Abstract
Keywords
Diabetes Metformin Captopril Atorvastatin Aspirin Heart Kidney
Introduction
Diabetes mellitus (DM) is associated with an increased risk of cardiovascular and renal diseases (1, 2). Oxidative stress plays a pivotal role in the development of cardiovascular and renal complications of diabetes. Reactive oxygen species (ROS) were shown to exert a direct inhibitory effect on myocardial function and cause oxidation of various molecules; also, they induce redox genes overexpression, intracellular calcium overload, and DNA fragmentation, which all damage myocardial cells (3). Oxidative stress is also an important pathogenic mechanism underlying chronic kidney disease (4). It is becoming increasingly evident that cellular function changes that may induce oxidative stress play a key role in the development and progression of diabetic nephropathy (DN) (5).
Metformin is an anti-hyperglycemic drug that was shown to improve cardiovascular complications of diabetes (6). Metformin could reduce intracellular ROS levels by increasing the activity of the antioxidative system (7).
The role of the renin-angiotensin-aldosterone system (RAAS) and variations in genes encoding different components of RAAS in susceptibility towards diabetic nephropathy and cardiomyopathy were investigated in numerous studies. The effects of variants of some RAAS constituents genes, including angiotensin-converting enzyme (ACE), as well as angiotensinogen and angiotensin II type 1 receptor (AT1R), on the risk of DN, were extensively studied (8, 9). ACE inhibitors such as captopril were shown to attenuate the progression of diabetes-induced cardiac and renal impairments (10). These agents also protect the cardiovascular system and kidneys against ROS, probably via their superoxide anion scavenging potential (10, 11).
Dyslipidemia is commonly found in diabetic patients (12). Statins such as atorvastatin are used as lipid-lowering drugs in diabetic patients to reduce the risk of cardiovascular diseases (13). Atorvastatin, possibly through boosting antioxidant markers, can preserve myocardial structure which is considered a non-lipid lowering benefit of statins (14). Statins reduce ROS generated by vascular NAD(P)H oxidase and antagonize the pro-oxidant effect of angiotensin II (15). The antioxidant effect of statins contributes to inhibition of myocardial hypertrophy and remodeling and modulation of vascular tone (16). Statins treatment prevented glomerular injury, independent of their cholesterol-lowering effects and it was suggested that such beneficial effect might be mediated through pleiotropic effects including an anti-inflammatory activity by suppression of oxidative stress, NFκB activation, and induction of ICAM-1 expression and macrophage infiltration in the early phase of DN (17).
Aspirin (ASA) is widely recommended for primary prevention of cardiovascular events in DM (18, 19). In 2013, to prevent cardiovascular diseases, the American Diabetes Association proposed the use of aspirin in diabetic patients with a high risk of cardiovascular complications (20).
Aspirin protects the cardiovascular system by reducing the production of superoxide and preventing the reduction of catalase and superoxide dismutase activity (21). According to the development of diabetes in association with statin use, the introduction of novel therapeutic approaches for the treatment of diabetes is of crucial importance. Recent researches reported that high-dose ASA affects glucose and lipid metabolism (22-24). Also, as high-dose ASA, unlike statins, can inhibit serine kinase IKKβ activity, it might help overcome insulin resistance and improve glucose tolerance in patients with type 2 diabetes (25, 26).
This study was carried out to determine the effect of high-dose ASA alone and in combination with metformin, atorvastatin and captopril on oxidative stress in the heart and kidney tissues of streptozotocin (STZ)-induced diabetic rats.
Experimental
Chemicals and drugs
All drugs were obtained from Sigma (Germany).
Animals
Eighty-eight male Wistar rats (250–280 g and 10 weeks old) were obtained from Central Animal House of Mashhad University of Medical Sciences, Mashhad, Iran. They were kept at 22 ± 1°C with 12 h/12 h light/dark cycles, and they had ad libitum access to food and water. All experiments were approved by the Animal Experimentation Ethics Committee of Mashhad University of Medical Sciences (Approval No. 89529).
The protocol used to induce diabetes
Diabetes was induced by a single intraperitoneal (i.p.) dose of streptozotocin (STZ, 60 mg/kg) injected immediately after dissolving the agent in distilled water. Three days after STZ injection, we confirmed the induction of diabetes by measuring fasting glucose levels in blood samples collected after 8-12 hour fasting from orbital sinus. Rats with blood glucose level ≥ 250 mg/dL were considered diabetic (27).
Experimental design
Rats were randomly divided into the following eleven groups (n = 8 in each group): control (Cont.), diabetic rats (D), diabetic rats treated with metformin (D + M), diabetic rats treated with aspirin (D + ASA), diabetic rats treated with metformin and aspirin (D + M + ASA), diabetic rats treated with metformin and captopril (D + M +C), diabetic rats treated with metformin and atorvastatin (D + M + AT), diabetic rats treated with metformin, captopril and aspirin (D + M + C + ASA), diabetic rats treated with metformin, captopril and atorvastatin (D + M + C + AT), diabetic rats treated with metformin, atorvastatin and aspirin (D + M + AT + ASA), diabetic rats treated with metformin, captopril, atorvastatin and aspirin (D + M + C + AT + ASA) which were treated (by gavage) daily for six weeks. Normal saline was orally administered (by gavage) to the control and diabetic groups and the other groups received metformin (300 mg/kg), aspirin (120 mg/kg), captopril (50 mg/kg), and atorvastatin (40 mg/kg). All drugs were dissolved in normal saline.
Preparation and analysis of the samples
Ether was used to induce anesthesia and the blood samples were obtained from rats orbital sinus. The samples were centrifuged at 3000 rpm and the serum was kept at -20 °C until analysis. Serum fasting glucose concentration was measured at three different time-points namely, before STZ injection (day 0), three days after STZ injection (day 3, when diabetes was confirmed), and six weeks after STZ injection (day 45). Serum cholesterol, triglyceride, LDL, and HDL were measured on day 0 and 45 using Pars Azmoon kits (Iran). The atherogenic index of plasma (AIP) was calculated as log (TG/HDL-C) (28). At the end of the experimental period, rats were sacrificed following induction of deep anesthesia by ether. The heart and kidney tissues were quickly removed and washed with normal cold saline. The heart and kidney samples were then immediately homogenized. Supernatant fractions were obtained by centrifugation of the homogenates at 5000 ×g for 10 min and stored at -80 °C until measurement of oxidative stress parameters levels.
Measurement of total thiol
Total thiol was determined by the method previously described by Sedlak and Lindsay (29). The heart or kidney tissues were homogenized using PBS (phosphate-buffered saline). The supernatant of homogenized heart or kidney (50 μL) was added to 1 mL Tris-EDTA (ethylenediaminetetraacetic acid) buffer (pH 8.6) and the absorbance was read at 412 nm against Tris-EDTA buffer alone (A1). Then, 20 μL of 2, 2’ dinitro 5, 5’ dithiodibenzoic acid (DTNB) (10 mM in methanol) was mixed with the supernatant and the absorbance was read again (A2). The absorbance of the DTNB reagent was also read as blank (B). Total thiol was calculated using the following equation and expressed as mmol/g tissue (30).
Total thiol concentration (mM) = (A2 − A1 − B) × 1.07/(0.05 × 14150)
Assessment of lipid peroxidation
Malondialdehyde (MDA) level is an index of lipid peroxidation extent. MDA reacts with thiobarbituric acid (TBA) as a TBA reactive substance (TBARS) and produces a red complex. Briefly, the heart or kidney tissues were homogenized using PBS. Then, 1 ml of the supernatant of homogenized heart or kidney was added to 2 mL of a complex solution containing TBA, trichloroacetic acid (TCA), and hydrochloric acid (HCl); then, the mixture was boiled in a water bath for 40 min. After reaching room temperature, the solution was centrifuged at 1000 ×g for 10 min and the absorbance of the supernatant was read at 535 nm. The MDA levels were expressed as µmol/g tissue weight, using an extinction coefficient of 1.56 × 105 cm-1 M-1 (31, 32).
Determination of superoxide dismutase (SOD) activity
Superoxide dismutase (SOD) activity was measured by the protocol explained by Madesh and Balasubramanian (33). This colorimetric assay involves the generation of superoxide by pyrogallol auto-oxidation and inhibition of superoxide-dependent reduction of the tetrazolium dye, MTT (3-(4, 5-dimethylthiazol-2-yl) 2, 5-diphenyltetrazolium bromide) to formazan by SOD; the absorption of the solution was measured at 570 nm. One unit of SOD activity was defined as the amount of enzyme causing 50% inhibition in the MTT reduction rate (34).
Measurement of catalase (CAT) activity
To determine CAT activity, the method described by Aebi’s was employed (35). This assay is based on the determination of the rate constant, k, (dimension: s-1, k) of hydrogen peroxide decomposition. The rate constant of the enzyme was determined by measuring the reduction in absorbance at 240 nm per minute. One unit (U) was defined as the amount of enzyme which decomposes 1 mol of H2O2 per min at 25 °C and pH 7.0 (35, 36).
Statistical analysis
Data were expressed as mean ± SEM. Statistical analyses were done using one-way ANOVA followed by Tukey’s post hoc test by SPSS 16. Statistical significance was defined as p < 0.05.
Results
Fasting blood glucose
Fasting serum glucose levels on days 3 and 45 in the non-treated diabetic group (i.e., group D) and in groups that received different combinations of metformin, aspirin, atorvastatin, and captopril, were significantly higher than that of the control group (p < 0.001 for the non-treated diabetic group on day 45, p < 0.01 for the non-treated diabetic group on day 3 and also for groups that received different combinations of drugs on days 3 and 45, p < 0.05 for D + M and D + M + C + AT + ASA groups on day 45). On day 45, fasting serum glucose levels in all drug-treated groups were significantly lower than that of the diabetic group (p < 0.01 for D + M + C + AT + ASA and D + M groups and p < 0.05 for other drug-treated groups, as compared to the diabetic group). There was no significant difference in serum glucose levels between the groups that received different combinations of these four drugs (Figure 1).
Serum lipid profile
On day 45, serum cholesterol levels in the non-treated diabetic group were significantly higher than the control group (p < 0.001). All drug-treated groups, except the aspirin-only-treated group, showed significant decreases in serum cholesterol levels compared to the non-treated diabetic group (p < 0.05 for groups that received one or two drugs but p < 0.01 for groups that received combinations of three or four drugs). Also, cholesterol levels in groups that received combinations of three or four drugs (i.e., D + M + C + ASA, D + M + C + AT, D + M + AT + ASA, and D + M + C + AT + ASA groups) showed significant decreases compared to groups that received one or two drugs (p < 0.05 and p < 0.01 compared to ASA group, p < 0.05 compared to D + M + ASA group and p < 0.05 compared to D + M + C group). Among the three groups that received combinations of two drugs, significant decreases in serum cholesterol levels were only observed in the D + M + ASA and D + M + AT groups, compared to the D + ASA group (Figure 2A).
The results showed significant increases in serum triglyceride levels in the non-treated diabetic group compared to the control group on day 45 (p < 0.001); however, serum triglyceride levels in groups that received combinations of three or four drugs were significantly lower than those of non-treated diabetic group (p < 0.01 to p < 0.001). Among the three groups treated with combinations of two drugs, only the D+M+AT group showed significant decreases in serum triglyceride levels compared to the non-treated diabetic group (p < 0.001). Also, triglyceride levels in groups that received combinations of three or four drugs (i.e., D + M + C + ASA, D + M + C + AT, D + M + AT + ASA, and D + M + C + AT + ASA groups) and D + M + AT group were significantly reduced as compared to groups treated with one medication or combinations of two drugs (p < 0.05 and p < 0.01 compared to D + M, D + ASA, D + M + ASA and D + M + C groups) In D + M + C + AT, D + M + AT + ASA, and D + M + C + AT + ASA groups, there were significant decreases in serum triglyceride levels compared to the D + M + C + ASA group (Figure 2B).
On day 45, serum LDL- cholesterol levels were significantly higher in non-treated diabetic (p < 0.001) and D+M groups (p < 0.05) compared to the control group. All drug-treated groups, except the aspirin-only-treated group, showed significant decreases in serum LDL- cholesterol levels compared to the non-treated diabetic group (p < 0.05 for groups that received one or two drugs and p < 0.01 for groups that received combinations of three or four drugs and D + M + AT group compared to the diabetic group). Also, LDL- cholesterol levels in all groups treated with different combinations of drugs were significantly lower compared to the ASA-only-treated group (p < 0.05) (Figure 2C).
Serum HDL- cholesterol levels on day 45, were significantly lower in non-treated diabetic (p < 0.01), D + ASA (p < 0.05) and D + M + C groups (p < 0.05) compared to the control group. All drug-treated groups, except the aspirin-only-treated group, showed significant increases in serum HDL- cholesterol levels compared to the non-treated diabetic group (p < 0.01 for the group that received a combination of four drugs and p < 0.05 for other drug-treated groups compared to the non-treated diabetic group). Also, HDL- cholesterol levels showed significant increases in the group who received a combination of four drugs (D + M + C + AT + ASA group) compared to the ASA-only-treated group (p < 0.05) (Figure 2D).
Atherogenic index of plasma
Atherogenic index of plasma (AIP) in the non-treated diabetic group and in all groups that received different combinations of metformin, aspirin, atorvastatin, and captopril, was significantly higher than that of the control group (p < 0.001 for the non-treated diabetic group, p < 0.01 for all groups that received combinations of two or one drugs and D + M + C + ASA group, p < 0.05 for groups that received combinations of three or four drugs and D + M + AT group) compared to the control group on day 45 (p < 0.001); however, groups that received combinations of three or four drugs showed significantly lower AIP compared to non-treated diabetic group (p < 0.01 to p < 0.001). Among groups that received combinations of two drugs, only the D + M + AT group had significantly reduced AIP compared to the non-treated diabetic group. Also, groups that received combinations of three or four drugs (i.e., D + M + C + ASA, D + M + C + AT, D + M + AT +ASA, and D + M + C + AT + ASA groups) and D + M + AT group had significantly decreased AIP compared to groups that received one or combinations of two drugs (Figure 3).
Malondialdehyde levels
Malondialdehyde (MDA) levels in the heart and kidney tissues were collected from the non-treated diabetic group (p < 0.001 and p < 0.01, respectively) and in groups that received one or combinations of two drugs (p < 0.05), were significantly higher than those of the control group. All drug-treated groups showed significantly lower MDA levels compared to the non-treated diabetic group (p < 0.05 for groups that received one or combinations of two drugs and p < 0.01 for groups that received combinations of three or four drugs) (Figure 4A and 4B).
Total thiol concentrations
Total thiol concentrations in the heart and kidney tissues collected from the non-treated diabetic group (p < 0.001 and p < 0.01, respectively) and in groups that received one or combinations of two drugs (p < 0.05) were significantly lower than those of the control group. Total thiol concentrations in the heart tissue of all drug-treated groups were significantly higher compared to the non-treated diabetic group (p < 0.05 for groups that received one or combinations of two drugs and p < 0.01 for groups that received combinations of three or four drugs). In the kidney tissue, only in groups that received combinations of three or four drugs, significantly lower levels of total thiol were observed as compared to the non-treated diabetic group (p < 0.05) (Figures 5A and 5B).
SOD and CAT activities
The SOD and CAT activities in the heart and kidney tissues of the non-treated diabetic group were significantly lower compared to the control group (p < 0.001 and p < 0.01, respectively). The heart tissues collected from all drug-treated groups had incredibly high levels of SOD activity compared to the non-treated diabetic group (p < 0.05 for groups that received one drug or combinations of two medications and p < 0.01 for groups that received combinations of three or four medications as compared to the diabetic group). In the kidney tissue obtained from groups that received combinations of three or four drugs and the D + M + C group, SOD activity was significantly increased compared to the non-treated diabetic group (p < 0.05 for D + M + C + AT + ASA group and p < 0.05 for other groups as compared to the diabetic group).
Also, significantly higher CAT activity was determined in the heart tissue collected from all drug-treated groups compared to the non-treated diabetic group (p < 0.05); however, only kidney tissues collected from groups that received combinations of three or four medications presented significant increases in CAT activity compared to the diabetic group (p < 0.05) (Figures 6A, 6B, 7A and 7B).
Discussion
The current findings suggested that treatment with aspirin, atorvastatin, metformin, captopril and different combinations can rectify the imbalance between the generation of ROS and the scavenging enzyme activity in the heart and kidney tissues of diabetic animals. Consistent with our findings, several studies showed the antioxidant effect of aspirin, atorvastatin, metformin and ACE inhibitors that led to reduction of lipid peroxidation and increment of total thiol concentration, and SOD and CAT activities that can be favorable for the kidney and cardiovascular system (37-40).
In this study, more marked improvements of oxidative stress factors were observed in groups that received combinations of three or four medications compared to groups that received one drug or combinations of two drugs, indicating that co-administration of these drugs synergistically enhance their antioxidant effect. Synergistic effects of co-administration of these drugs may be mediated via various antioxidative mechanisms induced by each of these medications; thus, a combination of these agents presents enhanced antioxidant effects. In this regard, metformin can decrease ROS through various mechanisms including inhibition of complex I of the electron transport chain, activation of glucose-induced protein kinase C-β2, reduction of NAD(P)H oxidase activity, inducing thioredoxin expression through activation of the AMPK-FOXO3 (Activated protein kinase- Forkhead box O3) pathway and increasing the activity of the antioxidative system (41-44, 6). Aspirin can prevent the generation of oxyradicals through inhibition of prostaglandins synthesis and induction of heme oxygenase-1 (HO-1) expression (45, 46). In addition, aspirin can increase the activity of the antioxidative system (21). Antioxidant effect of atorvastatin in diabetes may be mediated through inhibition of Rac-1 and geranylgeranyl pyrophosphate (GGPP), induction of heme oxygenase, interference with NAD(P)H oxidase expression and activity and antagonization of the pro-oxidant effect of angiotensin II (47-49, 15). Finally, captopril can decrease ROS levels via suppressing angiotensin II production, which stimulates the production of superoxide radicals by NADPH oxidase and uncoupled nitric oxide (NO) synthase (50).
In this study, blood glucose, cholesterol, TG, and LDL levels and AIP decreased, but HDL levels increased in drug-treated groups after 45 days. It should be noted that the administration of a combination of these medications produced more marked beneficial effects than the administration of each drug alone. In the aspirin-only-treated group (D + ASA), no significant alterations in cholesterol, LDL- cholesterol and HDL- cholesterol levels were found as compared to the non-treated diabetic group while in groups treated with metformin (D + M) or groups that received combinations of metformin and captopril (D + M + C), atorvastatin (D + M + AT) or aspirin (D + M + ASA), these factors were significantly improved; therefore, it can be concluded that aspirin alone has no positive effect on the improvement of lipid profile but in combination with other drugs, it could significantly improve lipid profile. Recent studies also reported that high-dose aspirin influences lipid metabolism (23, 24). In the present study, metformin could improve lipid profile when administered alone or in combination with captopril. These results are in line with data reported by previous studies demonstrating that metformin, as an antihyperglycaemic drug, as well as captopril can improve glucose and lipid metabolism (51-53).
In the present experiment, a comparison of the groups that received combinations of two medications indicated that a combination of metformin and atorvastatin (D + M + AT) could reduce cholesterol and LDL-cholesterol to a greater extent compared to the combination of metformin and aspirin (D + M + ASA) or captopril (D + M + C). Also, the combination of metformin and atorvastatin (D + M + AT) more markedly reduced TG than groups that received a combination of metformin, captopril and aspirin (D + M + C + ASA). Comparison of the groups that received combinations of three medications demonstrated that in groups where atorvastatin was present (i.e., D + M + C + AT and D + M + ASA + AT groups), there was a significant reduction in TG levels compared to the treatment that lacked atorvastatin (i.e., D + M + C + ASA group). These findings indicated the high capacity of atorvastatin in improving lipid profiles. In line with many previous studies, these results showed that atorvastatin has strong antihyperlipidemic activity (54).
Statins such as atorvastatin inhibit intracellular cholesterol storage by inhibiting HMG-COA reductase. As a result, they increase the expression of LDL receptors. Increased expression of these receptors led to increases in blood LDL clearance rate and decrease in plasma cholesterol levels (22).
The role of AMPK in the lipid metabolism induced by metformin and aspirin was reported (55). Metformin and aspirin suppress ATP utilization pathways such as lipid synthesis by activating AMPK. Metformin also reduces the concentration of cholesterol and LDL by inhibiting the intestinal absorption of bile acids (55). Moreover, captopril reduces insulin-stimulated lipogenesis by reducing glycerol synthesis from glucose. In other words, captopril reduces the lipogenesis capacity of adipocytes (56).
In this study, there was a significant decrease in AIP in groups that received combinations of three or four drugs compared to the non-treated diabetic group, but there was no significant reduction in AIP in groups treated with one or two drugs. One of the main risk factors and prognosticator for CVD (cardiovascular disease) is plasma lipid profile. AIP represents the correct relation between atherogenic and protective lipoprotein. Serious increase in AIP was observed as reflected by augmented TC, TG and LDL-C and reduced HDL-C. AIP act as a prognosticator for atherosclerosis, and can be used as an accurate indicator for cardiovascular risk factors (20, 21). Given that increasing evidence suggests AIP as an indicator of atherosclerosis, these results suggest that combinations of aspirin, atorvastatin, metformin and captopril could reduce AIP and consequently decrease CVD risk factors in DM (28). Moreover, comparing the groups that received combinations of two drugs showed that only in the D + M + AT group, AIP was significantly decreased compared to the non-treated diabetic group. This shows the high capacity of atorvastatin to improve lipid profile and, therefore, reduce AIP and CVD risk factors.
According to these results, to improve the lipid profile, the efficacy of different combinations was in the following order: a combination of four drugs > combinations of three drugs > combination of two drugs > aspirin alone; thus, it can be concluded that these drugs probably produce synergistic effects in terms of improvement of lipid profiles when administered together.
Very few studies were conducted to evaluate the effects of different combinations of these medications and there is little information in this regard. Berardis et al. showed putative additive effects of co-administration of aspirin and statins to control cardiovascular risk factors in diabetes (57). It was also revealed that the combination of statins with angiotensin II system inhibitors or AT-1 receptors blockers had more marked effects on oxidative stress (58, 59). Tousoulis showed that co-treatment with metformin and atorvastatin could be regarded as a useful intervention with significant antioxidant and antihyperlipidemic activities as reflected by reduced lipid peroxidation in diabetic patients (60).
The current findings suggested that in diabetic rats, oxidative stress was induced; however, high-dose aspirin in combination with metformin, captopril and atorvastatin can exert synergism in terms of rectifying the imbalance between ROS generation and scavenging enzymes activity and improving diabetic hyperglycemia and hyperlipidemia.
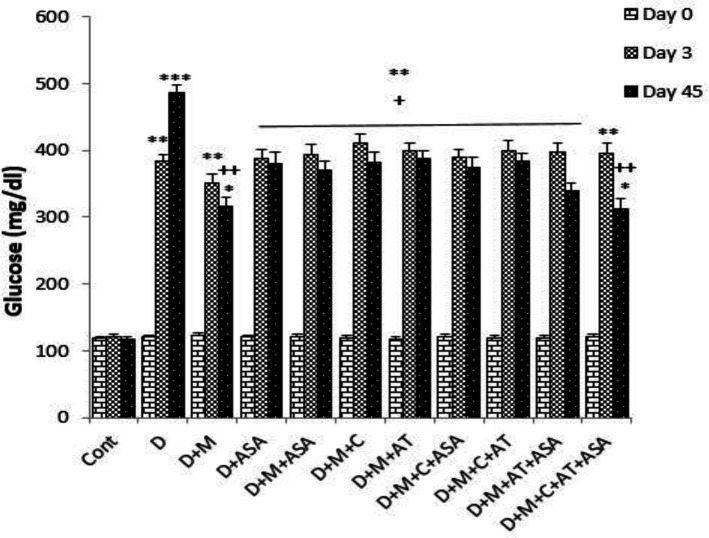
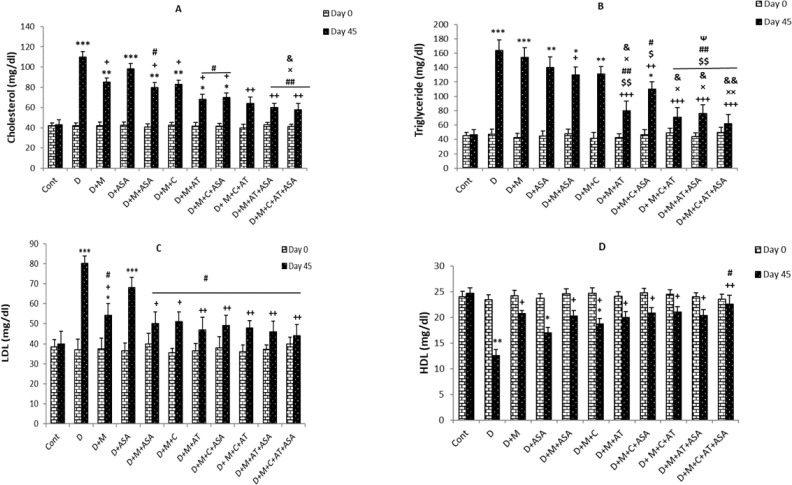
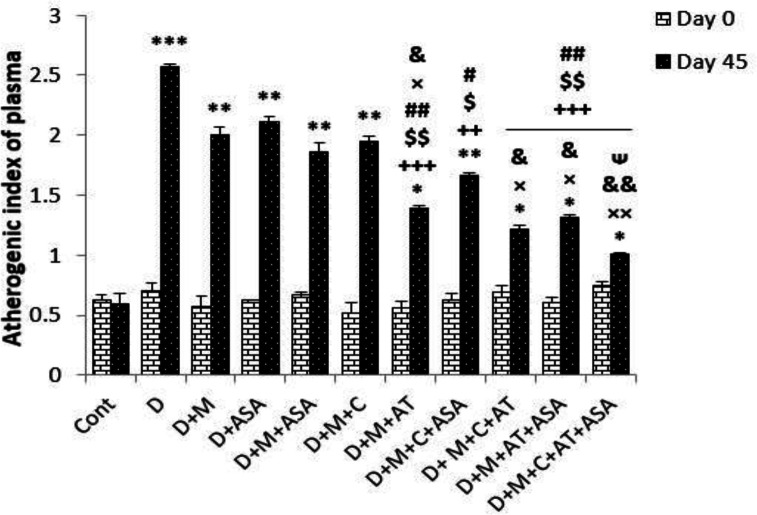
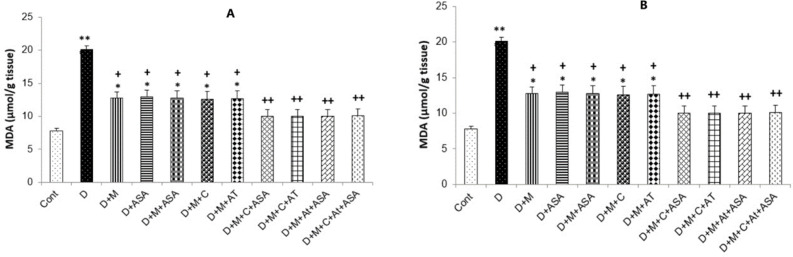
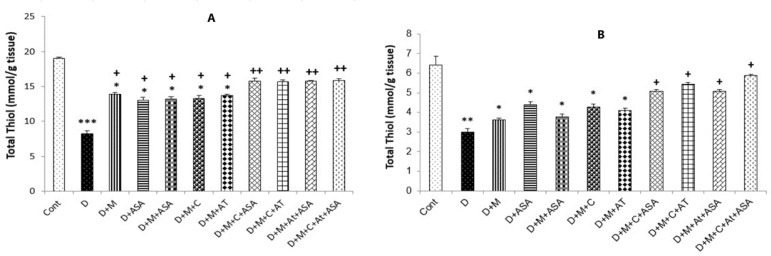
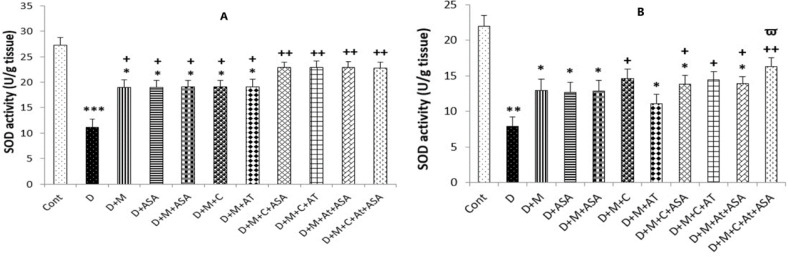
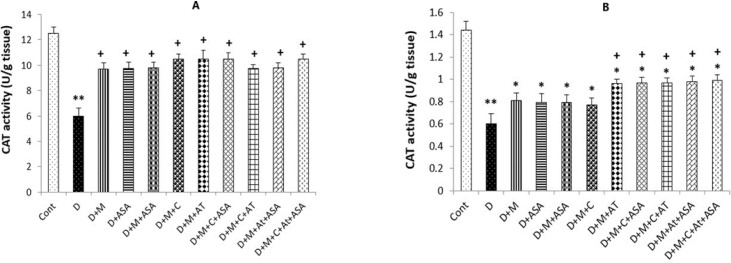
Conclusion
This study indicated that combinations of captopril, aspirin, atorvastatin and metformin could improve glucose and lipid metabolism and oxidative stress markers in the kidneys and heart tissues of diabetic rats to a greater extent as compared to their single administration.
Acknowledgements
References
-
1.
Suckling R, Gallagher H. Chronic kidney disease, diabetes mellitus and cardiovascular disease: risks and commonalities. J. Ren. Care. 2012;38:4-11. [PubMed ID: 22348359].
-
2.
Pálsson R, Patel UD. Cardiovascular complications of diabetic kidney disease. Adv. Chronic. Kidney Dis. 2014;21:273-80. [PubMed ID: 24780455].
-
3.
Kayama Y, Raaz U, Jagger A, Adam M, Schellinger IN, Sakamoto M, Suzuki H, Toyama K, Joshua M, Spin JM, Tsao PS. Diabetic cardiovascular disease induced by oxidative stress. Int. J. Mol. Sci. 2015;16:25234-63. [PubMed ID: 26512646].
-
4.
Boroushaki MT, Mollazadeh H, Rajabian A, Dolati K, Hoseini A, Paseban M, Farzadnia M. Protective effect of pomegranate seed oil against mercuric chloride-induced nephrotoxicity in rat. Ren. Fail. 2014;36:1581-6. [PubMed ID: 25154291].
-
5.
Nishikawa T, Brownlee M, Araki E. Mitochondrial reactive oxygen species in the pathogenesis of early diabetic nephropathy. J. Diabetes Investig. 2015;6:137-9.
-
6.
Petrie JR, Chaturvedi N, Ford I, Brouwers MC, Greenlaw N, Tillin T, Hramiak I, Hughes AD, Klein BEK, Klein R, Ooi TC, Rossing P, Stehouwer DA, Sattar N, Colhoun HM. Cardiovascular and metabolic effects of metformin in patients with type 1 diabetes (REMOVAL): a double-blind, randomised, placebo-controlled trial. Lancet Diabetes Endocrinol. 2017;5:597-609. [PubMed ID: 28615149].
-
7.
Mousavi SM, Niazmand S, Hosseini M, Hassanzadeh Z, Sadeghnia HR, Vafaee F, Keshavarzi Z. Beneficial effects of Teucrium polium and metformin on diabetes-induced memory impairments and brain tissue oxidative damage in rats. Int. J. Alzheimers Dis. 2015;2015:493729. [PubMed ID: 25810947].
-
8.
Rahimi Z. The role of renin angiotensin aldosterone system genes in diabetic nephropathy. Can. J. Diabetes. 2016;40:178-83. [PubMed ID: 26619914].
-
9.
Chawla T, Sharma D, Singh A. Role of the renin angiotensin system in diabetic nephropathy. World J. Diabetes. 2010;1:141-5. [PubMed ID: 21537441].
-
10.
Coughlan MT, Thallas-Bonke V, Pete J, Long DM, Gasser A, Tong DC, Arnstein M, Thorpe SR, Cooper ME, Forbes JM. Combination therapy with the advanced glycation end product cross-link breaker, alagebrium, and angiotensin converting enzyme inhibitors in diabetes: synergy or redundancy? Endocrinology. 2007;148:886-95. [PubMed ID: 17110423].
-
11.
de Cavanagh EM, Inserra F, Toblli J, Stella I, Fraga CG, Ferder L. Enalapril attenuates oxidative stress in diabetic rats. Hypertension. 2001;38:1130-6. [PubMed ID: 11711510].
-
12.
Wu L, Parhofer KG. Diabetic dyslipidemia. Metabolism. 2014;63:1469-79. [PubMed ID: 25242435].
-
13.
Tang XL, Sanganalmath SK, Sato H, Bi Q, Hunt G, Vincent RJ, Yong Peng, Shirk G, Dawn B, Bolli R. Atorvastatin therapy during the peri-infarct period attenuates left ventricular dysfunction and remodeling after myocardial infarction. PLoS One. 2011;6:e25320.
-
14.
Abdel-Hamid AA, Firgany AE-DL. Atorvastatin alleviates experimental diabetic cardiomyopathy by suppressing apoptosis and oxidative stress. J. Mol. Histol. 2015;46:337-45. [PubMed ID: 26041576].
-
15.
Inoguchi T, Sonta T, Tsubouchi H, Etoh T, Kakimoto M, Sonoda N, Sato N, Sekiguchi N, Kobayashi K, Sumimoto H, Utsumi H, Nawata H. Protein kinase C–dependent increase in reactive oxygen species (ROS) production in vascular tissues of diabetes: role of vascular NAD (P) H oxidase. J. Am. Soc. Nephrol. 2003;14:S227-32. [PubMed ID: 12874436].
-
16.
Briones AM, Rodríguez-Criado N, Hernanz R, García-Redondo AB, Rodrigues-Díez RR, Alonso MJ, Egido J, Ruiz-Ortega M, Salaices M. Atorvastatin prevents angiotensin II–induced vascular remodeling and oxidative stress. Hypertension. 2009;54:142-9. [PubMed ID: 19451411].
-
17.
Usui H, Shikata K, Matsuda M, Okada S, Ogawa D, Yamashita T, Hida K, Satoh M, Wada J, Makino H. HMG-CoA reductase inhibitor ameliorates diabetic nephropathy by its pleiotropic effects in rats. Nephrol. Dial. Transplant. 2003;18:265-72. [PubMed ID: 12543879].
-
18.
Calvin AD, Aggarwal NR, Murad MH, Shi Q, Elamin MB, Geske JB, Fernandez-Balsells MM, Albuquerque FN, Lampropulos JF, Erwin PJ, Smith SA, Montori VM. Aspirin for the primary prevention of cardiovascular events: a systematic review and meta-analysis comparing patients with and without diabetes. Diabetes Care. 2009;32:2300-6. [PubMed ID: 19741185].
-
19.
Paseban M, Marjaneh RM, Banach M, Riahi MM, Bo S, Sahebkar A. Modulation of microRNAs by aspirin in cardiovascular disease. Trends Cardiovasc. Med. 2020;30:249-54. [PubMed ID: 31444100].
-
20.
American Diabetes Association. Introduction: standards of medical care in diabetes—2020. Diabetes Care. 2020;43:S1-S2. [PubMed ID: 31862741].
-
21.
Ou HC, Lee WJ, Wu CM, Chen JFM, Sheu WHH. Aspirin prevents resistin-induced endothelial dysfunction by modulating AMPK, ROS, and Akt/eNOS signaling. J. Vasc. Surg. 2012;55:1104-15. [PubMed ID: 22244860].
-
22.
Paseban M, Butler AE, Sahebkar A. Mechanisms of statin-induced new-onset diabetes. J. Cell Physiol. 2019;234:12551-61. [PubMed ID: 30618154].
-
23.
Dervisevik M, Dinevska-Kovkarovska S, Dimitrovska M, Cipanovska N, Miova B. High dose of aspirin moderates diabetes-induced changes of heart glycogen/glucose metabolism in rats. J. Physiol. Sci. 2014;64:411-20. [PubMed ID: 25183483].
-
24.
Fullerton MD, Ford RJ, McGregor CP, LeBlond ND, Snider SA, Stypa SA, Day EA, Lhoták S, Schertzer JD, Austin RC, Kemp BE, Steinberg GR. Salicylate improves macrophage cholesterol homeostasis via activation of Ampk. J. Lipid Res. 2015;56:1025-33. [PubMed ID: 25773887].
-
25.
Hundal RS, Petersen KF, Mayerson AB, Randhawa PS, Inzucchi S, Shoelson SE, Shulman GI. Mechanism by which high-dose aspirin improves glucose metabolism in type 2 diabetes. J. Clin. Invest. 2002;109:1321-6. [PubMed ID: 12021247].
-
26.
Paseban M, Mohebbati R, Niazmand S, Sathyapalan T, Sahebkar A. Comparison of the neuroprotective effects of aspirin, atorvastatin, captopril and metformin in diabetes Mellitus. Biomolecules. 2019;9:118.
-
27.
Abbasnezhad A, Niazmand S, Mahmoudabady M, Rezaee SA, Soukhtanloo M, Mosallanejad R, Hayatdavoudi P. Nigella sativa L. seed regulated eNOS, VCAM-1 and LOX-1 genes expression and improved vasoreactivity in aorta of diabetic rat. J. Ethnopharmacol. 2019;228:142-7. [PubMed ID: 30223051].
-
28.
Lee MJ, Park JT, Han SH, Kim YL, Kim YS, Yang CW, Kim NH, Kang SW, Kim HJ, Yoo TH. The atherogenic index of plasma and the risk of mortality in incident dialysis patients: Results from a nationwide prospective cohort in Korea. PLoS One. 2017;12:e0177499. [PubMed ID: 28549070].
-
29.
Sedlak J, Lindsay RH. Estimation of total, protein-bound, and nonprotein sulfhydryl groups in tissue with Ellman’s reagent. Anal. Biochem. 1968;25:192-205. [PubMed ID: 4973948].
-
30.
Mohebbati R, Jalili-Nik M, Paseban M, Shafei MN, Rad AK. Effects of Zataria multiflora extract and Carvacrol on doxorubicin-induced oxidative stress in rat brain. Pharm. Sci. 2018;24:187-92.
-
31.
Uchiyama M, Mihara M. Determination of malonaldehyde precursor in tissues by thiobarbituric acid test. Anal. Biochem. 1978;86:271-8. [PubMed ID: 655387].
-
32.
Pasebasn M, Niazmand S, Soukhtanloo M, Meibodi NT, Abbasnezhad A, Mousavi SM, Niazmand MJ. The therapeutic effect of Nigella sativa seed on indomethacin-induced gastric ulcer in Rats. Curr. Nutr. Food Sci. 2020;16:276-83.
-
33.
Madesh M, Balasubramanian K. Microtiter plate assay for superoxide dismutase using MTT reduction by superoxide. Indian J. Biochem. Biophys. 1998;35:184-8. [PubMed ID: 9803669].
-
34.
Mohebbati R, Paseban M, Soukhtanloo M, Jalili-Nik M, Shafei MN, Yazdi AJ, Rad AK. Effects of standardized Zataria multiflora extract and its major ingredient, Carvacrol, on Adriamycin-induced hepatotoxicity in rat. Biomed. J. 2018;41:340-7. [PubMed ID: 30709575].
-
35.
Aebi H. Catalase in-vitro. Methods Enzymol. 1984;105:121-6. [PubMed ID: 6727660].
-
36.
Mohebbati R, Paseban M, Beheshti F, Soukhtanloo M, Shafei MN, Rakhshandeh H, Rad AK. The preventive effects of standardized extract of zataria multiflora and carvacrol on acetaminophen-induced hepatotoxicity in rat: -zataria multiflora and carvacrol and hepatotoxicity. J. Pharmacopuncture. 2018;21:249-57. [PubMed ID: 30652051].
-
37.
Podhaisky HP, Abate A, Polte T, Oberle S, Schröder H. Aspirin protects endothelial cells from oxidative stress–possible synergism with vitamin E. FEBS Lett. 1997;417:349-51. [PubMed ID: 9409749].
-
38.
Sena CM, Matafome P, Louro T, Nunes E, Fernandes R, Seiça RM. Metformin restores endothelial function in aorta of diabetic rats. Br. J. Pharmacol. 2011;163:424-37. [PubMed ID: 21250975].
-
39.
Yaribeygi H, Faghihi N, Mohammadi MT, Sahebkar A. Effects of atorvastatin on myocardial oxidative and nitrosative stress in diabetic rats. Comp. Clin. Path. 2018;27:691-7.
-
40.
Onozato ML, Tojo A, Goto A, Fujita T, Wilcox CS. Oxidative stress and nitric oxide synthase in rat diabetic nephropathy: effects of ACEI and ARB. Kidney Int. 2002;61:186-94. [PubMed ID: 11786100].
-
41.
Zou MH, Kirkpatrick SS, Davis BJ, Nelson JS, Wiles WG, Schlettner U, Neumann D, Brownlee M, Freeman MB, Goldman MH. Activation of the AMP-activated protein kinase by the anti-diabetic drug metformin in-vivo: role of mitochondrial reactive nitrogen species. J. Biol. Chem. 2004;279:43940-51. [PubMed ID: 15265871].
-
42.
Gallo A, Ceolotto G, Pinton P, Iori E, Murphy E, Rutter GA, Rizzuto R, Semplicini A, Avogaro A. Metformin prevents glucose-induced protein kinase C-β2 activation in human umbilical vein endothelial cells through an antioxidant mechanism. Diabetes. 2005;54:1123-31. [PubMed ID: 15793252].
-
43.
Piwkowska A, Rogacka D, Jankowski M, Dominiczak MH, Stępiński JK, Angielski S. Metformin induces suppression of NAD (P) H oxidase activity in podocytes. Biochem. Biophys. Res. Commun. 2010;393:268-73. [PubMed ID: 20123087].
-
44.
Hou X, Song J, Li XN, Zhang L, Wang X, Chen L, Shen YH. Metformin reduces intracellular reactive oxygen species levels by upregulating expression of the antioxidant thioredoxin via the AMPK-FOXO3 pathway. Biochem. Biophys. Res. Commun. 2010;396:199-205. [PubMed ID: 20398632].
-
45.
Prasad K, Lee P. Suppression of oxidative stress as a mechanism of reduction of hypercholesterolemic atherosclerosis by aspirin. J. Cardiovasc. Pharmacol. Ther. 2003;8:61-9. [PubMed ID: 12652331].
-
46.
Grosser N, Abate A, Oberle S, Vreman HJ, Dennery PA, Becker JC, Pohle T, Seidman DS, Schröder H. Heme oxygenase-1 induction may explain the antioxidant profile of aspirin. Biochem. Biophys. Res. Commun. 2003;308:956-60. [PubMed ID: 12927812].
-
47.
Vecchione C, Gentile M, Aretini A, Marino G, Poulet R, Maffei A, Passarelli F, Landolfi A, Vasta A, G Lembo G. A novel mechanism of action for statins against diabetes-induced oxidative stress. Diabetologia. 2007;50:874-80. [PubMed ID: 17279352].
-
48.
Muchova L, Wong RJ, Hsu M, Morioka I, Vitek L, Zelenka J, Schröder H, Stevenson DK. Statin treatment increases formation of carbon monoxide and bilirubin in mice: a novel mechanism of in-vivo antioxidant protection. Can. J. Physiol. Pharmacol. 2007;85:800-10. [PubMed ID: 17901890].
-
49.
Wassmann S, Laufs U, Müller K, Konkol C, Ahlbory K, Bäumer AT, Linz W, Böhm M, Nickenig G. Cellular antioxidant effects of atorvastatin in-vitro and in-vivo. Arterioscler. Thromb. Vasc. Biol. 2002;22:300-5. [PubMed ID: 11834532].
-
50.
Landmesser U, Drexler H. Oxidative stress, the renin-angiotensin system, and atherosclerosis. Eur. Heart. J. suppl. 2003;5:A3-7.
-
51.
Wulffelé eM, Kooy A, De Zeeuw D, Stehouwer C, Gansevoort R. The effect of metformin on blood pressure, plasma cholesterol and triglycerides in type 2 diabetes mellitus: a systematic review. J. Intern. Med. 2004;256:1-14. [PubMed ID: 15189360].
-
52.
Zhou G, Myers R, Li Y, Chen Y, Shen X, Fenyk-Melody J, Wu M, Ventre J, Doebber T, Fujii N, Musi N, Hirshman MF, Goodyear LJ, Moller DE. Role of AMP-activated protein kinase in mechanism of metformin action. J. Clin. Invest. 2001;108:1167-74. [PubMed ID: 11602624].
-
53.
Malmqvist K, Kahan T, Isaksson H, Östergren J. Regression of left ventricular mass with captopril and metoprolol, and the effects on glucose and lipid metabolism. Blood Press. 2001;10:101-10. [PubMed ID: 11467759].
-
54.
Kawamoto S, Kawamura T, Miyazaki Y, Hosoya T. Effects of atorvastatin on hyperlipidemia in kidney disease patients. Nihon Jinzo Gakkai Shi. 2007;49:41-8. [PubMed ID: 17299985].
-
55.
Ford RJ, Fullerton MD, Pinkosky SL, Day EA, Scott JW, Oakhill JS, Bujak AL, Smith BK, Crane JD, Blumer RM, Marcinko K, Kemp BE, Gerstein HC, Steinberg GR. Metformin and salicylate synergistically activate liver AMPK, inhibit lipogenesis and improve insulin sensitivity. Biochem. J. 2015;468:125–32.
-
56.
Caminhotto RO, Sertié RAL, Andreotti S, Campaãa AB, Lima FB. Renin-angiotensin system blockers regulate the metabolism of isolated fat cells in-vitro. Braz. J. Med. Biol. Res. 2016;49:e5409.
-
57.
De Berardis G, Sacco M, Evangelista V, Filippi A, Giorda CB, Tognoni G, Valentini U, Nicolucci A. Aspirin and simvastatin combination for cardiovascular events prevention trial in diabetes (ACCEPT-D): design of a randomized study of the efficacy of low-dose aspirin in the prevention of cardiovascular events in subjects with diabetes mellitus treated with statins. Trials. 2007;8:21. [PubMed ID: 17725825].
-
58.
Koh KK, Son JW, Ahn JY, Kim DS, Jin DK, Kim HS, Han SH, Seo YH, Chung WJ, Kang WC, Shin EK. Simvastatin combined with ramipril treatment in hypercholesterolemic patients. Hypertension. 2004;44:180-5. [PubMed ID: 15184351].
-
59.
Qin J, Zhang Z, Liu J, Sun L, Hu L, Cooper ME, Cao Z. Effects of the combination of an angiotensin II antagonist with an HMG-CoA reductase inhibitor in experimental diabetes. Kidney Int. 2003;64:565-71. [PubMed ID: 12846751].
-
60.
Tousoulis D, Koniari K, Antoniades C, Miliou A, Noutsou M, Nikolopoulou A, Papageorgiou N, Marinou K, Stefanadi E, Stefanadis C. Impact of 6 weeks of treatment with low-dose metformin and atorvastatin on glucose-induced changes of endothelial function in adults with newly diagnosed type 2 diabetes mellitus: A single-blind study. Clin. Ther. 2010;32:1720-8. [PubMed ID: 21194594].