Abstract
Keywords
Addiction Morphine Infertility pathway Reproductive hormone Testis
Introduction
Addiction to mind-altering and recreational drugs is increasingly becoming a major medical and social problem worldwide, being prevalent in rich and developing countries similarly (1). A considerable number of cases of “male factor” infertility are categorized as “idiopathic” owing to their unknown etiology (2). Infertility and problems of impaired fecundity have been a concern for ages and are also a significant clinical problem today, affecting 8–12% of couples worldwide. Approximately 40–50% of all infertility cases are due to “male factor” infertility (3).
Endogenous opioid peptides are present in different tissues of the male reproductive tract, suggesting that they may be involved in reproductive function (4). The effect of opioids on producing testosterone by inhibiting the Gonadotropin-releasing hormone (GnRH) secretion, injections of β-endorphin in the ventromedial, anterior and preoptic-septal hypothalamic areas reduce the luteinizing hormone (LH) secretion from the pituitary. To establish down-regulation of GnRH mRNA levels through morphine, an in situ hybridization was used by Li and Pelletier (5). Thus, the administration of chronic morphine inhibits the secretion of GnRH (6). On the other hand, levels of follicle-stimulating hormone (FSH) are not influenced by opioid analogs or antagonists (6). Moreover, as animal models have shown, modulation of the negative feedback of sex steroids on secreting LH can be by opioids (6). In male rats, the hypothalamus sensitivity to negative feedback is enhanced by testosterone morphine (7, 8).
According to previous studies, morphine alone does a great deal of damage to testicular tissue (9). Salahshoor et al. demonstrated that addiction in the male rat adversely affected fertility (10). Fronczak et al. reviewed the literature on the prevalence and effects of drug abuse on male fertility (11). Studies have shown that morphine induces oxidative stress in animals by producing free radicals and ROS, thereby affecting glycolysis, the mitochondrial respiratory chain, ATP production, amino acid metabolism, the antioxidant defense system, cellular detoxification, and gene toxicity (12).
There is a possible association between the increased side effect of addiction and gene expression changes; therefore, ways of reversing or modifying the drug action may be provided by finding genes whose expression possibly will underlie these phenomena. Also, there are many reports about other changes in gene expression induced by morphine (13, 14); but, changes in gene expression in the testis upon addiction to drugs abused are rarely known. In this study, to select a gene, we selected the entire genes associated with male infertility through the NCBI Entrez-Gene database using the keywords “male infertility”, “spermatogenesis”. The results showed that CREM, TBP, CREB1, HDAC1, and FOS had the most relationship with male infertility. In this study, we studied the effect of morphine toxicity on these genes. Chronic morphine treatment was found to change the expression of FBJ osteosarcoma oncogene (FOS) and cAMP-responsive element-binding protein 1 (CREB1), cAMP-responsive element modulator (CREM) (13) in the striatum and nucleus accumbens (13) it also increases the degeneration tissue, cytokines, stress signals such as free oxygen radicals (14), mitogenic stimulation (15), infections, and oncogenic compounds (16) and oxidative stress (17); also, it decreases growth factor in the various organs of rats (18). Another study revealed opioids’ effect on the expression of histone deacetylase 1 (HDAC1) and inducing tumor necrosis in the rat (19); also, HDAC1 -located in the nucleus of Sertoli cells of the testis- is involved in the spermatogenesis pathway (20). A series of experiments showed that the lack of expression of TATA-box-binding protein (TBP) suppressed the transcription of RNA polymerase III during mitosis (21). Given the potential adverse effects of morphine on male rat fertility, we aimed to determine the expression of these target genes involved in fertility-related pathways in opiate-addicted male rats, as well as the serum reproductive hormone levels.
Experimental
Animals
For this study, 30 male Wistar rats (200–250 g) were purchased from Tehran Pasteur Institute and kept in standard cages at the animal house of the Iran University of Medical Sciences. Before the experiments, the animals were fed a normal diet and water to acclimatize them to the environment and establish physiological adaptation. Throughout the study, the rats were kept under similar conditions, at a temperature of 22 ± 2 °C with 12 h light and 12 h darkness and free access to water and food. All experiments were conducted according to the guide for the care and use of laboratory animals, and were approved by the research and ethics committee of the Abadan University of Medical Sciences (IR-ABDANUMS.1395.121).
Chemicals
Morphine (C16H19NO3) was obtained from TEMAD Chemical Company (Tehran, Iran) and naloxone hydrochloride from Darou Pakhsh (Tehran, Iran). Both drugs were dissolved in saline (0.9%) for administration (22).
Experimental protocol
Rats were divided into three groups (n = 10): morphine group, saline and control groups.
Morphine administration
Morphine was freshly dissolved in 0.9% saline solution before each administration. Rats received repeated injections of the same dose (10 mg/kg [sc], twice/day, for 20 consecutive days). The saline group received a subcutaneous (S.C) injection of saline (1 mL/kg) at the same volume twice daily for 20 consecutive days. in the last control groups, the animals didn’t receive any drugs. Injections were performed at 09:00 and 15:00.
To determine the best protocol for the induction of addiction to morphine, a single dose of morphine (10 mg/kg) was used to determine its magnitude. In this set of experiments, rats received a dose of morphine (10 mg/kg [sc]) twice/day for 20 days. After that, On the twentieth day, naloxone (1 mL/kg) was injected 30 min after last morphine injection and morphine withdrawal signs were monitored (23, 24). The withdrawal symptoms included tremors and movement of the limbs off the baseline floor.
Hormone assay
Blood serum was separated from the collected blood by centrifugation (4000 ×g for 10 min). The serum samples were stored in a deep freezer at −20 °C. The blood testosterone, LH, and FSH concentrations were measured by enzyme-linked immunosorbent assays (Abcam 108666, Cambridge, MA, USA) (4).
RNA extraction and real-time quantitative reverse-transcription PCR
Total RNAs from the testis tissue ere extracted and purified with TRIzol reagent (Sigma, Pool, UK), following themanufacturer’s instructions. The RNA concentration was measured with a NanoDrop ND-100 spectrophotometer. The reverse transcription-polymerase chain reaction (RT-PCR) was performed to monitor the gene expression levels of HDAC1, CREM, TBP, FOS, and CREB1. A 500-ng sample of RNA was reverse transcribed with the Transcriptor High Fidelity cDNA Synthesis Kit (Invitrogen, Paisley, UK), using oligo(dT) primers (Roche, Basel, Switzerland). One microliter of the cDNA was amplified using the Opticon II system (Invitrogen, Paisley, UK) and the SYBR Green PCR Master Mix (Invitrogen, Paisley, UK), following the manufacturer’s instructions. Forty PCR cycles were performed, using an annealing temperature of 60 °C for all the genes tested. Primers were specifically designed between two adjacent exons (Gene Runner program); the sequences used in this study are presented in Table 1. The mRNA levels of these genes (Ct) were normalized to that of the reference gene glyceraldehyde-3-phosphate dehydrogenase (GAPDH) by subtracting the Ct value of GAPDH from the Ct value of the sample (ΔCt = CT Sample – CT Reference). The relative expression of the target gene to the calibrator was quantified using 2-ΔΔCt.
Western Blot (WB)
Based on materials,criteria WB was performed as described previously (25). In brief, after testis removal, complete testis was bilaterally micro dissected on ice, snap frozen and stored at −80 °C. Samples were homogenized with complete protease inhibitor cocktail (Roche, Mannheim, Germany), centrifuged and protein levels were measured according to Bicinchoninic Acid (BCA) protein assay method (Sigma-Aldrich). An equal amount of total protein (30 mg) was resolved on SDS-PAGE gels (8–10%) and transferred to polyvinylidene fluoride (PVDF) membranes via electrophoretic transfer system (Bio-Rad, Munchen, Germany). The membranes were blocked and incubated with specific primary antibodies at 4 °C overnight. Primary antibodies were rat monoclonal antibodies to HDAC1 (1:500, Santa Cruz Biotechnology), CREM (1:500, Santa Cruz Biotechnology), TBP (1:500, Santa Cruz Biotechnology), FOS (1:500, Santa Cruz Biotechnology), CREB1(1:500, Santa Cruz Biotechnology) as well as mouse monoclonal antibodies to GAPDH (1:500, Santa Cruz Biotechnology) to monitor loading. The membranes were washed with PBS, 0.05% Tween-20 (PBS-T), and incubated with respective HRP conjugated secondary antibodies (1:1000) at 4 °C for 4 h. The blots were exposed to HRP substrate solution (3, 3′-Diaminobenzidine and H2O2) for detection of target antigens. After staining, bands intensity was quantified using ImageJ (http://rsb.info.nih.gov/ij/) software after background subtraction and band density normalization.
Statistical analysis
The normal distribution of the data was evaluated using the Kolmogorov-Smirnov test. All data are presented as the mean ± SEM. The statistical significance of differences between the groups was determined using one-way analysis of variance, followed by Tukey’s post-hoc test. Statistical Package for the Social Sciences software (version 16.0, SPSS Inc., Chicago, IL, USA) was used for all the analyses. A value of P < 0.05 was considered statistically significant.
Results
Effects of morphine on serum reproductive hormone levels
As shown in Figure 1, the mean serum levels of LH and testosterone were significantly lower in the opiate-addicted subjects than in animals of the control and saline groups (P < 0.05). The FSH levels were also lower in the morphine group, although the difference was not statistically significant (P > 0.05).
Effects of morphine on genes of the male fertility-related pathways
The mRNA levels of HDAC1 were significantly lower in the morphine group than in the saline and control groups (P < 0.001) (Figure 2).
The mRNA levels of CREM and TBP were significantly lower in the morphine group than in the saline and control groups (P < 0.001) (Figure 3).
The mRNA levels of CREB1 and FOS were significantly lower in the morphine group than in the saline and control groups (P < 0.001) (Figure 4).
Confirmation of PCR-real time results by western blot analysis
Our PCR-real time results were confirmed by western blot analysis of all proteins, including HDAC1, CREM, TBP, CREB1, FOS (Figure 5). The expression of these proteins was significantly down-regulated (P < 0.05) in male rats with addict compared with control and saline.
Discussion
Our study detected significant associations of opiate addiction with the impairment of male fertility-related parameters and decreased markers in fertility-related pathways. Besides, the serum LH and testosterone levels were significantly lower in the addicted rats than in the saline and control rats. Despite the presence of hypotestosteronemia, low LH, and impaired spermatogenesis, the serum level of FSH did not increase in the addicted rats. These results are consistent with previous studies demonstrating that opiate consumption can result in a state of hypogonadotropic hypogonadism (26). In another similar study, it was shown that the administration of morphine to male rats led to a decrease in serum levels of LH and testosterone and decreases in the testis weight and sperm production (27). A subsequent study by Lee et al. in 1978 on addicts confirmed the lowering of the blood testosterone levels, but changes in the FSH and LH levels were not determined at that time (28). In animal studies conducted on rats and pigs, there was no change in the FSH levels despite a significant decrease in the LH and testosterone levels (29). Drug use appears to change the sexual function and the level of associated hormones (29). A decline in male fertility has occurred in recent years, with one of the main reasons for increased exposure to toxicants in the environment. These agents may be chemical materials, stress, and ionizing radiation, as well as substance abuse (30, 31). In line with our data, this study showed that administration of morphine also reduced serum reproductive hormones and confirmed that the damage induced by morphine caused to spermatogenesis.
Since previous studies have investigated the effect of opioids on male infertility, they examined various molecular pathways in testicular tissues. They showed that drug use was effective on pathways involved in infertility. In our present study, the morphine group had a significantly lower expression of male fertility genes. In addition, this finding implies that the impaired male fertility found in some cases could be due to drug influences in the hypothalamus and pituitary gland anda direct effect on the DNA integrity in testis tissue. The genes and proteins related to spermatogenesis and metabolism can be effective in normal sperm function. One of these genes is HDAC1, which is present in the nucleus of the Sertoli cells responsible for producing sperm. On the other hand, opiates have receptors in different parts of the testicles, including Sertoli cells and seminiferous tubules, which confirms their direct impact on spermatogenesis. They affect the HDAC1 gene in the nucleus of Sertoli cells and disrupt the spermatogenesis cycle; this gene has a critical role in regulating the changes in the major histones and in the control of chromatin changes that affect the transcription of tumor suppressor genes, causing tumorous and pathological changes in the tissue (20). HDAC1 is linked to different pathways, including the mTOR, MAPK, Notch, Hippo and Wnt signaling pathways associated with cell growth, proliferation and differentiation (32).
Different studies have shown that disruption in each of these pathways is associated with infertility in both men and women (33, 34). A study conducted by Jee Hyun Kim in 2014 showed that the level of HDAC1 expression in men with azoospermia was lower than that of normal people and attributed it to the reduction of HDAC1 gene expression with sperm DFI (35).
The assessment of CREB, FOS in our study showed that its protein expression was diminished in the addict animal which is in agreement with a previous research Elena H. Chartoff et al. suggested that decrease CREB1, FOS activation in portions of the striatum is related to addict by morphine (36). The gene transcript for CBP that is a protein binds to CREB (CBP or Crebbp) and strongly linked protein p300 are essential cofactors for many nuclear transcription factors (37). Since direct pathways for CBP activation by GnRH and insulin, i.e., mitogen-activated protein kinase (MAPK) and PKC can phosphorylate CBP, CBP action in the gonadotroph was studied (38). As FSH increases, it affects the Sertoli receptors and increases the adenylate cyclase and cAMP levels, resulting in phosphorylation of the CREB1 transcription factor on serine-133 (39). CREB1 induces the transcription factors required to activate other genes involved in spermatogenesis (40). It regulates the hormones that regulate spermatogenesis and anti-apoptotic factors (41). According to the data shown by the String database, there is an interaction between FOS with Interleukins receptor, apoptosis-related cysteine peptidase (CASP1), Mitogen-activated protein kinase 14 (MAPK14), Jun proto-oncogene (JUN), and FBJ murine (42); in addition, according to another study, in evaluating specific types of male infertility, Interleukins and FOS in seminal plasma should be extended; Thus, the changed FOS influences the innate immunity level in male infertility (43).
Studies have shown that CREB1, CREM, and FOS in the mouse and rat spermatogonial stem cell model helps the process of spermatogenesis by activating the Ras/Erk1/2 pathway and CDK2 promoter (44, 45). For expressing the late spermatogenic genes, there is a known master switch, including several spermatid-specific transcriptional regulators. The transcriptional activator CREMT, highly expressed in round spermatids is essential for expressing many important postmeiotic genes, for instance, Prm1, Prm2, Tnp1 and Tnp2, is encoded by the Crem gene (46, 47).
The coordinated action of a set of general transcription factors is necessary for transcription initiation by RNA polymerase II in eukaryotes (48, 49). The TBP is one of the major factors in transcription initiation; as well, during spermatogenesis, it shows a stage-specific expression pattern (50). Previous studies showed that testicular tissue in sterilized male mice was investigated, and the number of genes involved in spermatogenesis. The number of sperms in these mice was also evaluated. This study showed that spermatozoa were decreased and spermatogenesis was controlled by the TBP gene and TBP has been shown to affect the various factors involved in spermatogenesis (51).
Vesselin M. Chorbov et al. (2011) showed that DNA methylation in addicted men’s sperm was higher than those who quit the addiction. Hypermethylation of CpG loci in the promoter of genes involved in suppressing necrotic tumors, such as HDAC1 and HDAC1 gene expression, decreases. Increased DNA methylation in sperm may represent a method of epigenetic inheritance of opioid abuse or dependence phenotypes (52). According to Betina González in 2018, drug addiction modifies epigenetic homeostasis and next-generation outcomes, increases methylation of cytosine levels in sperm DNA and germ cells, and decreases HDAC1 gene expression. It also decreases spermatogenesis, which has a higher gene expression rate with drug withdrawal but is lower than normal (53). Jinghua Wang (2007) stated that morphine chronically inhibits interleukin-2 (IL-2) at the genomic and protein levelresulting in the inhibition of the CREB gene. In addition, chronic morphine treatment inhibits acetylation and trimethylation of histones and decreases DNA demethylation and access to the IL-2 promoter. These findings suggest that chronic morphine treatment may act through both transcriptional and epigenetic mechanisms to inhibit the IL-2 production and ultimately reduce CREB gene expression (13). Alcohol, cocaine, and nicotine increase methylation of cytosine levels in DNA and decrease FOS gene expression (54). According to another study, cellular and molecular examination in mice showed that spermatogonia stem cells were necessary for sperm production. Also, knocking down a set of genes, especially the TBP gene, reduces the production of sperm, which also increases apoptosis rates in these rats (55).
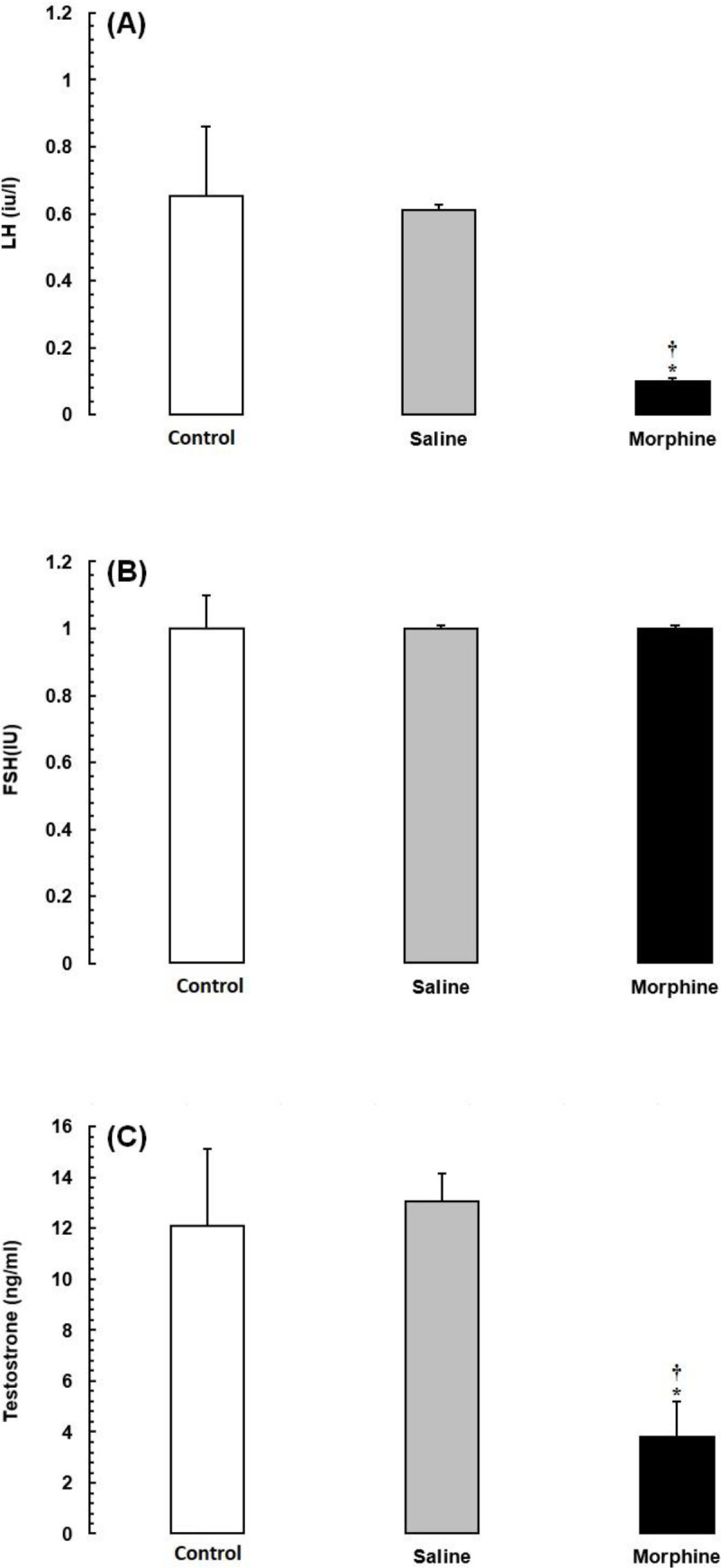
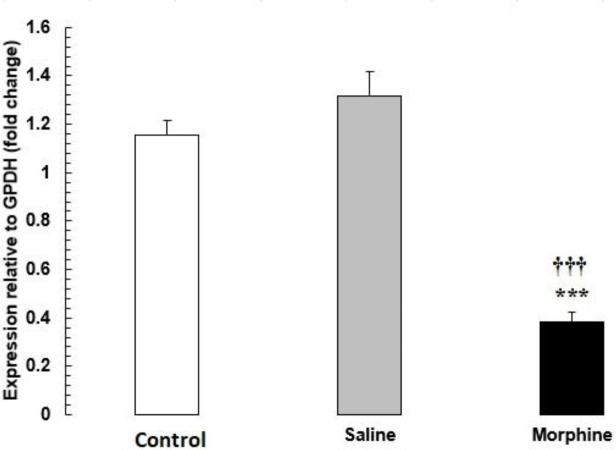
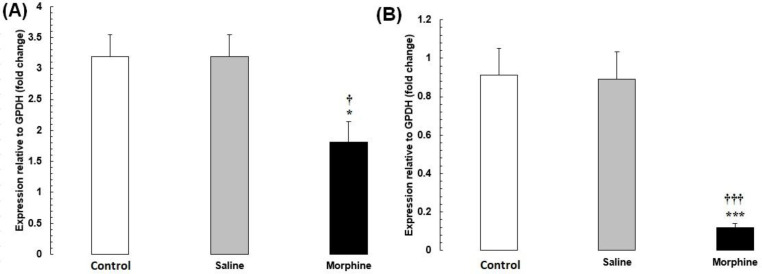
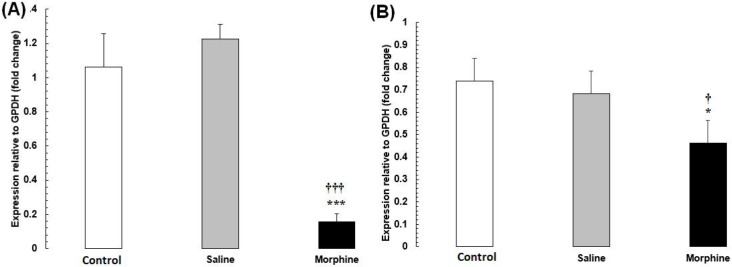
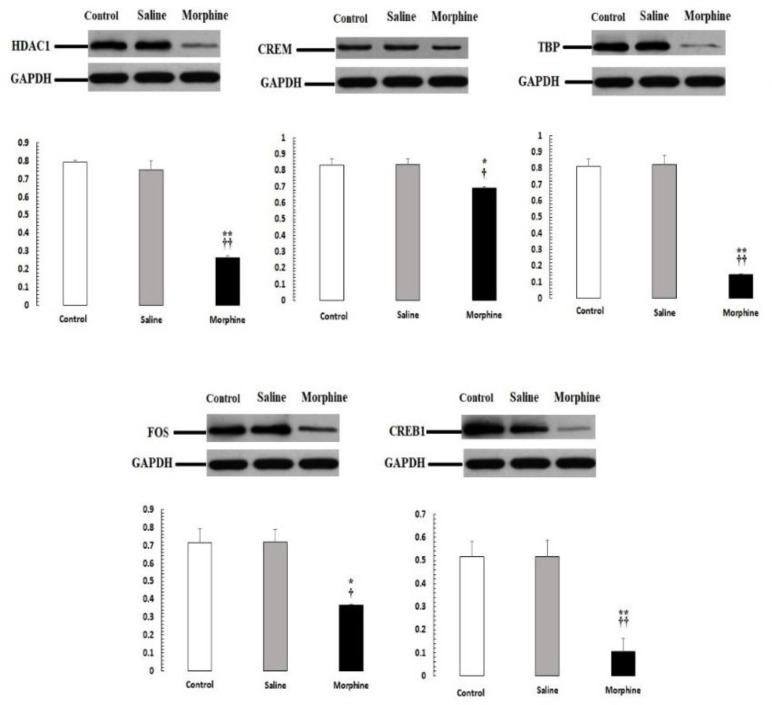
Sequence of primers used in the current investigation
Gene | Sequence (5'->3') | Length | Tm | GC (%) | Self-complementarity | Self-3' complementarity | |
---|---|---|---|---|---|---|---|
HDAC1 | Forward primer | GACGGGGATGATGGAAACTAC | 21 | 58.16 | 52.38 | 3.00 | 0.00 |
Reverse primer | GTTGGATTTGTGAGGACGATAG | 22 | 56.87 | 45.45 | 2.00 | 2.00 | |
CREM | Forward primer | GAAACAACATAGGGTAGAAAGGG | 23 | 56.85 | 43.48 | 2.00 | 0.00 |
Reverse primer | GAAAATGAGCACAACACTGGATG | 23 | 58.77 | 43.48 | 4.00 | 1.00 | |
TBP | Forward primer | ATCTTCATCCTTGTCCTCCAGCTTC | 25 | 62.38 | 48.00 | 4.00 | 0.00 |
Reverse primer | GCTCCCTCCAAAGCAATCTTCCTTA | 25 | 62.85 | 48.00 | 6.00 | 4.00 | |
FOS | Forward primer | GGTCCTGTCTGGTTCCTTCTATG | 23 | 60.12 | 52.17 | 3.00 | 0.00 |
Reverse primer | CTGCCTTGTCTGACTGCTCAC | 21 | 61.21 | 57.14 | 5.00 | 1.00 | |
CREB1 | Forward primer | CAG TTG TTA TGG CGTCCT | 18 | 54.61 | 50.00 | 2.00 | 2.00 |
Reverse primer | CTT GCT GCT TCC CTG TTC | 18 | 55.99 | 55.56 | 3.00 | 0.00 | |
GAPDH | Forward primer | CAT ACT CAG CAC CAG CAT CAC C | 22 | 61.32 | 54.55 | 3.00 | 0.00 |
Reverse primer | AAG TTC AAC GGC ACA GTC AAG G | 22 | 61.58 | 50.00 | 5.00 | 0.00 |
Conclusion
In conclusion, we have indicated for the first time the adverse effects of morphine on key molecules involved in fertility-related pathways in the male rat. It appears that morphine could decrease the reproductive parameters in this animal model of addiction. We suggest that morphine was able to affect the downregulation of the key molecule in the fertility-related pathways in the male rat.
Acknowledgements
References
-
1.
Cicero TJ, Davis LA, LaRegina MC, Meyer ER, Schlegel MS. Chronic opiate exposure in the male rat adversely affects fertility. Pharmacol. Biochem. Behav. 2002;72:157-63. [PubMed ID: 11900783].
-
2.
Nieschlag E. Scopes and goals of andrology. In: Nieschlag E, Behre HM, editors. Andrology: Male Reproductive Health and Dysfunction. Springer-Verlag; 2000. p. 1-8.
-
3.
Kumar N, Singh AK. Trends of male factor infertility, an important cause of infertility: A review of literature. J. Hum. Reprod. Sci. 2015;8:191-6. [PubMed ID: 26752853].
-
4.
Smeriglio VL, Wilcox HC. Prenatal drug exposure and child outcome. Past, present, future.Clin. Perinatol. 1999;26:1-16. [PubMed ID: 10214540].
-
5.
Li S, Pelletier G. Opioid regulation of gonadotropin-releasing hormone gene expression in the male rat brain as studied by in situ hybridization. Neuroreport. 1993;4:331-3. [PubMed ID: 8477057].
-
6.
Vuong C, Van Uum SH, O’dell LE, Lutfy K, Friedman TC. The effects of opioids and opioid analogs on animal and human endocrine systems. Endocrine Rev. 2009;31:98-132. [PubMed ID: 19903933].
-
7.
Gabriel SM, Simpkins JW, Kalra SP, Kalra PS. Chronic morphine treatment induces hypersensitivity to testosterone-negative feedback in castrated male rats. Neuroendocrinology. 1985;40:39-44. [PubMed ID: 2982110].
-
8.
Coluzzi F, Billeci D, Maggi M, Corona G. Testosterone deficiency in non-cancer opioid-treated patients. J. Endocrinol. Investig. 2018;41:1377-88. [PubMed ID: 30343356].
-
9.
Prasad GL, Naik BR, Ko JE, Nagaraju GP. Effects of naloxone, serotonin, and dopamine on reproduction of the freshwater crab Barytelphusa guerini. J. Exp. Zool. A Ecol. Genet. Physiol. 2014;321:173-82. [PubMed ID: 24273212].
-
10.
Salahshoor MR, Haghjoo M, Roshankhah S, Makalani F, Jalili C. Effect of thymoquinone on reproductive parameter in morphine-treated male mice. Adv. Biomed. Res. 2018;7:18. [PubMed ID: 29456989].
-
11.
Fronczak CM, Kim ED, Barqawi AB. The insults of illicit drug use on male fertility. J. Androl. 2012;33:515-28. [PubMed ID: 21799144].
-
12.
Ma J, Yuan X, Qu H, Zhang J, Wang D, Sun X, Zheng Q. The role of reactive oxygen species in morphine addiction of SH-SY5Y cells. Life Sci. 2015;124:128-35. [PubMed ID: 25623851].
-
13.
Wang J, Barke RA, Roy S. Transcriptional and epigenetic regulation of interleukin-2 gene in activated T cells by morphine. J. Biol. Chem. 2007;282:7164-71. [PubMed ID: 17227776].
-
14.
Cobellis G, Meccariello R, Fienga G, Pierantoni R, Fasano S. Cytoplasmic and nuclear Fos protein forms regulate resumption of spermatogenesis in the frog, Rana esculenta. Endocrinology. 2002;143:163-70. [PubMed ID: 11751605].
-
15.
Ebling FJ, Brooks AN, Cronin AS, Ford H, Kerr JB. Estrogenic induction of spermatogenesis in the hypogonadal mouse. Endocrinology. 2000;141:2861-9. [PubMed ID: 10919273].
-
16.
Minucci S, Matteo LD, Pierantoni R, Varriale B, Rastogi RK, Chieffi G. In-vivo and in-vitro stimulatory effect of a gonadotropin-releasing hormone analog (HOE 766) on spermatogonial multiplication in the frog, Rana esculenta. Endocrinology. 1986;119:731-6. [PubMed ID: 3089762].
-
17.
Persengiev SP, Robert S, Kilpatrick DL. Transcription of the TATA binding protein gene is highly up-regulated during spermatogenesis. Mol. Endocrinol. 1996;10:742-7. [PubMed ID: 8776734].
-
18.
Packer A, Besmer P, Bachvarova R. Kit ligand mediates survival of type A spermatogonia and dividing spermatocytes in postnatal mouse testes. Mol. Reprod. Dev. 1995;42:303-10. [PubMed ID: 8579844].
-
19.
Cherng CH, Lee KC, Chien CC, Chou KY, Cheng YC, Hsin ST, Lee SO, Shen CH, Tsai RY, Wong CS. Baicalin ameliorates neuropathic pain by suppressing HDAC1 expression in the spinal cord of spinal nerve ligation rats. J. Formos. Med. Assoc. 2014;113:513-20. [PubMed ID: 23684218].
-
20.
Jia H, Morris CD, Williams RM, Loring JF, Thomas EA. HDAC inhibition imparts beneficial transgenerational effects in Huntington’s disease mice via altered DNA and histone methylation. Proc. Natl. Acad. Sci. U S A. 2015;112:E56-E64. [PubMed ID: 25535382].
-
21.
Gottesfeld JM, Wolf VJ, Dang T, Forbes DJ, Hartl P. Mitotic repression of RNA polymerase III transcription in vitro mediated by phosphorylation of a TFIIIB component. Science. 1994;263:81-4. [PubMed ID: 8272869].
-
22.
Yilmaz B, Konar V, Kutlu S, Sandal S, Canpolat S, Gezen MR, Kelestimur H. Influence of chronic morphine exposure on serum LH, FSH, testosterone levels, and body and testicular weights in the developing male rat. Arch. Androl. 1999;43:189-96. [PubMed ID: 10624501].
-
23.
Mishra PR, Barik M, Ray SB. Effect of nimodipine on morphine-related withdrawal syndrome in rat model: an observational study. J. Pediatr. Neurosci. 2017;12:7-14. [PubMed ID: 28553371].
-
24.
Fujiwara A, Shimosawa M, Lino M. Physical dependence and plasma concentrations of morphine in rats. J. Drug Abuse. 2016;2:3.
-
25.
Sanadgol N, Golab F, Askari H, Moradi F, Ajdary M, Mehdizadeh M. Alpha-lipoic acid mitigates toxic-induced demyelination in the corpus callosum by lessening of oxidative stress and stimulation of polydendrocytes proliferation. Metab. Brain Dis. 2018;33:27-37. [PubMed ID: 29022246].
-
26.
Cicero TJ, Adams ML, Giordano A, Miller BT, O’Connor L, Nock B. Influence of morphine exposure during adolescence on the sexual maturation of male rats and the development of their offspring. J. Pharmacol. Exp. Ther. 1991;256:1086-93. [PubMed ID: 2005573].
-
27.
Salahshoor MR, Haghjoo M, Roshankhah S, Makalani F, Jalili C. Effect of thymoquinone on reproductive parameter in morphine-treated male mice. Adv. Biomed. Res. 2018;7:18. [PubMed ID: 29456989].
-
28.
Lee V, De Kretser D, Hudson B, Wang C. Variations in serum FSH, LH and testosterone levels in male rats from birth to sexual maturity. J. Reprod. Infertil. 1975;42:121-6.
-
29.
Chehrei S, Moradi M, Ghiabi H, Falahi M, Kaviani S, Ghanbari A. Pentoxifylline besides naltrexone recovers morphine-induced inflammation in male reproductive system of rats by regulating Toll-like receptor pathway. Andrologia. 2017;49:e12749.
-
30.
James RW, Heywood R, Crook D. Effects of morphine sulphate on pituitary-testicular morphology of rats. Toxicol. Lett. 1980;7:61-70. [PubMed ID: 7292515].
-
31.
Bablok L, Fracki S, Wielgos M, Czaplicki M, Marianowski L. The naloxone test in the degenerative changes of seminiferous tubules. Ginekol Pol. 1998;69:374-9. [PubMed ID: 9695346].
-
32.
Zhang JL, Yuan XB, Chen SJ, Chen HH, Xu N, Xue WH, Fu SJ, Zhang CX, Xu HJ. The histone deacetylase NlHDAC1 regulates both female and male fertility in the brown planthopper, Nilaparvata lugens. Open Biol. 2018;8:180158. [PubMed ID: 30977704].
-
33.
Ghaffari Novin M, Mirfakhraie R, Nazarian H. Aberrant Wnt/β-Catenin signaling pathway in testis of azoospermic men. Adv. Pharm. Bull. 2015;5:373-7. [PubMed ID: 26504759].
-
34.
Huang B, Butler R, Miao Y, Dai Y, Wu W, Su W, Fujii-Kuriyama Y, Warner M, Gustafsson JÅ. Dysregulation of Notch and ERα signaling in AhR-/- male mice. Proc. Natl. Acad. Sci. U S A. 2016;113:11883-8. [PubMed ID: 27688768].
-
35.
Kim JH, Jee BC, Lee JM, Suh CS, Kim SH. Histone acetylation level and histone acetyltransferase/deacetylase activity in ejaculated sperm from normozoospermic men. Yonsei Med. J. 2014;55:1333-40. [PubMed ID: 25048493].
-
36.
Chartoff EH, Papadopoulou M, Konradi C, Carlezon Jr WA. Dopamine-dependent increases in phosphorylation of cAMP response element binding protein (CREB) during precipitated morphine withdrawal in primary cultures of rat striatum. J. Neurochem. 2003;87:107-18. [PubMed ID: 12969258].
-
37.
Taghipoor Asramy A, Ghanbari-Niaki A, Naghizadeh Qomi M, Moghanny Bashi MM. The effect of running with bee pollen on muscle ABCA1 and APOA-1 mRNA expression in rats: brief report. Tehran Univ. Med. J. 2016;74:530-4.
-
38.
He L, Sabet A, Djedjos S, Miller R, Sun X, Hussain MA, Radovick S, Wondisford FE. Metformin and insulin suppress hepatic gluconeogenesis through phosphorylation of CREB binding protein. Cell. 2009;137:635-46. [PubMed ID: 19450513].
-
39.
Yun CY, You ST, Kim JH, Chung JH, Han SB, Shin EY, Kim EG. p21-activated kinase 4 critically regulates melanogenesis via activation of the CREB/MITF and β-catenin/MITF pathways. J. Invest. Dermatol. 2015;135:1385-94. [PubMed ID: 25560280].
-
40.
Guerriero G, Trocchia S, Abdel-Gawad FK, Ciarcia G. Roles of reactive oxygen species in the spermatogenesis regulation. Front. Endocrinol. 2014;5:56.
-
41.
Rajamanickam GD, Kastelic JP, Thundathil JC. The ubiquitous isoform of Na/K-ATPase (ATP1A1) regulates junctional proteins, connexin 43 and claudin 11 via Src-EGFR-ERK1/2-CREB pathway in rat Sertoli cells. Biol. Reprod. 2017;96:456-68. [PubMed ID: 28203706].
-
42.
Zamani-Badi T, Karimian M, Azami-Tameh A, Nikzad H. Association of C3953T transition in interleukin 1beta gene with idiopathic male infertility in an Iranian population. Hum. Fertil. Camb;2017:1-7.
-
43.
Havrylyuk A, Chopyak V, Boyko Y, Kril I, Kurpisz M. Cytokines in the blood and semen of infertile patients. Cent. Eur. J. Immunol. 2015;40:337-44. [PubMed ID: 26648778].
-
44.
He Z, Jiang J, Kokkinaki M, Golestaneh N, Hofmann MC, Dym M. Gdnf upregulates c-Fos transcription via the Ras/Erk1/2 pathway to promote mouse spermatogonial stem cell proliferation. Stem Cells. 2008;26:266-78. [PubMed ID: 17962702].
-
45.
Waheeb R, Hofmann MC. Human spermatogonial stem cells: a possible origin for spermatocytic seminoma. Int. J. Androl. 2011;34:e296-e305. [PubMed ID: 21790653].
-
46.
Foulkes NS, Mellstrom B, Benusiglio E, Sassone-Corsi P. Developmental switch of CREM function during spermatogenesis: from antagonist to activator. Nature. 1992;355:80-4. [PubMed ID: 1370576].
-
47.
Nantel F, Monaco L, Foulkes NS, Masquilier D, LeMeur M, Henriksen K, Dierich A, Parvinen M, Sassone-Corsi P. Spermiogenesis deficiency and germ-cell apoptosis in CREM-mutant mice. Nature. 1996;380:159-62. [PubMed ID: 8600390].
-
48.
Hampsey M, Reinberg D. RNA polymerase II as a control panel for multiple coactivator complexes. Curr. Opin. Genet. Dev. 1999;9:132-9. [PubMed ID: 10322136].
-
49.
Orphanides G, Lagrange T, Reinberg D. The general transcription factors of RNA polymerase II. Genes Dev. 1996;10:2657-83. [PubMed ID: 8946909].
-
50.
Sassone-Corsi P. Transcriptional checkpoints determining the fate of male germ cells. Cell. 1997;88:163-6. [PubMed ID: 9008156].
-
51.
Ornellas F, Bringhenti I, Mattos BAN, Mandarim-de-Lacerda CA, Aguila MB. Father’s obesity programs the adipose tissue in the offspring via the local renin–angiotensin system and MAPKs pathways, especially in adult male mice. Eur. J. Nutr. 2018;57:1901-12. [PubMed ID: 28534168].
-
52.
Chorbov VM, Todorov AA, Lynskey MT, Cicero TJ. Elevated levels of DNA methylation at the OPRM1 promoter in blood and sperm from male opioid addicts. J. Opioid. Manag. 2011;7:258-64. [PubMed ID: 21957825].
-
53.
González B, Pantoja CRG, Sosa MH, Vitullo AD, Bisagno V, González CR. Cocaine alters the mouse testicular epigenome with direct impact on histone acetylation and DNA methylation marks. Reprod. Biomed. Online. 2018;37:269-78. [PubMed ID: 30126647].
-
54.
Yohn NL, Bartolomei MS, Blendy JA. Multigenerational and transgenerational inheritance of drug exposure: the effects of alcohol, opiates, cocaine, marijuana, and nicotine. Prog. Biophys. Mol. Biol. 2015;118:21-33. [PubMed ID: 25839742].
-
55.
Kushwaha R, Mishra J, Tripathi S, Khare P, Bandyopadhyay S. Arsenic, cadmium, and lead like troglitazone trigger PPARγ-dependent poly (ADP-ribose) polymerase expression and subsequent apoptosis in rat brain astrocytes. Mol. Neurobiol. 2018;55:2125-49. [PubMed ID: 28283887].