Abstract
Keywords
Introduction
Old and new approach for determination of cleaning validation acceptance limits:
Cross contamination is one of the highest risks for patients using pharmaceutical products (1-4). Due to the perceived risk, certain classes of medicinal products have been previously required to be manufactured in dedicated or segregated self-contained facilities including, “certain antibiotics, certain hormones, certain cytotoxics ,and certain highly active drugs” (1).
There have been no standardized definitions in terms of what “certain” means and which subset of compounds in each class requires dedicated facilities. Since the new pharmacological and toxicological mechanisms challenge these categories, it is required to modify the historical use of these terms to identify and prioritize compounds requiring special attention (1, 4 and 5).
The technical and organisational deficiencies including insufficient cleaning of equipment, poor facility design, or inappropriate design of the HVAC system are the main reasons for cross contamination (4).
The formerly accepted methods for establishment and calculation of cleaning validation acceptance limits including visually clean, 0.1% dose, and 10-ppm criteria have become obsolete (6). Recent update to the European Union regulation on GMP parts 3 premises and equipment (7) and chapters 5 production (2) show that assessing risk to manage cross contamination and cleaning should be dependent on a toxicological assessment to set threshold, or acceptable daily exposure (ADE) values with the focus on minimizing the risk of cross contamination (1).
According to Paracelsus (1493–1541), the father of toxicology, “all substances are poisons; there is none which is not a poison”. The right dose differentiates poison from a remedy” (8). The acceptable daily exposure approach is inherent in fundamental principles of toxicology. According to the International Society for Pharmaceutical Engineering (ISPE), ADE is “an unlikely dose bringing about a negative effect in an individual upon exposure to, by any route, this or less than this dose daily for a lifespan” (4). According to the European Medicines Agency (EMA), the permitted daily exposure (PDE) is “an unlikely substance-specific dose bringing about a negative effect in an individual upon exposure to this or less than this dose daily for a lifespan” (1). Adverse effect is undesirable and unintended, although not necessarily unexpected result of therapy or other intervention (9, 10).
European Medicines Agency (EMA) guideline and ISPE Risk-MaPP Baseline Guide focus on the use of quality risk management (QRM) on compound-specific Acceptable Daily Exposure to calculate the limits for the maximum carryover by taking into account the toxicological and pharmacological properties of each single product, Occupational Exposure Limit (OEL), and movement away from putting compounds into categories (1, 4, 11 and 12).
Although criteria for Industrial Hygiene (IH) and current Good Manufacturing Practices (cGMP) originate from the same toxicological or clinical data, differences are applied to use these data to set acceptable limits and adequate control strategies.
Differences between IH and cGMP considerations perspectives include worker and product exposure, route of entry, exposure mechanism, and basics of standards for risk assessment (4, 11 and 12). Table 1 summarizes IH and cGMP considerations for risk assessment.
In order to prevent cross contamination, it is necessary to have an overview on the process map and identify IH and cGMP requirements in each process and relevant sub processes. Table 2 shows exposure potential risk assessment based on dustiness, quantity, task duration. Table 3 describes cross contamination risk reduction, considering occupational exposure band (OEB), occupational exposure level (OEL), and containment level (4, 11 and 12).
In order to obtain the appropriate criteria to support cleaning, it is necessary to determine the acceptable level of retention or carryover from one product on a case-by-case basis. The hazard(s) created by the product, the nature and route of administration of the product should be processed in the equipment, unit, or facility. A suitable safety margin, alert and action levels should be incorporated when establishing acceptable levels. Factors for evaluating are feasibility, practicability, cost, and patient, if the cleaning criteria can be met or detected. By dealing with these factors satisfactorily, a multi-product facility could be a viable option to process or make the target material or product (4).
Based on the results of a risk assessment, segregation and dedication may also be required for some compounds to prevent cross contamination during pharmaceutical products manufacturing (4, 13 and 14).
Experimental
Materials and Methods
HPLC grade of Acetonitrile (ACN) was purchased from Romil (UK). Triethylamine, and Trifluoroacetic acid were obtained from Merck (Germany). HPLC grade water was obtained in-house from Puris Expe-UP water purification system (Korea). Gliclazide BPCRS was used as the reference standard.
The Alpha swabs (Texwipe® 761) used for the cleaning assessment were from Texwipe (Philippines). The coupons with surfaces similar to the manufacturing equipment were constructed in house from Stainless Steel, Polyvinyl Chloride (PVC), and Polyethylene (PE) with dimensions of 5 cm × 5 cm. The internal material finish should have an arithmetical average surface roughness of no longer than 0.8 micrometer (15). The Stainless Steel coupons were electro-polished and the average roughness was 0.074 µm. In order to increase reproducibility in the recoveries, to increase the recovery results and to reduce the variability, it is important to regenerate the test surfaces back to the original state. Appropriate cleaning the surface of the coupons is of tremendous importance to perform the quantitative swabbing process successfully. The coupons were ultrasonicated in water, rinsed with purified water, and dried at ambient temperature.
Instrument
Throughout the measurements, a Waters Alliance series HPLC system involving Waters e2695 Separations Module, and UV-VIS detector model 2489 was employed (USA). The system was fitted with a pump, auto-sampler, and Thermostatic Column Compartment (TCC). Empower 3 software was applied for gathering HPLC data and processing. Operating conditions of HPLC in this study are summarized in Table 4.
Standard preparation
Stock standard solution of Gliclazide (0.2 mg/mL) was prepared by weighing approximately 40 mg of Gliclazide BPCRS into a 200 mL volumetric flask, dissolving it in 10 mL of Acetonitrile, and diluting to 200 mL using a mixture of 2 volumes of Acetonitrile and 3 volumes of Water.
Method validation
Method validation requirements depend on the intended use of the method, the status of the method, and the development stage of the product (16-19).
System suitability
Verification of the appropriate performance of the overall system composing of instrument and method at the time of use is regarded as an integral part of the analytical procedure. System suitability test (SST) verifies the actual suitability each time the analytical procedure is applied and can be regarded as a part of continued method performance verification. SST should be designed to detect variation in the performance of a procedure in routine use and it should be based on an understanding of the risk and impact of variation with the acceptance criteria chosen to ensure that the measurement uncertainty remains acceptable (17). SST solution was prepared by dissolving 5 mg of Gliclazide BPCRS and Gliclazide impurity F BPCRS in 25 mL of Acetonitrile, diluting to 50 mL with water and subsequent dilution of 1 volume of the resultant solution to 20 volumes with a mixture of 45 volumes of Acetonitrile and 55 volumes of water (20).
Linearity and Range
Linearity of an analytical procedure is its capability within the target range to yield test results that are directly proportionate to the concentration of analyte in the sample. Range is the span between the higher and lower concentration of analyte in the sample according to which the analytical procedure has an appropriate level of precision, accuracy, and linearity (17-19). Linearity of the method was studied by determining standard solutions at five different concentration levels.
Accuracy
Accuracy of an analytical procedure expresses the closeness of agreement between the value, which is accepted as either a conventional true value or an accepted reference value and the value found. This is sometimes termed “trueness” (18, 19). The uncorrected bias or systematic error in an analysis is measured by accuracy (17). It is necessary to report accuracy as percent recovery by the assay of known added amount of analyte in the sample or as the discrepancy between the mean and the established true value together with the confidence intervals. It is also necessary to assess accuracy using a minimum of 9 determinations over a minimum of 3 concentration levels covering the specified range and 3 replicates each (18). There should be evidence that the samples are accurately recovered. A recovery of >80% is considered good, >50% reasonable, and <50% questionable (21).
Precision
Precision of an analytical procedure is the degree of agreement among individual test results when the procedure is applied repeatedly to multiple samplings of a homogeneous sample. The precision of an analytical procedure is usually expressed as the variance, standard deviation, or coefficient of variation of a series of measurements (18, 19).
Connection between Accuracy and Precision
Precision and accuracy, as the two key components, can be considered together to show if the total error is within the boundary conditions of the material acceptance criteria. Increased precision of a procedure makes any bias inherent in the system more obvious, sheds light on the linearity and range, makes the capability of determining an error brought about by a lack of specificity more obvious. It also enhances the probability of a material meeting the assay acceptance criteria receiving a passing result. Precision and accuracy are the most meticulously connected of these relationships (17).
Specificity
Specificity is the ability to assess the analyte in the presence of components, which may be expected to be present. Typically, these might include impurities, degradation products, and matrix components (18, 19).
LOD and LOQ
The minimum detectable amount of analyte in a sample that cannot be necessarily quantitated under the stated experimental conditions is called Limit of detection (LOD).
Limit of quantitation (LOQ) is the minimum amount of analyte in a sample that can be determined with acceptable precision and accuracy under the stated experimental conditions.
Sample preparation
Defined volume of sample solution at a specific concentration was loaded on 5cm×5cm pre-defined surfaces. The coupons were left to dry at room temperature. A dry swab was pre-wetted with water for wiping the surface of the coupon up to the total 25-cm2 test area.
Four edges of coupon were also wiped. The swab head was cut and put into the premeasured solvent volume in the vial. The process was performed over again by a second swab. Two swabs were put in each vial. The vial was sonicated for five minutes; then, the solution was transferred to an HPLC vial and labeled as sample solution.
Adjusting Health-Based Safety Thresholds/Acceptance Limits
The establishment of acceptance limits is necessary for cleaning the validation programs. Setting the limits requires the right margin of safety. The “Margin of Safety” by definition is the distance of the cleaning data from the acceptance limit. Adjusting the acceptance criteria to a health-based limit such as the ADE leads to several enhancements.
The ADE is derived based on toxicology and not just dosage. The ADE is a properly adjusted “safe level” to clean residues. Operationally, the ADE makes it possible to estimate the true “Margin of Safety” upon the assessment of cleaning residue data. It is made sure that any residuals following cleaning are as low as possible below the health-based criteria, and reducing the risk of cross contamination is possible by assessing the cleaning validation data. It is noted that the ADE is not a “limit” in the true sense. However, it is a reference point to determine the level of “risk” made by the residue data. It is believed that acceptable daily exposure and permitted daily exposure are synonyms, but with the emphasis that specific terms are selected by various regulatory bodies.
It is possible to derive ADE from the following Formula (4):
Where: NOAEL shows the no observed adverse effect level, BW is body weight, UFc shows the composite uncertainty factor, MF shows the modifying factor, PK is the Pharmacokinetic Adjustment(s).
PDE can be derived from the following Equation (1):
Where: NOAEL shows the no observed adverse effect level and F1 to F5 deal with different forms of uncertainty.
Results and Discussion
Method validation
The method was validated for the critical validation parameters (CVPs).
System suitability
The system suitability test is not valid, unless, in the chromatogram obtained, the resolution factor between the peaks due to Gliclazide and Gliclazide impurity F is at least 1.8. The resolution found to be 2.03
Linearity and Range
The result of the standard curve, which was constructed by the least square regression method, is presented in Table 5. Linearity calibration curve is shown in Figure 1, which indicates that the procedure is capable of yielding accurate and precise results at any concentration to measure the target material. The standard stock solution of Gliclazide was diluted to concentration containing 7.43, 10.89, 21.78, 43.56, and 87.12 µg/mL Gliclazide. All solutions were injected in triplicate. Mean found to be 310142, 455426, 906887, 1807240, 3622481 and standard deviations were 2531, 2451, 3452, 3163, and 7330, respectively.
Accuracy
Accuracy test results revealed that the recovery of reference material covering the specified range including 50, 100, and 200% concentration levels, which were spiked to 5 cm × 5 cm coupons, were in accordance to the acceptable criteria. As shown in Table 6, the proposed method was highly accurate for the quantitative analysis. Figure 2 illustrates chromatograms of system suitability, standard, and recovery studies at 100% concentration level of Gliclazide swab sampling from PVC, Stainless Steel, and Polyethylene.
Precision
Precision was determined by assaying a homogeneous sample using nine determinations covering the specified range of the procedure for each pre-defined surface. Table 7 indicates acceptable precision, R.S.D. lower than 15%, across the entire dataset.
Specificity
Specificity was performed by spiking product with appropriate level of excipients. Specificity test results proved that all excipients could be separated completely.
LOD and LOQ
The LODs (S/N = 3) and LOQs (S/N = 10) were found to be 2.23 µg/mL and 7.43 µg/mL, respectively, based on the analytical method validation of assay.
For mitigating the risk of cross contamination based on the failure mode and effect analysis (FMEA), more restrictive acceptance limit through adding safety factors to cleaning validation acceptance criteria was considered as preventive control. Obtained results showed that this approach only leads to failed results and costly measures in production operations. The better developed cleaning process, focusing on decreasing the remained residue in production instead of adding safety factors to acceptance limits leads to the main goal, which is patient safety.
The initial attempts for recovery studies revealed low and changeable recoveries from coupon surfaces. Ishikawa diagram was used to break down root causes that potentially contribute to failure in recovery test results as a defect. Method modification was studied for obtaining consistent and high recovery. Critical parameters were swabbing, spiking, and extraction technique of the API from swab. None of the parameters eliminated the previously observed variability. The major contributor to low and variable recoveries from coupons was traced to the lack of standard operating procedure for cleaning the coupon surfaces.
Material investigation was done to study if the coupon was a source of inconsistency. Roughness test was performed on both the coupons and drums. In order to improve the resistance of the stainless-steel material and prevent corrosion and improper cleaning of the surface of the coupons in recovery studies and production equipment, mechanical and electro-polishing were employed on the surfaces which were not meeting roughness acceptance criteria.
Variable recoveries from stainless steel coupons in different spiking levels including 50, 100, and 200% were studied. It was observed that spiking at the lower concentration levels displayed not acceptable recoveries due to experimental errors on recovering lower mass in comparison to the larger mass of the drug. The precision results in Table 7, with R.S.D. lower than 15% indicates that preventive and detection controls, based on the FMEA, including analyst training, following standard operating procedure, and monitoring the process were effective.
Sampling and dispensing tools with very low risk of cross-contamination were considered disposable and dedicated for the worst cases based on the result of the risk assessment. Although the cleaning validation results were within the limit leading to extra costs to have added safety to the patient, they were unjustifiable by measurable means.
Assessment of the worst case of the cleaning validation was done based on solubility, potency, toxicity (LD50), ADE, PDE, improvement of LOD of the method through analytical method validation, and consideration of the dosage form. The higher risk of cross-contamination of uncoated tablets compared to coated tablets is a quantitative factor to increase the margin of safety.
Cleaning acceptance criteria based on the MAC approach and 10 ppm were 16390 and 672 mg, respectively. Ten ppm was utilized as the worst case to establish the limit. The highest dosage of Gliclazide was defined as the worst case. Gliclazide 80 mg was selected as the worst case among Gliclazide 80 mg, 60 mg, and 30 mg.
Standards for risk assessment, Industrial Hygiene and cGMP considerations (8).
Industrial Hygiene (IH) | Quality (cGMP) |
---|---|
Occupational Exposure Limit (OEL) expressed by an airborne concentration (mass per cubic meter of air) to address primary route of entry for exposure: Inhalation | Acceptable Daily Exposure (ADE) expressed as mg/day |
Cleaning Limit expressed as mg/swab or mg/L to address primary route of exposure: Ingestion, 4 |
Defining factor of the quantity of loose powder transfer considering dustiness potential, quantity handled, and task duration in exposure potential risk assessment (4, 11 and 12)
Factor | Level | ||
---|---|---|---|
Dustiness potential | Low | Medium | High |
Quantity handled | Small | Medium | High |
Task duration | Short | Medium | Long |
Reducing the risk of cross-contamination by airborne or mechanical transfer routes considering occupational exposure band (OEB), occupational exposure level (OEL), and containment level (4, 11 and 12).
Occupational exposure band (OEB) | Occupational exposure level (OEL) | Containment level |
---|---|---|
5 | < 1 µg/m3 | High |
4 | 1 to 10 µg/m3 | |
3 | 10 to 100 µg/m3 | |
2 | 100 to 1000 µg/m3 | |
1 | 1000-5000 µg/m3 | Low |
Chromatographic conditions
Column | Zorbax Eclipse |
C8, Octylsilyl silica gel | |
250 mm × 4.6 mm, 5 µm | |
Mobile phase | 0.1 volume of Triethylamine |
0.1 volume of Trifluroacetic acid | |
45 volumes of Acetonitrile | |
55 volumes of Water | |
Flow rate | 0.9 mL/min |
Injection volume | 20 µL |
Detector UV-VIS | 235 nm |
Column temperature | Ambient |
Statistical data of calibration curve of Assay
Analyte (Gliclazide) | |
---|---|
Regression equation | y = 41546.36255x + 1377.780656 |
Linearity range | 7.43-87.12 (µg/mL) |
y-intercept | 1377.780656 |
Slope | 41546.36255 |
Correlation coefficient | 0.999997 |
Accuracy by recovery of the chromatographic method
Material substance of product contact surfaces | Sampling Method | Concentration Levels | ||
---|---|---|---|---|
50 (%) | 100 (%) | 200 (%) | ||
Stainless Steel | Swab | 95.30 | 84.84 | 81.20 |
Polyvinyl Chloride (PVC) | Swab | 88.21 | 92.50 | 73.65 |
Polyethylene (PE) | Swab | 69.56 | 72.52 | 74.93 |
Precision by recovery of the chromatographic method
Material substance of product contact surfaces | Sampling Method | Concentration Levels | ||
---|---|---|---|---|
50 (%) | 100 (%) | 200 (%) | ||
Stainless Steel | Swab | 3.66 | 4.02 | 7.26 |
Polyvinyl Chloride (PVC) | Swab | 1.94 | 6.69 | 3.85 |
Polyethylene (PE) | Swab | 5.15 | 9.94 | 5.47 |
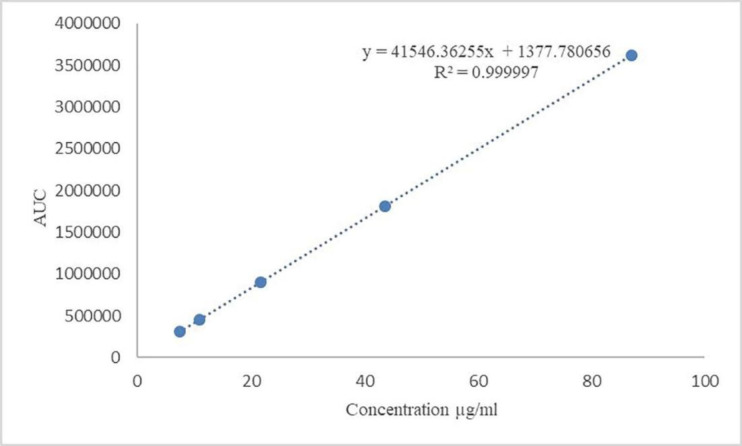
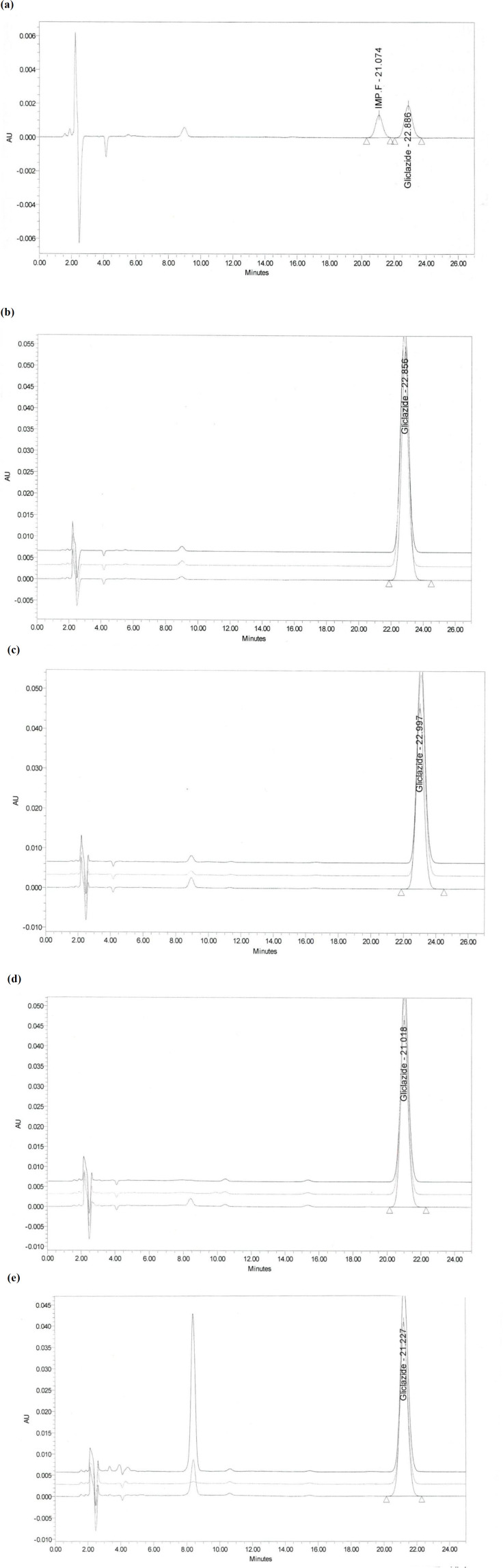
Conclusion
This study demonstrates that the cleaning method is validated for specificity, limit of detection, limit of quantitation, precision, accuracy, linearity, and range. Risk assessment for Industrial Hygiene (IH) and cGMP was done science based criteria were defined for making decision to manage the risk to the patients and worker protection.
Acknowledgements
References
-
1.
European Medicines Agency. EMA Guideline on Setting Health Based Exposure Limits for Use in Risk Identification in the Manufacture of Different Medicinal Products in Shared Facilities. 2014:1-11.
-
2.
European commission. EU Guidelines for Good Manufacturing Practice for Medicinal Products for Human and Veterinary Use. 2014:1-12.
-
3.
Parenteral Drug Association (PDA). Points to Consider for Cleaning Validation, Technical Report No. 29. 2012:29-42.
-
4.
International Society for Pharmaceutical Engineering (ISPE). Baseline Pharmaceutical Engineering Guide, Risk-Based Manufacture of Pharmaceutical Products: A Guide to Managing Risks Associated with Cross Contamination. 2010:7-128.
-
5.
National Institute for Occupational Safety. List of Antineoplastic and Other Hazardous Drugs in Healthcare Settings, Department of Health and Human Services Centers for Disease Control and Prevention. 2016. p. 1-34.
-
6.
LeBlanc DA. Validated Cleaning Technologies for Pharmaceutical Manufacturing. Interpharm/CRC. 2000. p. 135-167.
-
7.
European commission. EU Guidelines for Good Manufacturing Practice for Medicinal Products for Human and Veterinary Use. Volume 4, Part 1 Chapter 3: Premises and Equipment. 2014. p. 1-5.
-
8.
Casarett and Doull’s Toxicology, The Basic Science of Poisons. McGraw-Hill Professional. 7th ed. 2007. p. 3-9.
-
9.
International Society for Pharmaceutical Engineering (ISPE). Oral Solid Dosage Forms, Volume 2, 3rd ed. 2016. p. 77-83.
-
10.
Guideline on good pharmacovigilance practices (GVP). European Medicines Agency EMA. 2014:5-14.
-
11.
Ter Burg W, Muller JJA, Jongeneel WP. Risks of systemic effects after dermal exposure for workers, Part A: Proposed approaches for risk evaluation. National Institute for Public Health and Environment. 2011:7-50.
-
12.
Methodology for the Derivation of Occupational Exposure Limits. Scientific Committee on Occupational Exposure Limits (SCOEL). 2013:2-38.
-
13.
ICH Q9. Harmonised Tripartite Guideline, Quality risk management. 2005:1-19.
-
14.
Chrysler LLC. Ford Motor Company, General Motors Corporation. Potential Failure Mode and Effect Analysis (FMEA). 4th ed. 2008. p. 67-112.
-
15.
World Health Organization (WHO). Good Manufacturing Practices: water for pharmaceutical use, WHO Technical Report Series No. 970, Annex 2. 2012. p. 78-81.
-
16.
Shokraneh F, Asgharian R, Abdollahpour A, Ramin M, Montaseri A, Mahboubi A. A novel high performance liquid chromatographic method for determination of nystatin in pharmaceutical formulations by Box–Behnken statistical experiment design. Iran. J. Pharm. Res. 2015;14:43-9. [PubMed ID: 26185504].
-
17.
Ermer J, Nethercote P. Method Validation in Pharmaceutical Analysis. 2nd ed., Wiley. 2012:220-95.
-
18.
ICH Q2 (R1). Harmonised Tripartite Guideline, Validation of Analytical Procedures, Text and Methodology. 2005. p. 1-13.
-
19.
The United States Pharmacopeial Convention. USP 39, National Formulary (NF) 34, Chapter <1225> Validation of Compendial Procedures. 2016. p. 1640-5.
-
20.
British Pharmacopoeia. 2017;Volume 3:653-4.
-
21.
World Health Organization (WHO). Quality assurance of pharmaceuticals, A compendium of guidelines and related materials, Volume 2, 2nd ed., Good manufacturing practices and inspection. 2007. p. 120-33.