Abstract
Keywords
Introduction
Acquired immunodeficiency syndrome (AIDS), induced by human immunodeficiency virus (HIV), remains a devastating infectious disease all over the world. It has been estimated that approximately 36.7 million people are living with HIV-1 (1). During the past two decades, over 30 FDA-approved anti-HIV drugs have been developed with different mechanisms, such as targeting viral diffusion, reverse transcription, DNA polymerization, and integration (2). In recent years, progressions of HIV-1 infection have been managed by highly active antiretroviral therapy (HAART), in which a combination of various anti-HIV drugs is used (3). Integrase (IN), one of the critical enzymes in the virus life cycle, catalyzes an important process in viral replication. It inserts the product of the reverse transcription, viral double strand DNA (dsDNA), within the human genome. The integration step is composed of two reactions called 3′-processing (3′-P) and strand transfer (ST) that both of them are mediated by HIV-1 IN enzyme. The (3′-P) reaction is carried out in the host cell′s cytoplasm, in which IN hydrolyses a phosphodiester bond and removes a conserved GT dinucleotide from the 3′-end of the viral DNA releasing two free 3′-OH groups as nucleophiles. The package of reverse transcripted viral DNA and IN, preintegration complex (PIC), transfers into the nucleus where the next step, strand transfer, occurs. During the ST reaction, IN enzyme catalyzes the joining of virus DNA to the host DNA (4-8).
IN has been considered as an appropriate target for the development of antiretroviral agents (9-11). To date, four HIV-1 INST inhibitors (INSTIs) have been developed that selectively suppress the ST reaction (12): The first generation Elvitegravir (1) and Raltegravir (2), and the second generation Dolutegravir (3) and Bictegravir (4) (Figure 1) (13-19). These approved integrase inhibitors fit at the IN/DNA interface tightly and inhibit the HIV IN by binding to the Mg2+ ions present in the active site (20, 21). Resistance of HIV strain to the first generation is confirmed (22, 23); therefore, recently, many efforts have been made to develop a new generation of INSTIs to overcome the resistant mutant. Cabotegravir (5) is another generation integrase strand transfer inhibitor under clinical investigation (24, 25).
Beta-Diketo acid (DKA) derivatives were identified as the first selective inhibitors of the IN strand transfer process. This compound class is characterized by a 4-oxo-2-butenoic acid moiety that is capable of coordinating Mg2+ ions and a flexible halobenzyl portion that interacts with the hydrophobic pocket present in the enzyme active site. Among DKA derivatives, indolyl and pyrrolyl diketo acids (exemplified by compounds CHI-1043 (6) and L-731,988 (7), respectively) exhibited submicromolar inhibitory activity in both enzymatic and cellular assays with low cell toxicity (26-30). Considering the above facts, and as part of our studies on IN inhibitors, we designed a novel series of DKA derivatives by replacing the central pyrrolyl or indolyl rings with the bicyclic benzimidazolyl platform (Figure 2). Different substituted benzyl groups were examined at the N-1 position of benzimidazolyl ring because the nature and pattern of substitution significantly affect the IN inhibitory activity (27, 28).
Experimental
General
All materials and solvents applied in this study were prepared from Merck AG and Aldrich Chemical. Melting points were determined with a Thomas–Hoover capillary apparatus. Infrared spectra were recorded using a Perkin Elmer Model 1420 spectrometer. 1HNMR and 13CNMR spectra were acquired on a Bruker FT-400 MHz instrument (Brucker Biosciences, USA). DMSO-d6 was used as a deuterated solvent. A 6410 Agilent LCMS triple quadrupole mass spectrometer (LCMS) with an electrospray ionization (ESI) interface was used to acquire the mass spectra. The standard methods were used to dry the organic solvents. Analytical results were determined within ± 0.4% of theoretical values. C, H, and N elemental analysis were performed on a Costech 4010 elemental analyzer.
Synthesis of compounds 1-(1H-benzo[d]imidazol-2-yl)ethan-1-ol (10) and 1-(1H-benzo[d]imidazol-2-yl)ethan-1-one (11)
Compounds 10 and 11 were synthesized according to the procedures described in the literature (31). Melting point and spectroscopic data were compared with the literature for confirmation.
General Procedure for the Synthesis of 12a-i
To a solution of 1-(1H-benzo[d]imidazol-2-yl)ethan-1-one 11 (3 mmol) in DMF at room temperature was added anhydrous K2CO3 (6 mmol). After stirring for 5 minutes, corresponding benzyl chlorides (3.3 mmol) were added drop wisely. The reaction mixture was stirred for 12 hours at room temperature. After completion, the mixture was poured into the ice water. Then the obtained precipitate was collected, filtered, and dried to give compounds 12a-i.
1-(1-benzyl-1H-benzo[d]imidazol-2-yl) ethan-1-one (12a)
Yield 98%; orange oil; IR (CHCl3) υ (cm-1) 1685 (C=O ketone), LC-MS (ESI) m/z 251 (M+H+).
1-(1-(2-methylbenzyl)-1H-benzo[d]imidazol-2-yl)ethan-1-one (12b)
Yield 98%; orange oil; IR (CHCl3) υ (cm-1) 1695 (C=O ketone), LC-MS (ESI) m/z 265 (M+H+).
1-(1-(3-methylbenzyl)-1H-benzo[d]imidazol-2-yl)ethan-1-one (12c)
Yield 98%; orange oil; IR (CHCl3) υ (cm-1) 1686 (C=O ketone), LC-MS (ESI) m/z 265 (M+H+).
1-(1-(4-methylbenzyl)-1H-benzo[d]imidazol-2-yl)ethan-1-one (12d)
Yield 98%; orange oil; IR (CHCl3) υ (cm-1) 1674 (C=O ketone), LC-MS (ESI) m/z 265 (M+H+).
1-(1-(2-chlorobenzyl)-1H-benzo[d]imidazol-2-yl)ethan-1-one (12e)
Yield 98%; orange oil; IR (CHCl3) υ (cm-1) 1677 (C=O ketone), LC-MS (ESI) m/z 285 (M+H+).
1-(1-(3-chlorobenzyl)-1H-benzo[d]imidazol-2-yl)ethan-1-one (12f)
Yield 98%; orange oil; IR (CHCl3) υ (cm-1) 1686 (C=O ketone), LC-MS (ESI) m/z 285 (M+H+).
1-(1-(2-fluorobenzyl)-1H-benzo[d]imidazol-2-yl)ethan-1-one (12g)
Yield 98%; orange oil; IR (CHCl3) υ (cm-1) 1688 (C=O ketone), LC-MS (ESI) m/z 269 (M+H+).
1-(1-(3-fluorobenzyl)-1H-benzo[d]imidazol-2-yl)ethan-1-one (12h)
Yield 98%; orange oil; IR (CHCl3) υ (cm-1) 1683 (C=O ketone), LC-MS (ESI) m/z 269 (M+H+).
1-(1-(3-methoxybenzyl)-1H-benzo[d]imidazol-2-yl)ethan-1-one (12i)
Yield 98%; orange oil; IR (CHCl3) υ (cm-1) 1687 (C=O ketone), LC-MS (ESI) m/z 281 (M+H+).
General Procedure for the Synthesis of 13a-i
To a stirring suspension of 12a-i derivatives (2 mmol) in 10 mL dry diethyl ether at −10 °C was added diethyl oxalate (2.4 mmol). After stirring under an argon atmosphere for 10 min at −10 °C, a solution of sodium ethoxide (3 mmol) in 5 mL anhydrous absolute ethanol was added dropwisely. The reaction mixture was stirred at room temperature for 2 h. Then the organic phase was evaporated under reduced pressure, the obtained residue was dissolved in water. After acidification of the solution with 6M HCl solution to pH 2, the precipitates of DKA derivatives 13a-i were yielded. The obtained solid was washed with water and methanol to afford pure 13a-i products.
4-(1-benzyl-1H-benzo[d]imidazol-2-yl)-2-hydroxy-4-oxo-2-butenoic acid (13a)
Yield 50-60%; 127 °C. IR (KBr) υ (cm-1) 3414 (OH), 1722 (C=O acid), 1617 (C=O ketone). 1H NMR (DMSO-d6) δ 5.99 (s, 2H, N-CH2), 7.04 (br s, 1H, butanoate C3-H), 7.18-7.30 (m, 5H, benzyl H), 7.38-7.42 (t, 2H, J = 8 Hz, benzimidazol C5-H & C6-H), 7.70 (d, 1H, J = 6.8 Hz, benzimidazol C7-H), 7.87 (d, 1H, J = 6.4 Hz, benzimidazol C4-H); 13C-NMR (DMSO-d6) δ 48.58, 112.64, 121.38, 124.87, 126.91, 127.16, 127.98, 128.12, 129.13, 136.77, 137.40, 140.58, 145.85, 161.47, 162.06, 164.28; LC-MS (ESI) m/z 321 [M-H]-; Anal. (C18H14N2O4) C, 67.08; H, 4.38; N, 8.69; Found: C, 67.22; H, 4.15; N, 8.90.
4-(1-(2-methylbenzyl)-1H-benzo[d]imidazol-2-yl)-2-hydroxy-4-oxo-2-butenoic acid (13b)
Yield 50-60%; 142 °C. IR (KBr) υ (cm-1) 3460 (OH), 1740 (C=O acid), 1621 (C=O ketone). 1H NMR (DMSO-d6) δ 2.44 (s, 3H, CH3), 5.94 (s, 2H, N-CH2), 6.09 (d, 1H, J = 7.6 Hz, 2-methylbenzyl C6-H), 6.94-6.98 (t, 1H, J = 7.2 Hz, 2-methylbenzyl C4-H), 7.11-7.15 (t, 1H, J = 7.2 Hz, 2- methylbenzyl C5-H), 7.22 (s, 1H, butanoate C3-H), 7.25 (d, 1H, J = 7.2 Hz, 2- methylbenzyl C3-H), 7.45-7.46 (t, 2H, benzimidazol C5-H & C6-H), 7.62 (d, 1H, J = 6.8 Hz, benzimidazol C7-H), 7.97 (d, 1H, J = 7.2 Hz, benzimidazol C4-H), 14.07 (br s, 1H, COOH); 13C-NMR (DMSO-d6) δ 19.22, 46.91, 102.89, 112.52, 121.48, 124.05, 124.94, 126.63, 127.00, 127.38, 130.61, 135.24, 135.67, 137.06, 140.59, 146.14, 164.25, 184.08, 189.74; LC-MS (ESI) m/z 335 [M-H]-; Anal. (C19H16N2O4) C, 67.85; H, 4.80; N, 8.33; Found: C, 67.99; H, 4.65; N, 8.10.
4-(1-(3-methylbenzyl)-1H-benzo[d]imidazol-2-yl)-2-hydroxy-4-oxo-2-butenoic acid (13c)
Yield 50-60%; 133 °C. IR (KBr) υ (cm-1) 3444 (OH), 1725 (C=O acid), 1621 (C=O ketone). 1H NMR (DMSO-d6) δ 2.23 (s, 3H, CH3), 5.96 (s, 2H, N-CH2), 6.93-7.20 (m, 5H, 3-methylbenzyl H and butanoate C3-H), 7.44 (s, 2H, benzimidazol C5-H & C6-H), 7.72 (s, 1H, benzimidazol C7-H), 7.92 (m, 1H, benzimidazol C4-H); 13C-NMR (DMSO-d6) δ 21.47, 48.55, 112.64, 121.34, 121.88, 124.17, 124.35, 124.81, 126.82, 127.69, 128.65, 129.04, 136.79, 137.37, 138.30, 140.59, 145.98, 164.38; LC-MS (ESI) m/z 335 [M-H]-; Anal. (C19H16N2O4) C, 67.85; H, 4.80; N, 8.33; Found: C, 67.60; H, 4.91; N, 8.03.
4-(1-(4-methylbenzyl)-1H-benzo[d]imidazol-2-yl)-2-hydroxy-4-oxo-2-butenoic acid (13d)
Yield 50-60%; 146 °C. IR (KBr) υ (cm-1) 3417 (OH), 1729 (C=O acid), 1617 (C=O ketone). 1H NMR (DMSO-d6) δ 2.23 (s, 3H, CH3), 5.94 (s, 2H, N-CH2), 7.09 (m, 4H, 4-methylbenzyl H), 7.20 (s, 1H, butanoate C3-H), 7.40-7.43 (t, 1H, J = 6.8, benzimidazol C6-H), 7.45-7.49 (t, 1H, J = 7.6, benzimidazol C5-H), 7.75 (d, 1H, J = 8, benzimidazol C7-H), 7.91 (d, 1H, J = 8, benzimidazol C4-H) What about OH groups?; 13C-NMR (DMSO-d6) δ 21.09, 48.07, 112.49, 121.82, 121.86, 124.14, 126.40, 127.23, 127.34, 129.60, 137.12, 141.57, 164.25; LC-MS (ESI) m/z 335 [M-H]-; Anal. (C19H16N2O4) C, 67.85; H, 4.80; N, 8.33; Found: C, 68.05; H, 4.94; N, 8.10.
4-(1-(2-chlorobenzyl)-1H-benzo[d]imidazol-2-yl)-2-hydroxy-4-oxo-2-butenoic acid (13e)
Yield 50-60%; 137 °C. IR (KBr) υ (cm-1) 3414 (OH), 1733 (C=O acid), 1617 (C=O ketone). 1H NMR (DMSO-d6) δ 6.02 (s, 2H, N-CH2), 6.36 (d, 1H, J = 7.6 Hz, 2-chlorobenzyl C6-H), 7.13-7.17 (t, 1H, J = 7.6 Hz, 2-chlorobenzyl C5-H), 7.22 (s, 1H, butanoate C3-H), 7.28-7.32 (t, 1H, J = 7.6 Hz, 2-chlorobenzyl C4-H), 7.43-7.49 (m, 2H, benzimidazol C5-H & C6-H), 7.55 (d, 1H, J = 8, 2-chlorobenzyl C3-H), 7.64 (d, 1H, J = 7.4 Hz, benzimidazol C7-H), 7.96 (d, 1H, J = 7.6, benzimidazol C4-H); 13C-NMR (DMSO-d6) δ 46.89, 112.24, 121.59, 123.91, 124.97, 126.78, 127.09, 128.12, 129.44, 129.96, 131.73, 134.89, 136.90, 140.73, 146.23, 164.22; LC-MS (ESI) m/z 355 [M-H]-; Anal. (C18H13ClN2O4) C, 60.60; H, 3.67; N, 7.85; Found: C, 68.02; H, 4.70; N, 8.10.
4-(1-(3-chlorobenzyl)-1H-benzo[d]imidazol-2-yl)-2-hydroxy-4-oxo-2-butenoic acid (13f)
Yield 50-60%; 149 °C. IR (KBr) υ (cm-1) 3440 (OH), 1729 (C=O acid), 1617 (C=O ketone). 1H NMR (DMSO-d6) δ 6.00 (s, 2H, N-CH2), 7.10 (br s, 1H, 3-chlorobenzyl C6-H), 7.23 (s, 1H, butanoate C3-H), 7.33-7.34 (m, 3H, 3-chlorobenzyl C2-H & C4-H & C5-H), 7.42-7.46 (t, 1H, J = 7.2, benzimidazol C6-H), 7.48-7.51 (t, 1H, J = 7.6, benzimidazol C5-H), 7.77 (d, 1H, J = 8, benzimidazol C7-H), 7.94 (d, 1H, J = 8, benzimidazol C4-H); 13C-NMR (DMSO-d6) δ 48.06, 112.48, 121.47, 124.96, 125.75, 125.88, 127.10, 128.00, 131.07, 133.74, 136.69, 139.93, 140.57, 145.86, 164.30, 184.03; LC-MS (ESI) m/z 355 [M-H]-; Anal. (C18H13ClN2O4) C, 60.60; H, 3.67; N, 7.85; Found: 60.52; H, 3.50; N, 7.55.
4-(1-(2-fluorobenzyl)-1H-benzo[d]imidazol-2-yl)-2-hydroxy-4-oxo-2-butenoic acid (13g)
Yield 50-60%; 135 °C. IR (KBr) υ (cm-1) 3414 (OH), 1725 (C=O acid), 1621 (C=O ketone). 1H NMR (DMSO-d6) δ 6.05 (s, 2H, N-CH2), ), 6.75-6.79 (t, 1H, J = 7.6 Hz, 2-fluorobenzyl C5-H), 7.05-7.08 (t, 1H, J = 7.6 Hz, 2-fluorobenzyl C4-H), 7.23 (s, 1H, butanoate C3-H), 7.25-7.33 (m, 2H, 2-fluorobenzyl C3-H & C6-H), 7.42-7.46 (t, 1H, J = 7.2, benzimidazol C6-H), 7.47-7.51 (t, 1H, J = 8, benzimidazol C5-H), 7.73 (d, 1H, J = 8.4 Hz, benzimidazol C7-H), 7.94 (d, 1H, J = 7.6, benzimidazol C4-H); 13C-NMR (DMSO-d6) δ 43.16, 43.20, 112.34, 115.82, 116.03, 121.53, 124.37, 124.52, 124.93, 125.20, 125.23, 127.04, 128.32, 128.36, 129.97, 130.05, 136.88, 140.61, 145.97, 158.91, 161.34, 164.22, 184.26; LC-MS (ESI) m/z 339 [M-H]-; Anal. (C18H13FN2O4) C, 63.53; H, 3.85; N, 8.23; Found: C, 63.38; H, 3.61; N, 8.45.
4-(1-(3-fluorobenzyl)-1H-benzo[d]imidazol-2-yl)-2-hydroxy-4-oxo-2-butenoic acid (13h)
Yield 50-60%; 144 °C. IR (KBr) υ (cm-1) 3406 (OH), 1725 (C=O acid), 1613 (C=O ketone). 1H NMR (DMSO-d6) δ 6.00 (s, 2H, N-CH2), 6.98 (d, 1H, 3-fluorobenzyl C6-H), 7.07-7.12 (m, 2H, 3-fluorobenzyl C2-H & C4-H), 7.22 (s, 1H, butanoate C3-H), 7.32-7.38 (m, 1H, 3-fluorobenzyl C5-H), 7.41-7.45 (t, 1H, J = 7.6 Hz, benzimidazol C6-H), 7.47-7.51 (t, 1H, J = 8, benzimidazol C5-H), 7.76 (d, 1H, J = 8.4 Hz, benzimidazol C7-H), 7.93 (d, 1H, J = 8, benzimidazol C4-H); 13C-NMR (DMSO-d6) δ 48.13, 112.50, 114.08, 114.30, 114.74, 114.94, 121.44, 123.10, 123.12, 124.93, 126.99, 131.16, 131.24, 136.70, 140.24, 140.31, 140.59, 145.88, 161.47, 162.06, 163.89, 164.29; LC-MS (ESI) m/z 339 [M-H]-; Anal. (C18H13FN2O4) C, 63.53; H, 3.85; N, 8.23; Found: C, 63.66; H, 3.62; N, 8.50.
4-(1-(3-methoxybenzyl)-1H-benzo[d]imidazol-2-yl)-2-hydroxy-4-oxo-2-butenoic acid (13i)
Yield 50-60%; 130 °C. IR (KBr) υ (cm-1) 3414 (OH), 1729 (C=O acid), 1610 (C=O ketone). 1H NMR (DMSO-d6) δ 3.69 (s, 3H, OCH3), 5.96 (s, 2H, N-CH2), 6.67 (d, 1H, J = 7.6 Hz, 3-methoxybenzyl C6-H), 6.80-6.83 (m, 3H, methoxybenzyl C2-H & C4-H & C5-H), 7.21 (s, 1H, butanoate C3-H), 7.40-7.44 (t, 1H, J = 7.2 Hz, benzimidazol C6-H), 7.46-7.49 (t, 1H, J = 7.6 Hz, benzimidazol C5-H), 7.75 (d, 1H, J = 8, benzimidazol C7-H), 7.92 (d, 1H, J = 8, benzimidazol C4-H); 13C-NMR (DMSO-d6) δ 48.47, 55.44, 112.65, 112.96, 113.32, 119.09, 121.37, 124.87, 126.90, 130.31, 136.77, 138.95, 140.54, 145.87, 159.84, 164.29; LC-MS (ESI) m/z 351 [M-H]-; Anal. (C19H16N2O5) C, 64.77; H, 4.58; N, 7.95; Found: C, 64.53; H, 4.70; N, 8.12.
In-vitro anti-HIV and cytotoxicity assays
Anti-HIV-1 activity of synthesized compounds was determined by single cycle replication assay which was reported previously (32-34). We constructed plasmid containing the HIV-1 genome mutated in the pol gene, which was co-transfected with plasmids expressing the pol gene products reverse transcriptase (RT) and integrase (IN), and the glycoprotein G of vesicular stomatitis virus. The virions produced in HEK 293 T cells were antigenic, but able to replicate only for one cycle, e.g. first generation single-cycle replicable (SCR) virions. The compounds were dissolved in DMSO at different concentrations of 10 mmol/L to 10 μmol/L. These stocks were diluted 100 times in cell environment so that the final concentrations of compounds were 100 μmol/L to 10 nmol/L. Zidovudine (AZT) and DMSO (1% v/v) were used as positive and negative controls. All tests were performed in triplicate. In the presence of different concentrations of compounds, HeLa cells (6 × 103 per well of 96-wells plate) were infected with single cycle replicable HIV NL4-3 virions (200 ng p24). The compounds were added to the cells’ environment simultaneously with viral infection. After 72 h of infection, the supernatants of cell culture were collected, and p24 antigen load was measured by capture ELISA (Biomerieux, France). Percentage inhibition of p24 expression in treated culture was calculated as inhibition rate of p24 (%). XTT proliferation method was performed to evaluate the cellular toxicity of compounds. XTT (sodium 3-[1 (phenyl aminocarbonyl)-3,4-tetrazolium]-bis(4-methoxy-6-nitro) benzene sulfonic acid) reagent was used according to the kit instruction (Roche, Germany). The cells were cultured in 96 well plates (3.5 × 104 cells/well) containing fresh phenol-red free medium and incubated for 72 h in a CO2 incubator. Subsequently, 50 μL of XTT was added into each well and incubated 4 h at 37 °C. The plates were evaluated by ELISA reader at the test and reference OD of 450 and 630 nm, respectively. The cytotoxic concentration that reduced number of viable cells by 50% (CC50) was calculated after determining p24 load in the HIV-1 replication assay plates.
Molecular modeling studies
Molecular modeling was performed using the Autodock Vina (35). 3OYA was used for binding mode analysis of HIV-2.54 cm inhibitory activity. The protein and ligands were prepared in Autodock tools 1.5.6 from MGL Tools package (36). The co-crystallized ligand and water molecules were extracted, Kollman charges were added, nonpolar hydrogens were merged, and AutoDock4 atom type was assigned to the protein structure. The ligand was created and minimized using HyperChem 8.0 (37). The active site was defined as a Grid box around the crystallographic ligand Raltegravir in 20 × 20 × 20 dimensions. Selected molecules were docked in the active site, and the bioactive conformations were generated using Autodock Vina.
Results and Discussion
Chemistry
The preparation procedure of final benzimidazolyl DKA derivatives was shown in Scheme 1. 2-(α-hydroxyethyl)benzimidazole 10 was achieved via Philips condensation of commercially available o-phenylendiamine (8) and lactic acid (9) in 4N HCl (38). Then 10 was oxidized in the presence of K2Cr2O7 in dil. H2SO4 and the mixture was neutralized by aq.NH3 to pH of 5.5-6.0 to afford 2-acetylbenzimidazole 11 intermediates (31). N-benzylation of 11 with substituted benzyl chlorides proceeded to yield 12a-i derivatives (39), which were then condensed with diethyl oxalate using sodium ethoxide in diethyl ether and salt-ice bath to synthesis the corresponding DKA compounds 13a-i (40).
Anti-HIV-1 activities
The novel benzimidazolyl DKA derivatives containing different substituted benzyl derivatives at N-1 position was synthesized and tested in-vitro for the inhibition of the single-cycle HIV-1 replication in HeLa cell culture. Raltegravir was used as a positive control. The synthesized compounds were also evaluated for cytotoxicity in a cell-based XTT assay. The biological results were expressed as anti-HIV-1 EC50, CC50, and SI (selectivity index, given by the CC50/EC50 ratio). The results of the biological activities of the synthesized compounds were summarized in Table 1. All newly synthesized compounds had a safety profile with no significant cytotoxicity (CC50 values > 380 μM). The tested compounds displayed EC50 values lower than 110 mM, except the compound 13e with EC50 values of 155 μM. The compounds containing substituted benzyl moiety (13b-i) were more potent than unsubstituted corresponding compounds (13a). Among the compounds containing substituted benzyl moiety, increased activity was achieved when the substituents including fluoro, chloro, or methyl were introduced at the 2-, 3-, or 4-positions of benzyl ring, respectively (compounds 13g, 13h, 13f, and 13d). The compounds bearing 4-methoxybenzyl (13i) or 2- and 3-methylbenzyl groups (13b and 13c) showed lower potencies. The best activity was observed for compound 13g with EC50 value of 40 μM and SI value of 13.7.
Molecular modeling
In order to examine the binding mechanism of our synthesized benzimidazolyl DKA derivatives, the most active compound 13g, was selected for molecular docking studies. We performed molecular modeling studies by AutoDock Tools software using the X-ray structures of prototype foamy virus (PFV) intasome, an acceptable model for HIV integrase active site, in complex with double-strand DNA, two metal ions, and Raltegravir (PDB: 3OYA) (41, 42). The docking pose of compound 13g within HIV IN CCD is illustrated in Figure 3a. According to the docking results, 13g fits in the IN binding site perfectly through binding of a chelating triad in DKA with two Mg+2 ions present in the active site by the distance of 2.58, 2.51, 1.95, and 2.76 Å. On the other hand, based on the docking analysis, more confirmation is obtained by π-π stacking of 2-flourobenzyl group via placing in the hydrophobic pocket composed by viral nucleotide DA17 and DC16 and Pro214. Moreover, Figure 3b reveals that the binding mode of compound 13g resembles that of Raltegravir, suggesting that the tested compounds may exhibit the anti-HIV-1 activity through the inhibition of integrase enzyme.
Cytotoxicity and Anti-HIV Activities of Compounds 13a-i
Cpd. | R | Anti-HIV-1 activity (EC50, µM) | Cytotoxicity (CC50, µM) | SI |
13a | H | 110 | 500 | 4.5 |
13b | 2-Me | 60 | 590 | 9.8 |
13c | 3-Me | 75 | 430 | 5.7 |
13d | 4-Me | 50 | 580 | 11.6 |
13e | 2-Cl | 155 | 380 | 2.4 |
13f | 3-Cl | 55 | 410 | 7.4 |
13g | 2-F | 40 | 550 | 13.7 |
13h | 3-F | 55 | 480 | 8.7 |
13i | 3-OMe | 90 | 490 | 5.4 |
Raltegravir | 0.9 | >500 | - |
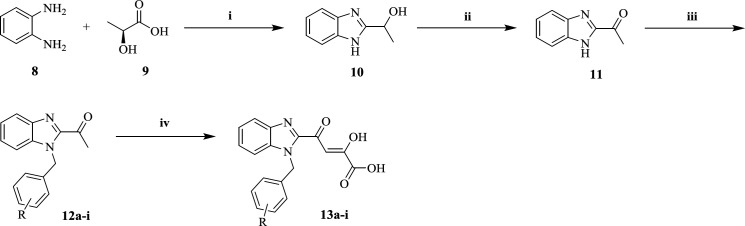
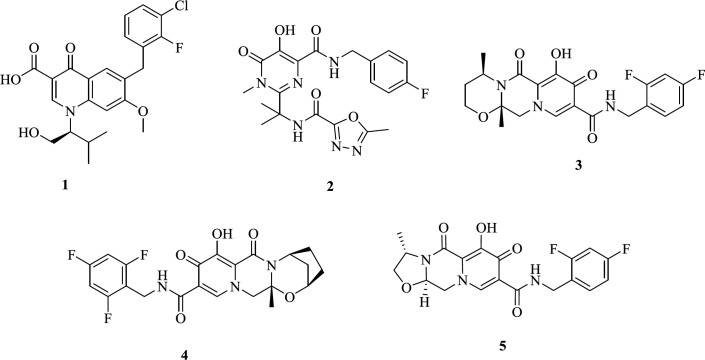
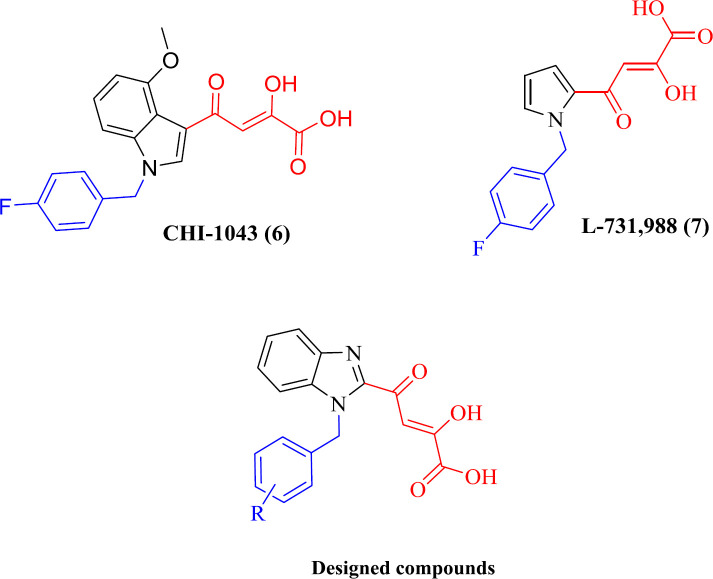
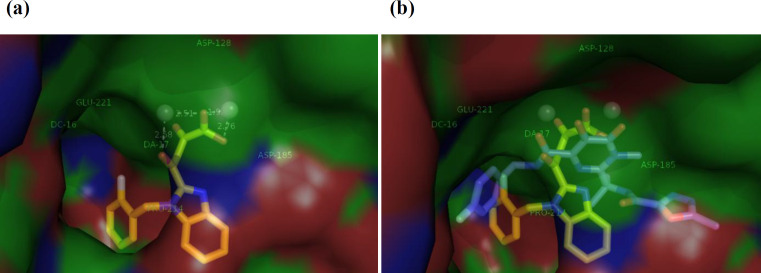
Conclusion
We have designed, synthesized, and evaluated a series of novel benzimidazolyl DKA derivatives bearing different substituted benzyl moiety for their inhibition of HIV-1 in cell culture. Most of the designed compounds demonstrated good to moderate potency with no significant cytotoxicity. The compound 13g was found to be the most active in this series with An EC50 value of 40 μM and SI value of 13.7. Docking studies indicated that the binding mode of compound 13g was similar to INSTIs. Therefore, benzimidazolyl DKA represents a potentially useful platform for further structural variations to find more potent anti-HIV-1 compounds.
Acknowledgements
References
-
1.
Zhan P, Pannecouque C, De Clercq E, Liu X. Anti-HIV Drug Discovery and Development: Current Innovations and Future Trends. J. Med. Chem. 2016;59:2849-78. [PubMed ID: 26509831].
-
2.
Kinch MS, Patridge E. An analysis of FDA-approved drugs for infectious disease: HIV/AIDS drugs. Drug Discov. Today. 2014;19:1510-3. [PubMed ID: 24880109].
-
3.
Meintjes G, Moorhouse MA, Carmona S, Davies N, Dlamini S, van Vuuren C, Manzini T, Mathe M, Moosa Y, Nash J, Nel J, Pakade Y, Woods J, Van Zyl G, Conradie F, Venter F. Adult antiretroviral therapy guidelines 2017. South Afr. J. HIV. Med. 2017;18:776.
-
4.
Bushman FD, Craigie R. Activities of human immunodeficiency virus (HIV) integration protein in vitro: specific cleavage and integration of HIV DNA. Proc. Natl. Acad. Sci. USA. 1991;88:1339-43. [PubMed ID: 1847518].
-
5.
Pommier Y, Pilon A, Bajaj K, Mazumder A, Neamati N. HIV-1 Integrase as a Target for Antiviral Drugs. Antivir. Chem. Chemother. 1997;8:463-83.
-
6.
Zeinalipour-Loizidou E, Nicolaou C, Nicolaides A, Kostrikis LG. HIV-1 integrase: from biology to chemotherapeutics. Curr. HIV Res. 2007;5:365-88. [PubMed ID: 17627500].
-
7.
McColl DJ, Chen X. Strand transfer inhibitors of HIV-1 integrase: bringing IN a new era of antiretroviral therapy. Antivir. Res. 2010;85:101-18. [PubMed ID: 19925830].
-
8.
Yoder KE, Bushman FD. Repair of Gaps in Retroviral DNA Integration Intermediates. J. Virol. 2000;74:11191. [PubMed ID: 11070016].
-
9.
Nair V, Chi G. HIV integrase inhibitors as therapeutic agents in AIDS. Rev. Med. Virol. 2007;17:277-95. [PubMed ID: 17503547].
-
10.
Pommier Y, Johnson AA, Marchand C. Integrase inhibitors to treat HIV/AIDS. Nat. Rev. Drug Discov. 2005;4:236-48. [PubMed ID: 15729361].
-
11.
Quashie PK, Sloan RD, Wainberg MA. Novel therapeutic strategies targeting HIV integrase. BMC Med. 2012;10:34. [PubMed ID: 22498430].
-
12.
Yang LL, Li Q, Zhou LB, Chen SQ. Meta-analysis and systematic review of the efficacy and resistance for human immunodeficiency virus type 1 integrase strand transfer inhibitors. Int. J. Antimicrob. Agents. 2019;54:547-55. [PubMed ID: 31398480].
-
13.
Wills T, Vega V. Elvitegravir: a once-daily inhibitor of HIV-1 integrase. Expert Opin. Investig. Drugs. 2012;21:395-401.
-
14.
Croxtall JD, Scott LJ. Raltegravir. Drugs. 2010;70:631-42. [PubMed ID: 20329808].
-
15.
Ballantyne AD, Perry CM. Dolutegravir: First Global Approval. Drugs. 2013;73:1627-37. [PubMed ID: 24052331].
-
16.
Johns BA, Kawasuji T, Weatherhead JG, Taishi T, Temelkoff DP, Yoshida H, Akiyama T, Taoda Y, Murai H, Kiyama R, Fuji M, Tanimoto N, Jeffrey J, Foster SA, Yoshinaga T, Seki T, Kobayashi M, Sato A, Johnson MN, Garvey EP, Fujiwara T. Carbamoyl Pyridone HIV-1 Integrase Inhibitors 3. A Diastereomeric Approach to Chiral Nonracemic Tricyclic Ring Systems and the Discovery of Dolutegravir. S/GSK1349572;and (S/GSK1265744).J. Med. Chem. (2013) 56:5901-16.
-
17.
Peese KM, Naidu BN, Patel M, Li C, Langley DR, Terry B, Protack T, Gali V, Lin Z, Samanta HK, Zheng M, Jenkins S, Dicker IB, Krystal MR, Meanwell NA, Walker MA. Heterocycle amide isosteres: An approach to overcoming resistance for HIV-1 integrase strand transfer inhibitors. Bioorg. Med. Chem. Lett. 2020;30:126784.
-
18.
Wohl DA, Yazdanpanah Y, Baumgarten A, Clarke A, Thompson MA, Brinson C, Hagins D, Ramgopal MN, Antinori A, Wei X, Acosta R, Collins SE, Brainard D, Martin H. Bictegravir combined with emtricitabine and tenofovir alafenamide versus dolutegravir, abacavir, and lamivudine for initial treatment of HIV-1 infection: week 96 results from a randomised, double-blind, multicentre, phase 3, non-inferiority trial. Lancet HIV. 2019;6:e355-e63. [PubMed ID: 31068270].
-
19.
Sax PE, DeJesus E, Crofoot G, Ward D, Benson P, Dretler R, Mills A, Brinson C, Peloquin J, Wei X, White K, Cheng A, Martin H, Quirk E. Bictegravir versus dolutegravir, each with emtricitabine and tenofovir alafenamide, for initial treatment of HIV-1 infection: a randomised, double-blind, phase 2 trial. Lancet HIV. 2017;4:e154-e60. [PubMed ID: 28219610].
-
20.
Mesplède T, Quashie PK, Zanichelli V, Wainberg MA. Integrase strand transfer inhibitors in the management of HIV-positive individuals. Ann. Med. 2014;46:123-9. [PubMed ID: 24579911].
-
21.
Pandey KK, Grandgenett DP. HIV-1 Integrase Strand Transfer Inhibitors: Novel Insights into their Mechanism of Action. Retrovirology. 2008;2:11-6. [PubMed ID: 19915684].
-
22.
Adhikari N, Halder A, Mondal C, Jha T. Structural findings of quinolone carboxylic acids in cytotoxic, antiviral, and anti-HIV-1 integrase activity through validated comparative molecular modeling studies. Med. Chem. Res. 2014;23.
-
23.
Hajimahdi Z, Zarghi A. Progress in HIV-1 Integrase Inhibitors: A Review of their Chemical Structure Diversity. Iran. J. Pharm. Res. 2016;15:595-628. [PubMed ID: 28243261].
-
24.
Pons-Faudoa FP, Sizovs A, Di Trani N, Paez-Mayorga J, Bruno G, Rhudy J, Manohar M, Gwenden K, Martini C, Chua CYX, Varchi G, Marzinke MA, Grattoni A. 2-Hydroxypropyl-beta-cyclodextrin-enhanced pharmacokinetics of cabotegravir from a nanofluidic implant for HIV pre-exposure prophylaxis. J. Control. Release. 2019;306:89-96. [PubMed ID: 31136811].
-
25.
Margolis DA, Gonzalez-Garcia J, Stellbrink HJ, Eron JJ, Yazdanpanah Y, Podzamczer D, Lutz T, Angel JB, Richmond GJ, Clotet B, Gutierrez F, Sloan L, Clair MS, Murray M, Ford SL, Mrus J, Patel P, Crauwels H, Griffith SK, Sutton KC, Dorey D, Smith KY, Williams PE, Spreen WR. Long-acting intramuscular cabotegravir and rilpivirine in adults with HIV-1 infection (LATTE-2): 96-week results of a randomised, open-label, phase 2b, non-inferiority trial. Lancet. 2017;390:1499-510. [PubMed ID: 28750935].
-
26.
Deo K, Singhvi IJ, Patil SR, Patil A. Docking, Synthesis and Biological Evaluation of Novel Diketoquinoline Analogues as HIV-1 Integrase Inhibitor. Asian J. Chem. 2019;31:2000-8.
-
27.
Corona A, Di Leva FS, Rigogliuso G, Pescatori L, Madia V, Subra F, Delelis O, Esposito F, Cadeddu M, Costi R, Cosconati S, Novellino E, Di Santo R, Tramontano E. New insights into the interaction between pyrrolyl diketoacids and HIV-1 integrase active site and comparison with RNase H. Antivir. Res. 2016;134:236.
-
28.
Di Santo R. Diketo Acids Derivatives as Dual Inhibitors of Human Immunodeficiency Virus Type 1 Integrase and the Reverse Transcriptase RNase H Domain. Curr. Med. Chem. 2011;18:3335-42. [PubMed ID: 21728968].
-
29.
Beare KD, Coster MJ, Rutledge PJ. Diketoacid inhibitors of HIV-1 integrase: from L-708,906 to raltegravir and beyond. Curr. Med. Chem. 2012;19:1177-92. [PubMed ID: 22214459].
-
30.
De Luca L, Gitto R, Christ F, Ferro S, De Grazia S, Morreale F, Debyser Z, Chimirri A. 4-[1-(4-Fluorobenzyl)-4-hydroxy-1H-indol-3-yl]-2-hydroxy-4-oxobut-2-enoic acid as a prototype to develop dual inhibitors of HIV-1 integration process. Antivir. Res. 2011;92:102-7. [PubMed ID: 21767569].
-
31.
Azami Movahed M, Daraei B, Shahosseini S, Esfahanizadeh M, Zarghi A. Design, synthesis, and biological evaluation of new pyrazino[1,2-a]benzimidazole derivatives as selective cyclooxygenase (COX-2) inhibitors. Arch. Pharm. 2019;352:e1800265.
-
32.
Hajimahdi Z, Zabihollahi R, Aghasadeghi MR, Zarghi A. Design, synthesis and docking studies of new 4-hydroxyquinoline-3-carbohydrazide derivatives as anti-HIV-1 agents. Drug Res. 2013;63:192-7.
-
33.
Ebrahimzadeh E, Tabatabai SA, Vahabpour R, Hajimahdi Z, Zarghi A. Design, synthesis, molecular modeling study and biological evaluation of new N’-arylidene-pyrido [2,3-d]pyrimidine-5-carbohydrazide derivatives as anti-HIV-1 agents. Iran. J. Pharm. Res. 2019;18:237-48. [PubMed ID: 32802103].
-
34.
Safakish M, Hajimahdi Z, Aghasadeghi MR, Vahabpour R, Zarghi A. Design, synthesis, molecular modeling and anti-HIV assay of novel quinazolinone incorporated coumarin derivatives. Curr. HIV Res. 2020;18:41-51. [PubMed ID: 31820700].
-
35.
Trott O, Olson AJ. AutoDock Vina: improving the speed and accuracy of docking with a new scoring function, efficient optimization, and multithreading. J. Comput. Chem. 2010;31:455-61. [PubMed ID: 19499576].
-
36.
Morris GM, Goodsell DS, Halliday RS, Huey R, Hart WE, Belew RK, Olson AJ. Automated docking using a Lamarckian genetic algorithm and an empirical binding free energy function. J. Comput. Chem. 1998;19:1639-62.
-
37.
HyperChem(TM) Professional 8.0. 1115 NW 4th Street, Gainesville, Florida 32601, USA: Hypercube, Inc;
-
38.
Hsieh CY, Ko PW, Chang YJ, Kapoor M, Liang YC, Lin HH, Horng JC, Hsu MH. Design and Synthesis of Benzimidazole-Chalcone Derivatives as Potential Anticancer Agents. Molecules. 2019;24:3259.
-
39.
Zhu W, Zhai X, Fu Q, Guo F, Bai M, Wang J, Wang H, Gong P. Design, synthesis and anticancer activity of 4-morpholinothieno[3,2-d]pyrimidine derivatives bearing arylmethylene hydrazine moiety. Chem. Pharm. Bull. 2012;60:1037-45.
-
40.
Joksimović N, Baskić D, Popović S, Zarić M, Kosanić M, Ranković B, Stanojković T, Novaković SB, Davidović G, Bugarčić Z, Janković N. Synthesis, characterization, biological activity, DNA and BSA binding study: novel copper(ii) complexes with 2-hydroxy-4-aryl-4-oxo-2-butenoate. Dalton Trans. 2016;45:15067-77. [PubMed ID: 27711668].
-
41.
Hare S, Gupta SS, Valkov E, Engelman A, Cherepanov P. Retroviral intasome assembly and inhibition of DNA strand transfer. Nature. 2010;464:232-6. [PubMed ID: 20118915].
-
42.
Hare S, Vos AM, Clayton RF, Thuring JW, Cummings MD, Cherepanov P. Molecular mechanisms of retroviral integrase inhibition and the evolution of viral resistance. Proc. Natl. Acad. Sci. USA. 2010;107:20057-62. [PubMed ID: 21030679].