (3). In this regard, one important mechanism by which sigma-1 receptor ligands protected the neuronal cells in glutamate receptor-mediated excitotoxicity, was modulating intracellular calcium homeostasis and inhibiting ion channel function (2, 3 and 9). For example; it has been shown that in neurocortical culture which was exposed to ischemia, activation of sigma-1 receptors protects the neuronal cells by decreasing in intracellular calcium concentrations (3).
Sodium azide/glucose deprivation model (chemical OGD) has been demonstrated to provoke electrophysiological and neurochemical changes similar to what is observed in the OGD model and could simulate ischemic conditions. This model is preferable to OGD because of producing neurochemical changes more rapidly and reproducibly, therefore making it easier to record the events that are related to changes in [Ca2+]i (49). Thus, in this study, sodium azide/glucose deprivation model was used. Our results showed that at the time of chemical OGD, the [Ca2+]i was significantly increased compared to the external control condition. This result is consistent with previous reports, which showed that sodium azide could increase neuronal [Ca2+]i, to reduced energy production (3, 49). So, we examined the [Ca2+]i level in neuronal cells exposed to 2 µM noscapine after 30 min of sodium azide and glucose deprivation treatment by using the Ca+2-indicator Fura-2. The results suggested that exposure to 2 µM noscapine was able to reverse the effects of chemical OGD on intracellular Ca2+ levels in primary cortical culture. This study also showed that increase in intracellular calcium by 5 µM BD1047, sigma-1 receptor antagonist, was diminished by noscapine, suggesting that noscapine acted as a sigma-1 receptor agonist. Our results support previous observations in other tissues that sigma-1 receptor ligands could modify intracellular calcium ion concentration. Katnik et al. (3) demonstrated that 1,3-di-o-tolyl- guanidine (DTG), a sigma receptor agonist, was able to decrease [Ca2+]i elevations in cultured cortical neurons from the embryonic rats exposed to chemical OGD. Also when the cells were treated with BD1047, DTG was not able to prevent ischemia induced increases in [Ca2+]i. Mueller et al. (34) showed that sigma-1 receptors exert neuroprotective effects on retinal ganglionic cells by suppression of calcium signaling through L-type VGCCs. Hayashi et al. (50) also showed that in a neuroblastoma-glioma cell line (NG108), sigma-1 receptors modulated calcium signaling. Our data in the line with these works, demonstrate that noscapine has a protective effect on primary cortical culture exposed to sodium azide and glucose deprivation via reduction of intracellular Ca2+ levels thorough sigma-1 receptors. Thus, the role of sigma-1 receptor activation by noscapine was confirmed from the antagonism observed by BD1047.
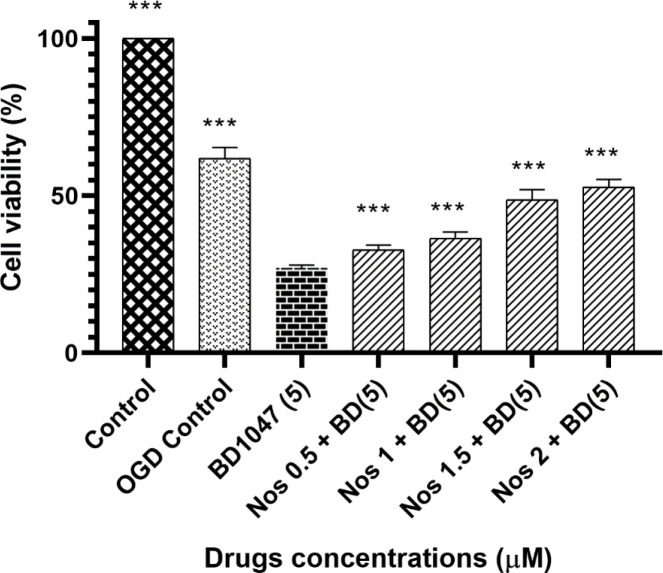
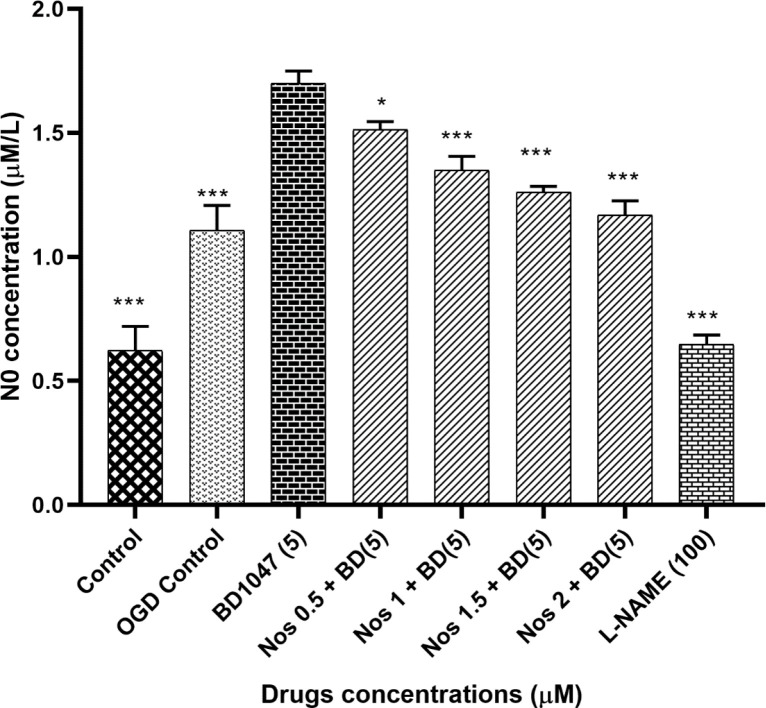
![Changes in [Ca2+]i levels induced by chemical OGD (4 mM NaN3) in the presence of 2 µM noscapine in primary cortical neurons. (A) Time course of NaN3-induced [Ca2+]i rise in presence of 2 µM noscapine. NaN3 (4 mM) stimulated [Ca2+]i levels increase. After 30 min ischemic insult, the cells were treated with 2 µM noscapine during chemical ischemia. Two micromolar noscapine could decrease [Ca2+]i levels during chemical ischemia. (B) Bar graph of mean change in [Ca2+]i levels acquired in response to chemical ischemia in presence of 2 µM noscapine. ***P < 0.001 vs. NaN3 group](https://services.brieflands.com/cdn/serve//315db/ca41b59d4c4ba9720a0018544bd2432afa761585/ijpr-19-331-g003.jpg)
![Changes in [Ca2+]i levels induced by chemical OGD (4 mM NaN3) in the presence of BD1047 with or without 2 µM noscapine in primary cortical neurons. (A) Time course of NaN3-induced [Ca2+]i rise in presence and absence of 2 µM noscapine and 5 µM BD1047. NaN3 (4 mM) stimulated [Ca2+]i levels increase. After 30 min ischemic insult, the cells treated with 5 µM BD1047. BD1047 could rise [Ca2+]i levels during chemical ischemia. Two micromolar noscapine added to 5 µM BD1047 during ischemia and reduced the [Ca2+]i levels compare to BD1047 alone. (B) Bar graph of mean change in [Ca2+]i levels acquired in response to chemical ischemia in presence and absence of 2 µM noscapine and 5 µM BD1047. ***P < 0.001 vs. NaN3 group](https://services.brieflands.com/cdn/serve//315db/061d6f999ea49bb0f5eeea6c21a6ea4bf0fc1693/ijpr-19-331-g004.jpg)
Conclusion
In conclusion, our data suggests that at least some of the neuroprotective effects of noscapine are due to its agonistic action on the sigma-1 receptor and that neuroprotective effects of noscapine against ischemia involve sigma-1 receptor activations induced suppression of intracellular NO levels and calcium.
Acknowledgements
References
-
1.
Maya S, Prakash T, Das K. Assessment of neuroprotective effects of Gallic acid against glutamate-induced neurotoxicity in primary rat cortex neuronal culture. Neurochem. Int. 2018;121:50-8. [PubMed ID: 30339985].
-
2.
Nguyen L, Lucke-Wold BP, Mookerjee SA, Cavendish JZ, Robson MJ, Scandinaro AL, Matsumoto RR. Role of sigma-1 receptors in neurodegenerative diseases. J. Pharmacol. Sci. 2015;127:17-29. [PubMed ID: 25704014].
-
3.
Katnik C, Guerrero WR, Pennypacker KR, Herrera Y, Cuevas J. Sigma-1 receptor activation prevents intracellular calcium dysregulation in cortical neurons during in-vitro ischemia. J. Pharmacol. Exp. Ther. 2006;319:1355-65. [PubMed ID: 16988055].
-
4.
Hazell AS. Excitotoxic mechanisms in stroke: an update of concepts and treatment strategies. Neurochem. Int. 2007;50:941-53. [PubMed ID: 17576023].
-
5.
Carnally SM, Johannessen M, Henderson RM, Jackson MB, Edwardson JM. Demonstration of a direct interaction between sigma-1 receptors and acid-sensing ion channels. Biophys. J. 2010;98:1182-91. [PubMed ID: 20371317].
-
6.
Martina M, Turcotte MEB, Halman S, Bergeron R. The sigma-1 receptor modulates NMDA receptor synaptic transmission and plasticity via SK channels in rat hippocampus. J. Physiol. 2007;578:143-57. [PubMed ID: 17068104].
-
7.
van Waarde A, Rybczynska AA, Ramakrishnan NK, Ishiwata K, Elsinga PH, Dierckx RAJO. Potential applications for sigma receptor ligands in cancer diagnosis and therapy. Biochim. Biophys. Acta. 2015;1848:2703-14. [PubMed ID: 25173780].
-
8.
Kume T, Nishikawa H, Taguchi R, Hashino A, Katsuki H, Kaneko S, Minami M, Satoh M, Akaike A. Antagonism of NMDA receptors by σ receptor ligands attenuates chemical ischemia-induced neuronal death in-vitro. Eur. J. Pharmacol. 2002;455:91-100. [PubMed ID: 12445574].
-
9.
Katnik C, Garcia A, Behensky AA, Yasny IE, Shuster AM, Seredenin SB, Petrov AV, Cuevas J. Activation of sigma1 and sigma2 receptors by afobazole increases glial cell survival and prevents glial cell activation and nitrosative stress after ischemic stroke. J. Neurochem. 2016;139:497-509. [PubMed ID: 27488244].
-
10.
Maurice T, Su TP. The pharmacology of sigma-1 receptors. Pharmacol. Ther. 2009;124:195-206. [PubMed ID: 19619582].
-
11.
Goyagi T, Goto S, Bhardwaj A, Dawson VL, Hurn PD, Kirsch JR. Neuroprotective effect of σ1-receptor ligand 4-phenyl-1-(4-phenylbutyl) piperidine (PPBP) is linked to reduced neuronal nitric oxide production. Stroke. 2001;32:1613-20. [PubMed ID: 11441209].
-
12.
Guzman-Lenis MS, Navarro X, Casas C. Selective sigma receptor agonist 2-(4-morpholinethyl)1-phenylcyclohexanecarboxylate (PRE084) promotes neuroprotection and neurite elongation through protein kinase C (PKC) signaling on motoneurons. Neuroscience. 2009;162:31-8. [PubMed ID: 19345724].
-
13.
Tchedre KT, Huang RQ, Dibas A, Krishnamoorthy RR, Dillon GH, Yorio T. Sigma-1 receptor regulation of voltage-gated calcium channels involves a direct interaction. Invest. Ophthalmol. Vis. Sci. 2008;49:4993-5002. [PubMed ID: 18641291].
-
14.
Vagnerova K, Hurn PD, Bhardwaj A, Kirsch JR. Sigma-1 receptor agonists act as neuroprotective drugs through inhibition of inducible nitric oxide synthase. Anesth. Analg. 2006;103:430-4. [PubMed ID: 16861428].
-
15.
Sung B, Ahn KS, Aggarwal BB. Noscapine, a benzylisoquinoline alkaloid, sensitizes leukemic cells to chemotherapeutic agents and cytokines by modulating the NF-κB signaling pathway. Cancer Res. 2010;70:3259-68. [PubMed ID: 20354190].
-
16.
Mahmoudian M, Mehrpour M, Benaissa F, Siadatpour Z. A preliminary report on the application of noscapine in the treatment of stroke. Eur. J. Clin. Pharmacol. 2003;59:579-81. [PubMed ID: 14517705].
-
17.
Altinoz MA, Topcu G, Hacimuftuoglu A, Ozpinar A, Hacker E, Elmaci İ. Noscapine, a non-addictive opioid and microtubule-inhibitor in potential treatment of glioblastoma. Neurochem. Res. 2019;44:1796-106. [PubMed ID: 31292803].
-
18.
Ke Y, Ye K, Grossniklaus HE, Archer DR, Joshi HC, Kapp JA. Noscapine inhibits tumor growth with little toxicity to normal tissues or inhibition of immune responses. Cancer Immunol. Immunother. 2000;49:217-25. [PubMed ID: 10941904].
-
19.
Barken I, Geller J, Rogosnitzky M. Noscapine inhibits human prostate cancer progression and metastasis in a mouse model. Anticancer Res. 2008;28:3701-4. [PubMed ID: 19189652].
-
20.
Kamei J. Possible role of sigma-receptors in the regulation of cough reflex, gastrointestinal and retinal function. Nihon Yakurigaku Zasshi. 1999;114:35-41. [PubMed ID: 10562963].
-
21.
Ebrahimi SA, Zareie MR, Rostami P, Mahmoudian M. Interaction of noscapine with the bradykinin mediation of the cough response. Acta Physiol. Hung. 2003;90:147-55. [PubMed ID: 12903913].
-
22.
Mahmoudian M, Siadatpour Z, Ziai SA, Mehrpour M, Benaissa F, Nobakht M. Reduction of the prenatal hypoxic-ischemic brain edema with noscapine. Acta Physiol. Hung. 2003;90:313-8. [PubMed ID: 14708873].
-
23.
Khanmoradi M, Mard SA, Aboutaleb N, Nobakht M, Mahmoudian M. The protective activity of noscapine on renal ischemia–reperfusion injury in male Wistar rat. Iran. J. Basic Med. Sci. 2014;17:244-9. [PubMed ID: 24904716].
-
24.
Vahabzadeh G, Rahbar-Roshandel N, Ebrahimi SA, Mahmoudian M. Neuroprotective effect of noscapine on cerebral oxygen–glucose deprivation injury. Pharmacol. Rep. 2015;67:281-8. [PubMed ID: 25712651].
-
25.
Dawson VL, Dawson TM, London ED, Bredt DS, Snyder SH. Nitric oxide mediates glutamate neurotoxicity in primary cortical cultures. Proc. Natl. Acad. Sci. U. S. A. [PubMed ID: 1648740].
-
26.
Dawson VL, Dawson TM, Bartley DA, Uhl GR, Snyder SH. Mechanisms of nitric oxide-mediated neurotoxicity in primary brain cultures. J. Neurosci. 1993;13:2651-61. [PubMed ID: 7684776].
-
27.
Frantseva MV, Carlen PL, El-Beheiry H. A submersion method to induce hypoxic damage in organotypic hippocampal cultures. J. Neurosci. Methods. 1999;89:25-31. [PubMed ID: 10476680].
-
28.
Fan W, Li X, Huang L, He S, Xie Z, Fu Y, Fang W, Li Y. S-oxiracetam ameliorates ischemic stroke induced neuronal apoptosis through up-regulating α7 nAChR and PI3K/Akt/GSK3β signal pathway in rats. Neurochem. Int. 2018;115:50-60. [PubMed ID: 29355569].
-
29.
Nguyen L, Kaushal N, Robson MJ, Matsumoto RR. Sigma receptors as potential therapeutic targets for neuroprotection. Eur. J. Pharmacol. 2014;743:42-7. [PubMed ID: 25261035].
-
30.
Kostandy BB. The role of glutamate in neuronal ischemic injury: the role of spark in fire. Neurol. Sci. 2012;33:223-37. [PubMed ID: 22044990].
-
31.
Allahtavakoli M, Shabanzadeh A, Roohbakhsh A, Pourshanazari A. Combination therapy of rosiglitazone, a peroxisome proliferator-activated receptor-γ ligand and NMDA receptor antagonist (MK-801) on experimental embolic stroke in rats. Basic Clin. Pharmacol. Toxicol. 2007;101:309-14. [PubMed ID: 17910613].
-
32.
Wegleiter K, Hermann M, Posod A, Wechselberger K, Stanika RI, Obermair GJ, Kiechl-Kohlendorfer U, Urbanek M, Griesmaier E. The sigma-1 receptor agonist 4-phenyl-1-(4-phenylbutyl) piperidine (PPBP) protects against newborn excitotoxic brain injury by stabilizing the mitochondrial membrane potential in-vitro and inhibiting microglial activation in-vivo. Exp. Neurol. 2014;261:501-9. [PubMed ID: 25111531].
-
33.
Shimazu S, Katsuki H, Takenaka C, Tomita M, Kume T, Kaneko S, Akaike A. σ Receptor ligands attenuate N-methyl-D-aspartatecytotoxicity in dopaminergic neurons of mesencephalic slice cultures. Eur. J. Pharmacol. 2000;388:139-46. [PubMed ID: 10666505].
-
34.
Mueller 2nd BH, Park Y, Daudt 3rd DR, Ma HY, Akopova I, Stankowska DL, Clark AF, Yorio T. Sigma-1 receptor stimulation attenuates calcium influx through activated L-type voltage gated calcium channels in purified retinal ganglion cells. Exp. Eye Res. 2013;107:21-31. [PubMed ID: 23183135].
-
35.
Yang ZJ, Carter EL, Torbey MT, Martin LJ, Koehler RC. Sigma receptor ligand 4-phenyl-1-(4-phenylbutyl)-piperidine modulates neuronal nitric oxide synthase/postsynaptic density-95 coupling mechanisms and protects against neonatal ischemic degeneration of striatal neurons. Exp. Neurol. 2010;221:166-74. [PubMed ID: 19883643].
-
36.
Harukuni I, Bhardwaj A. Mechanisms of brain injury after global cerebral ischemia. Neurol. Clin. 2006;24:1-21. [PubMed ID: 16443127].
-
37.
Yang S, Bhardwaj A, Cheng J, Alkayed NJ, Hurn PD, Kirsch JR. Sigma receptor agonists provide neuroprotection in-vitro by preserving bcl-2. Anesth. Analg. 2007;104:1179-84. [PubMed ID: 17456670].
-
38.
Kamei J, Iwamoto Y, Misawa M, Kasuya Y. Effects of rimcazole, a specific antagonist of σ sites, on the antitussive effects of non-narcotic antitussive drugs. Eur. J. Pharmacol. 1993;242:209-11. [PubMed ID: 8253118].
-
39.
Wang C, Nguyen HN, Maguire JL, Perry DC. Role of intracellular calcium stores in cell death from oxygen-glucose deprivation in a neuronal cell line. J. Cereb. Blood Flow Metab. 2002;22:206-14. [PubMed ID: 11823718].
-
40.
Bacigaluppi S, Donzelli E, De Cristofaro V, Bragazzi NL, D’Amico G, Scuteri A, Tredici G. Human endothelial progenitor cells rescue cortical neurons from oxygen-glucose deprivation induced death. Neurosci. Lett. 2016;631:50-55. [PubMed ID: 27521752].
-
41.
Tavakoli-Far B, Rahbar-Roshandel N, Rahimi-Moghaddam P, Mahmoudian M. Neuroprotective effects of mebudipine and dibudipine on cerebral oxygen–glucose deprivation/reperfusion injury. Eur. J. Pharmacol. 2009;610:12-7. [PubMed ID: 19285496].
-
42.
Zeng X, Ren H, Zhu Y, Zhang R, Xue X, Tao T, Xi H. Gp91phox (NOX2) in activated microglia exacerbates neuronal damage induced by oxygen glucose deprivation and hyperglycemia in an in-vitro model. Cell. Physiol. Biochem. 2018;50:783-97. [PubMed ID: 30317245].
-
43.
Rahbar-Roshandel N, Razavi L, Tavakoli-Far B, Mahmoudian M. Mebudipine and dibudipine protect PC12 cells against oxygen–glucose deprivation and glutamate-induced cell death. Pathophysiology. 2008;15:227-31. [PubMed ID: 18945602].
-
44.
Goldberg MP, Choi DW. Combined oxygen and glucose deprivation in cortical cell culture: calcium-dependent and calcium-independent mechanisms of neuronal injury. J. Neurosci. 1993;13:3510-24. [PubMed ID: 8101871].
-
45.
Huang L, Li Q, Li H, He Z, Cheng Z, Chen J, Guo L. Inhibition of intracellular Ca2+ release by a Rho-kinase inhibitor for the treatment of ischemic damage in primary cultured rat hippocampal neurons. Eur. J. Pharmacol. 2009;602:238-44. [PubMed ID: 19070614].
-
46.
Kolesarova M, Pavel J, Lukacova N, Kolesar D, Marsala J. Effect of ischemia in-vivo and oxygen-glucose deprivation in-vitro on NOS pools in the spinal cord: comparative study. Cell. Mol. Neurobiol. 2006;26:1281-94. [PubMed ID: 16691443].
-
47.
Zhang M, Ning GM, Hong DH, Yang Y, Kutor J, Zheng XX. The Influence of oxygen-glucose deprivation on nitric oxide and intracellular ca2+ in cultured hippocampal neurons. Acta Biochim. Biophys. Sin. 2003;35:561-6. [PubMed ID: 12796818].
-
48.
Babahajian A, Sarveazad A, Golab F, Vahabzadeh G, Alizadeh A, Rasoolijazi H, Amini N, Entezari M, Soleimani M, Katebi M, Haramshahi SMA. Neuroprotective effects of trolox, human chorionic gonadotropin and carnosic acid on hippocampal neurodegeneration after ischemiareperfusion injury. Curr. Stem. Cell Res. Ther. 2019;14:177-83.
-
49.
Vahabzadeh G, Ebrahimi SA, Rahbar-Roshandel N, Mahmoudian M. The effect of noscapine on oxygen-glucose deprivation on primary murine cortical neurons in high glucose condition. Iran. J. Pharm. Res. 2016;15:501-12.
-
50.
Hayashi T, Maurice T, Su TP. Ca2+ Signaling via ς1-Receptors: novel regulatory mechanism affecting intracellular Ca2+ concentration. J. Pharmacol. Exp. Ther. 2000;293:788-98. [PubMed ID: 10869377].