Abstract
Keywords
Renal toxicity Nephrotoxicity Descurainia Sophia Gentamicin Acute kidney injury
Introduction
Drug-induced acute kidney injury (AKI) is a dreadful condition comprising around 60% of all renal toxicities (1). On the other hand, 3.2% of all adverse reactions induced by drugs have been against renal cells (2). Intravenous antibiotics including vancomycin and gentamicin (Gm) have been responsible for about 29% of all drug-induced AKIs (2, 3). Gm is a widely used antibiotic belonging to aminoglycosides used to treat infections caused by gram-negative bacteria (4). Despite its high anti-bacterial efficiency, Gm is deterred due to potential renal toxicity (5). AKI has been noted in as high as 10-25% of the patients treated with Gm; even those received a single dose of this drug (6-9). Renal tubules uptake around 10% of intravenously administrated Gm which is considered to be the primary source of renal structural and functional alternations (5).
It is believed that oxidative stress plays a major role in Gm-induced AKI; nevertheless, a multifactorial phenomenon seems to be more plausible. The antioxidant powers in the kidneys exposed to Gm are reduced leading to renal tubular and epithelial cells necrosis and apoptosis. Gm also promotes cellular death by activating mitochondrial initiated apoptotic pathway and B cell lymphoma-2 (Bcl-2) associated protein X (Bax) (10). Furthermore, renal tubular injury in Gm-associated renal dysfunction is associated with attenuated glomerular filtration rate (8).
Extensive efforts have been dedicated to find agents that prevent or at least reduce the nephrotoxicity effects of Gm. In this regard, a recent study has reviewed 54 nephroprotectants evaluated in animal models (11). These agents can be categorized in various subclasses including anti-oxidative and anti-inflammatory agents, diuretics, calcium channel blockers, and inhibitors of aminoglycoside tubular uptake (11). In particular, plants extracts with anti-oxidant and anti-inflammatory properties have been always under attention as nephroprotective agents (12).
Descurainia sophia is a plant member of Brassicaceae family. This plant, which is also known as “flixweed” or “Khak-e-sheer” (in Iranian traditional medicine), grows in a wide geographic belt expanding throughout Asia, North Africa, North America, and Europe (13). This plant has been used to alleviate asthma, cough, cardiac dysfunction, and edema in Iranian, Chinese and Indian traditional medicines (13-15). It has been shown that at least 26 biologically active elements regulating metabolism of amino acids, metabolic pathways, and energy expenditure are present in the aqueous seed extract of Descurainia sophia (16). In another study, Lee et al isolated 14 active components from the seeds of Descurainia sophia and investigated their anti-proliferative and anti-inflammatory effects (17). Alcoholic extract of Descurainia sophia has been shown to present antipyretic, anti-inflammatory, analgesic (18), anti-tumor, anti-proliferative (19, 20) and anti-asthma (21) effects. In clinical terms, the beneficial role of Descurainia sophia has been reported in chronic heart diseases (22) and functional constipation (23) as well.
A variety of agents with wide-range biological activities such as dapagliflozin (5), sika deer antler protein (8), costus afer, hesperidin, grape seed extract, pinocembrin, Zn, Portulaca oleracea extract, dipyridamole, α-tocopherol (vitamin E), troxerutin, cobalamin, Rudgea viburnoides and apocynin have been evaluated as nephroprotectants in different models of AKI (24-37). All of these agents have resulted in variable results and side effects. However, despite diverse anti-oxidative and anti-inflammatory effects, the protective role of Descurainia sophia against AKI induced by Gm has not been studied. Therefore, we aimed to use an animal model of Gm-induced AKI to assess if extract of this plant can prevent or at least mitigate renal adverse effects induced by this antibiotic.
Experimental
To assess the protective effects of Descurainia sophia on Gm-induced renal insufficiency, 35 male Wistar rats were treated with the antibiotic for 7 consecutive days. The rats were provided by Tehran University of Medical Sciences animal house. The rats’ weight ranged as 200-250 grams, and they had free access to fresh water and food. They were kept in standard cages at room temperature (25 ± 3 °C), 50-60% moisture and under natural 12-hour dark/light cycle.
Also, based on old Persian pharmaceutical manuscripts (24), Descurainia sophia has been reported as a general antidote to neutralize toxicity of toxic herbs. Moreover, previous studies have shown that this herb has hepatoprotective activity against hepatotoxicity induced by acetaminophen (25) Therefore, and as a next step, our study has focused on the nephroprotective activity of this herb.
Plant extraction and standardization
Descurainia sophia seed was prepared from Tehran botanical market and authenticated by the Herbarium of School of Traditional Medicine, Shahid Beheshti University of Medical Sciences (HMS-520). hydroalcoholic extract of Descurainia sophia was prepared using a previously described method (26). Briefly, the seeds were ground and extracted by macerating the powder in 96% ethanol (1:1) for 24 h. Then, the extract was concentrated using rotary evaporation system (Hiedolph, Geremany) at 60°C. The extract was then left to dry in a desiccator. Based on a previously described method (27) and to standardize the extract, total phenolic content was determined via spectrophotometry and reported as gallic acid equivalent.
The extract consisted of an upper oil phase and a lower solid phase. The yield of extraction was 8.57% (6.2% for solid phase). The result, which is mean of three replicates showed that total phenolic compounds of the extract is 95 mg/g.
Experimental groups
The experimental groups included 1) control: administrating normal saline throughout the study; 2) group 1: four weeks normal saline, 3) group 2: four weeks of 800 mg/Kg Descurainia sophia extract, 4) Group 3: four weeks of 1600 mg/Kg of Descurainia sophia extract, and 5) Group 4: four weeks of 2400 mg/Kg Descurainia sophia extract. At the end of four weeks, the rats in all the experimental groups (i.e. group 1 to 4) received Gm injections (80 mg/Kg) for 7 consecutive days (Figure 1).
Sample collection and apoptosis evaluation
The rats were sacrificed at the end of treatments. The kidneys were removed, fixed in 4% formaldehyde solution and stored in 70% ethanol solution until the day of tissue processing and apoptosis evaluation. We used TUNEL apoptosis detection kit (Abbkine Scientific Co,USA) based on the manufacturer’s instructions. In this assay, DNA nicks and fragments are detected using florescence microscopy. The results were recorded as the percentage of the damaged cells.
Urine and blood biochemistry analysis
Biochemical assays were performed using standard protocols in a clinical laboratory. Plasma BUN, creatinine, cholesterol, triglyceride and urine creatinine levels were measured using spectrophotometric methods (Pars Azmon, Iran) on a calibrated automatic biochemistry analyzer (ELITech Group Clinical Systems, France). Blood and urine sodium concentrations were determined using a closed electrolyte analyzer (Caretium, China). Measurements were performed as baseline (i.e. before plant extract administration, day 1), at the day 28th (i.e. before starting Gm), and at the day 35th (i.e. the end of study).
Statistical analysis
Comparison between the measurement steps was done using repeated measure analysis of variances (ANOVA). The confidence interval level was considered 95%. TUNEL test results were compared between the groups using ANOVA and HSD-Tukey test.
Results
Effects of Descurainia sophia on BUN and creatinine level
Serum BUN level significantly increased in group 1 (four weeks normal saline and then treated 7 days Gm). However, pre-treatment with either 800, 1600 or 2400 mg/kg of Descurainia sophia dose-dependently prevented elevation of BUN following Gm treatment in Wistar rats with the most efficient dose that was 2400 mg/Kg (Figure 2). The rats in group 1 also had significantly increased serum creatinine level while those received various doses of Descurainia sophia showed significantly lower creatinine level with the lowest level observed in 2400 mg/kg group (Figure 3).
Effects of Descurainia sophia on urinary Na+ concentration and fractional excretion of sodium (FeNa+)
The rats received normal saline showing significant increase in urinary Na+ concentration and fractional excretion of sodium (FeNa+). On the other hand, pre-treatment with either 800, 1600 or 2400 mg/Kg of Descurainia sophia significantly decreased urine Na+ concentration (Figure 4) and FeNa+ (Figure 5) at all the studied doses.
Effects of Descurainia sophia on lipid profile
The serum levels of cholesterol (Figure 6) and triglycerides (Figure 7) levels significantly increased at the day 35th (i.e. 7 days after Gm exposure) but Pre-treatment with either 800, 1600 or 2400 mg/kg Descurainia sophia prevented increments in serum cholesterol and triglycerides levels in Wistar rats. All the doses were significantly efficient; however, the most potent effects were noticed in the dose of 2400 mg/Kg.
Effects of Descurainia sophia on apoptosis of kidney cells
Gm. treatment resulted in the apoptosis of kidney cells; however, treatment with Descurainia sophia pre-administration dose-dependently protected renal cells from Gm induced apoptosis (Figure 8).
Discussion
However, there were significant and dose-dependent reductions in serum BUN, creatinine, cholesterol and triglyceride, urinary Na+ excretion, apoptosis rate, and inflammatory cells infiltration in renal tissues. Other studies have also evaluated protective effects of various agents against Gm-induced AKI and reported similar findings as increased serum BUN and creatinine levels in animal models (5, 8, 32). Increased levels of BUN and creatinine in the context of Gm-induced renal impairment have been correlated with prominent aberrations in renal function and structure (33). In line, Gm administration for 8 days reduced creatinine clearance and renal blood flow in previous reports (32, 34). The rats exposed to Gm have also shown dose-dependent decline in glomerular filtration rate (29, 35). In support, Troxerutin,a plant flavonoid, was able to correct alternations in urinary protein and albumin levels, as well as serum creatinine and BUN in part by augmenting glomerular filtration rate (29). Although elevations in serum BUN and creatinine indicate renal functional impairment, these traditional markers may not be adequately sensitive to reveal renal toxicity in early phases (36). Some novel markers including urinary neutrophil gelatinase associated protein (NGAL), β2-microglobulin, albumin, clusterin, glucose excretion, histological findings, urinary level of kidney injury molecule-1 (KIM-1) and alpha glutathione S-transferase have been suggested to develop a multisystem model for assessing drug induced renal injury (36-38). In mice and HEK293 cells, Gm exposure increased urinary levels of KIM-1, NGAL, and Cys-C activities (8, 29). In addition, as early as four days post Gm-exposition, increased expression of four miRNAs (miR-138-5p miR-1971, miR-218-1-3p, and miR-489) predicted Gm-induced toxicity before elevation of serum BUN and creatinine (39). With advancements in diagnostic methods, novel biomarkers have been proposed to be used as alternatives to serum BUN and creatinine to early detect drug-induced AKI. In current study, pre-treatment with either 800, 1600 or 2400 mg/Kg of Descurainia sophia significantly decreased urine Na+ concentration and FeNa+ at all the studied doses. In accordance, Gm treatment exerted similar patterns in Na+ levels in previous studies (34, 40). Also previous experiments showed that Gm treatment significantly increased FeNa+ level (41, 42). Alternations in electrolytes distribution in Gm-induced AKI can be related to the depressed activity of Na+, K+-ATPase pump in proximal renal tubules correlating with higher Na+ excretion (43). Nevertheless, Sugarman et al described that Gm had no significant impact on the activity of this transporter in renal medullary or cortical cells (35). Gm-induced accumulation and release of Na+ and K+ respectively from renal proximal tubular cells can also contribute to the disturbed electrolyte turnover in Gm-induced nephrotoxicity (44). In fact, the concentration dysfunction in tubular cells, rather than defects in sodium excretion disturbance, has been suggested as the main cause of disturbed Na+ distribution induced by Gm (35). According to studies, Gm damages tubular tissue in kidneys resulting in functional disturbances of tubular cells in regulating the balance of electrolytes and other molecules (45). Trace elements perform important biological roles in the body including participation as structural elements in enzymes, proteins, and hormones (46), and using agents protecting electrolyte disturbance can result in healthy renal and other organs functions. Pre-treatment with either 800, 1600, or 2400 mg/kg Descurainia sophia prevented increments in serum cholesterol and triglycerides levels in Wistar rats. All the doses were significantly efficient; however, the most potent effects were noticed in the dose of 2400 mg/Kg. In line with this, Anandan et al in their study also noted that hesperidin effectively prevented Gm-induced elevations of serum cholesterol, free fatty acids, and triglycerides in rats (30). It has been shown that Gm can damage lysosomes resulting in high levels of membrane lipids (sphingomyelin and sphingosine) in serum (47). However, studies evaluating the effects of Gm on lipid profile and its interaction with renoprotective pathways are scare and more studies are needed in this area. Moreover, the results of this study showed that treatment with Descurainia sophia pre-administration dose-dependently protected renal cells from Gm induced apoptosis. This observation was in line with a previous report indicating higher apoptosis rate in Gm-induced renal cells (29). Accordingly, after 14 days of administration, Gm induced apoptosis in renal tubular cells in rats (5). In addition to in-vivo models, Gm also induced dose-dependent apoptosis in HEK293 (1, 8) and HK-2 (1) kidney cell lines. Gm was shown to activate Bax; a proapoptotic member of Bcl-2 family, which subsequently triggers caspase-3 dependent cellular death (48). Increased expression of Fas ligand (CD95) in renal cells can also contribute to Gm-induced apoptosis (32). In accordance with our results, Descurainia sophia extract reduced apoptosis rate in cardiomyocytes of rat model of cardiac disease which was concomitant with depressed levels of Bax and caspase 3 (22). In A549 tumor cells, Descurainia sophia seed extract induced the expression of death receptors 4 (DR4) and 5 (DR5) through activating endoplasmic reticulum stress response pathway (49). In another study, dapagliflozin inhibited Gm-induced renal tubular cells apoptosis in part by increasing miR-21 and decreasing miR-181a expressions (5). Overall, the exact anti-apoptotic mechanisms of Descurainia sophia in Gm-induced renal injury are to be elucidated.
Finally, Gm treatment for 7 consecutive days resulted in renal cellular damage and infiltration of inflammatory cells in kidney tissue in the rats receiving saline. Pre-treatment with Descurainia sophia significantly reduced cellular injuries and recruitment of inflammatory cells to kidney tissue (Figure 9). An interstitial nephritis may also accompany the tubular damage by Gm (53-57). A lymphoproliferative phenomenon was also observed in renal tissue of Gm-exposed rats in a study by Oliveira et al (50). In other studies, histological characteristics have been noted in Gm-treated kidneys as degenerative changes (32, 51) and vaculation (5) in tubular epithelial cells (5, 36) and glomeruli (30), proliferative glomerulonephritis (32), tubular necrosis (30, 33, 35, 40, 51, 52), inter-tubular bleeds (5), vascular inflammation (5), tubular hyaline casts formation (5, 35, 37, 40) and dilation of renal tubules (5, 37). Gm nephrotoxicity has been associated with ultrastructural changes in basal membrane of glomeruli and renal tubules as well (53). Gm is filtrated through glomeruli, and during this process, binds to negatively charged structures triggering membrane permeability dysfunction and finally necrosis of tubular cells (54). The essential role of tubular necrosis in Gm-induced renal toxicity is supported by the reversal of renal function after inhibition of tubular necrosis by pre-administration with dipyridamole (33). In addition, dapagliflozin restored structural and functional capacities of kidneys in the rats treated with Gm through inhibiting renal tubular cells apoptosis (5). Vitamin E (α-tocopherol) also corrected functional deficiencies of Gm-exposed kidneys in part through reversing structural changes in glomerular basal membrane and tubules (53). Accordingly, as the role of inflammatory cells in histopathological events in Gm-induced renal injury has been indicated, the administration of anti-inflammatory agents such as Descurainia sophia can be beneficial.
Gm-induced nephrotoxicity is mediated through a complex multifactorial phenomenon with various pathways participating in this process. Among the most important toxic effects of Gm is inducing oxidative stress by overproducing oxidative agents such as reactive oxygen species (ROS) and depressing anti-oxidative capacities in renal cells. Multiple reports have verified the role of oxidative stress in adverse renal effects of Gm in rats (5, 8, 34, 50, 53). Anti-oxidant enzymes and mediators including super oxide dismutase (SOD), glutathione peroxidase, heme oxygenase -1, catalase and glutathione have been depressed in kidneys of Gm-treated rats (6, 29, 30, 32). The rats treated with Gm have shown higher levels of malondialdehyde (MDA), a marker of lipid peroxidation, in renal tissue (29, 32, 50). In fact many protective agents have exerted their effects at least in part by reducing oxidative markers and augmenting anti-oxidative mechanisms (6, 8, 29, 34, 48, 51, 53). In line, the anti-oxidative effects of Descurainia sophia have been established in multiple reports (16-18). Propagation of oxidants within renal tissue leads to structural changes in proteins, lipids, DNA, as well as functional exhaustion of critical organelles such as mitochondria (53). Subsequently, these molecular events can trigger adverse pathological outcomes and tubular necrosis as one of the main underlying causes of Gm-induced nephrotoxicity (55). Therefore, using anti-oxidative agents such as Descurainia sophia can be helpful to prevent adverse effects of Gm on kidneys.
Gm also recruits inflammatory mediators to promote its nephrotoxic effects. This is supported by studies that showed beneficial effects of anti-inflammatory agents in preventing Gm-induced AKI (48, 51). Elevated levels of inflammatory cytokines such as interleukin-6 (IL-6), IL-10, and tumor necrosis factor-α (TNF-α) have been described in renal tissue of models of Gm-induced AKI (8, 29, 33). On the other hand, Descurainia sophia seeds extract decreased the expression of IL-4, a major marker of type 2 helper T response and inflammation, in mouse model of asthma (21). In mouse model of asthma, Descurainia sophia seeds extract also downregulated VEGFa (21). Other protective mechanisms by Descurainia sophia may be exerted by encountering the processes initiated by Gm. For example, the plant extract through its active ingredients can modulate signaling pathways such as endoplasmic reticulum stress (i.e. unfolded protein response) (49) and PI3k/Akt/mTOR pathways (22). Helveticoside; an active ingredient of Descurainia sophia was shown to role as a potent regulator of intracellular signaling pathways and gene expression (56). Finally, ethanolic extract of Descurainia sophia seeds suppressed the activity of cytochrome P450 isoforms (i.e. CYP1A2, CYP2C9, and CYP2C19) which are essential enzymes participating in metabolization of drugs (57-59). Other underlying mechanisms by which Descurainia sophia extract and its ingredient suppress adverse effects of Gm on renal tissue are yet to be identified.
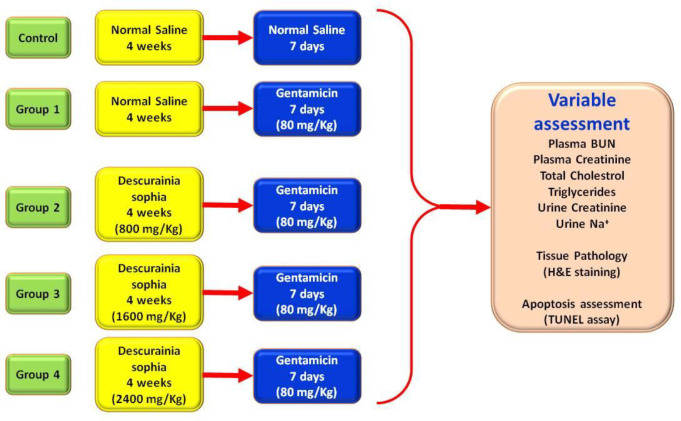
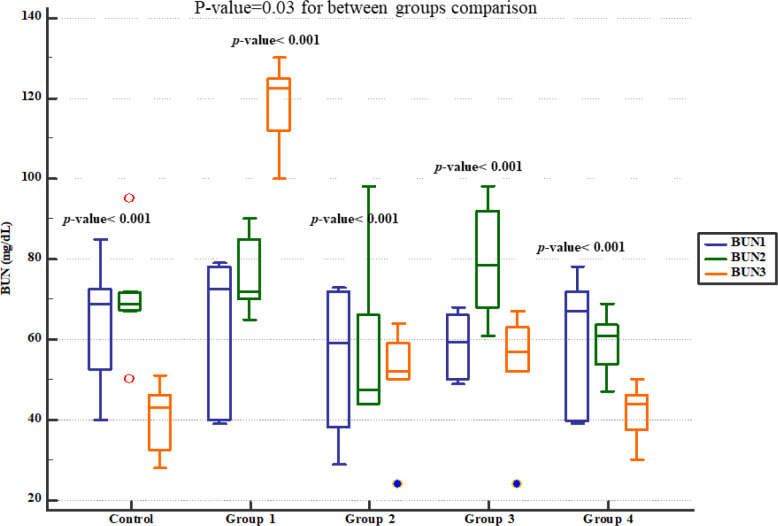
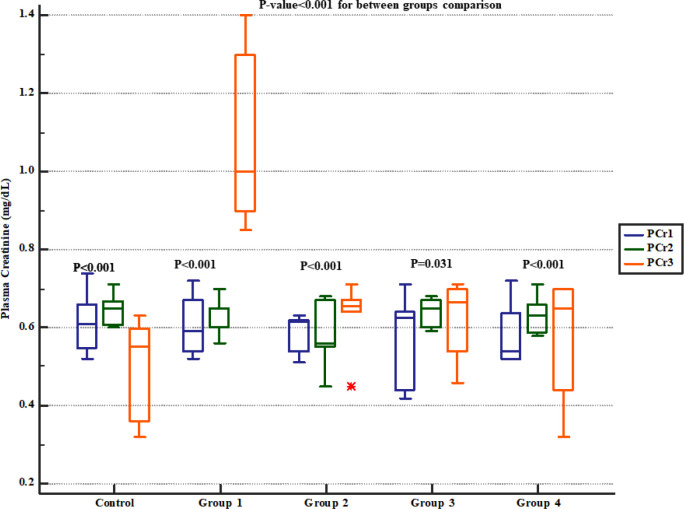
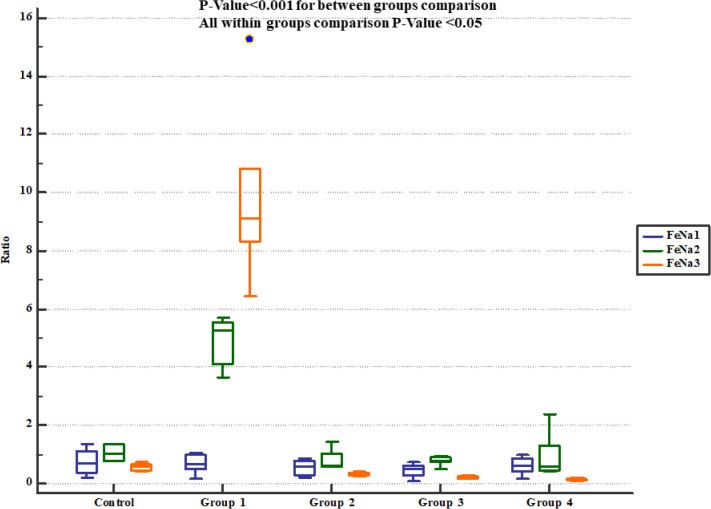
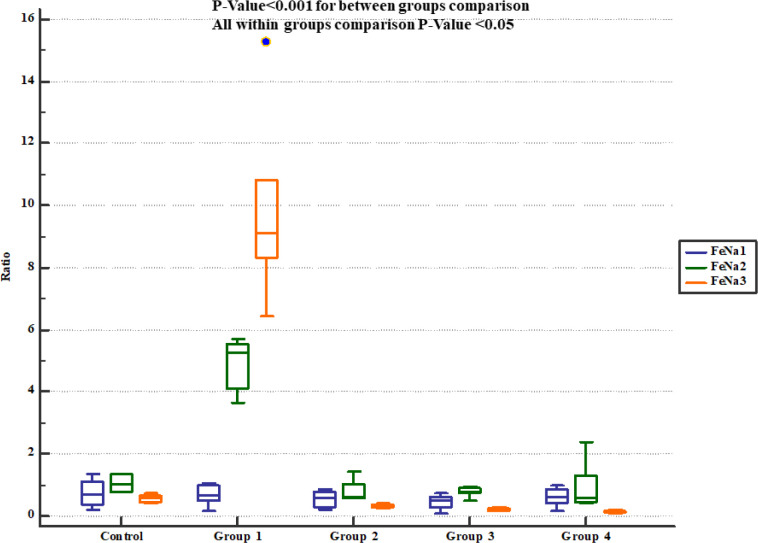
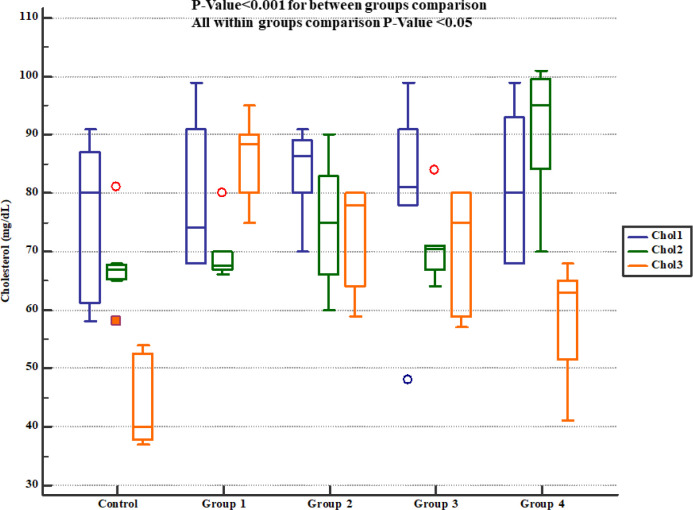
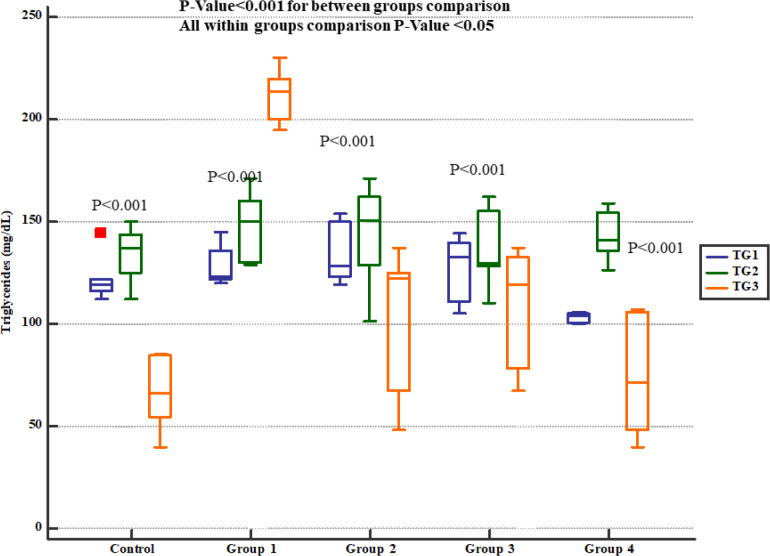
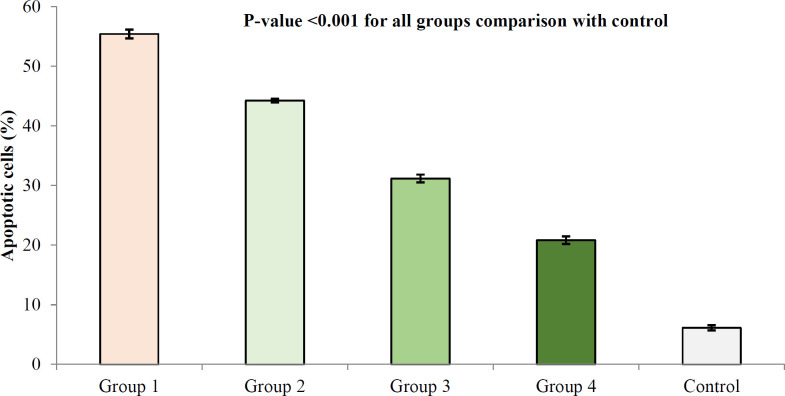
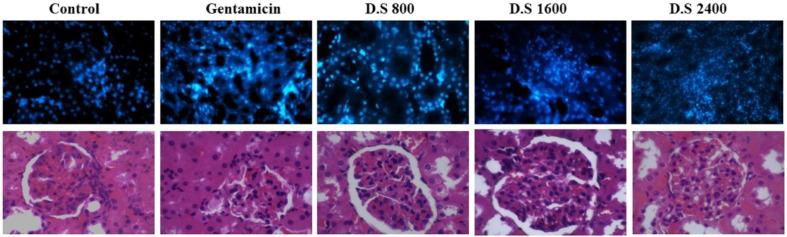
Conclusion
We showed that Descurainia sophia was effective in preventing adverse effects of Gm on renal tissue. This was reflected in reduced serum BUN and creatinine; two indicators of renal toxicity in Wistar rats exposed to Gm for 7 consecutive days. Other biochemical and histological markers indicating protective role of Descurainia sophia included reduction in Na+ urinary concentration and FeNa+, as well as lower recruitment of inflammatory cells and apoptosis in renal tissue. These effects were dose dependent and were more pronounced in higher dose of Descurainia sophia extract. Regarding these observations, and excellent tolerability and safety of this plant, it is applicable to use this plant as a preventive agent in the patients receiving Gm.
References
-
1.
Campos MA, de Almeida LA, Grossi MF, Tagliati CA. In-vitro evaluation of biomarkers of nephrotoxicity through gene expression using gentamicin. J. Bodyw. Mov. Ther;2018) 32:e22189.
-
2.
Pierson-Marchandise M, Gras V, Moragny J, Micallef J, Gaboriau L, Picard S. The drugs that mostly frequently induce acute kidney injury: a case - noncase study of a pharmacovigilance database. Br. J. Clin. Pharmacol. 2017;83:1341-9. [PubMed ID: 28002877].
-
3.
Slater MB, Gruneir A, Rochon PA, Howard AW, Koren G, Parshuram CS. Identifying high-risk medications associated with acute kidney injury in critically Ill patients: a pharmacoepidemiologic evaluation. Paediatr. Drugs. 2017;19:59-67. [PubMed ID: 27943125].
-
4.
Brereton CJ, Lennon D, Browning S, Dunn E, Ferguson JK, Davis JS. Is gentamicin safe and effective for severe community-acquired pneumonia? An 8-year retrospective cohort study. Int. J. Antimicrob. Agents. 2018;51:862-6. [PubMed ID: 29410326].
-
5.
Mohamed DI, Khairy E, Saad SST, Habib EK, Hamouda MA. Potential protective effects of Dapagliflozin in gentamicin induced nephrotoxicity rat model via modulation of apoptosis associated miRNAs. Gene. 2019;707:198-204. [PubMed ID: 31075409].
-
6.
Duarte F, Pessoa EA, Reis LA, Schor N, Borges FT. Priming prevent nephrotoxic acute renal failure through stimulation of antioxidant defense mechanism. J. Bras. Nefrol. 2016;38:161-72. [PubMed ID: 27438971].
-
7.
Sia CS, Ananda-Rajah MR, Adler NR, Yi-Wei B, Liew D, Tong EY. Renal safety of short-term empiric gentamicin therapy in aged patients. Australas. J. Ageing. 2018;37:227-31. [PubMed ID: 29704297].
-
8.
Sun H, Yang H, Ruan H, Li W, He X, Wang L. The protective effect of sika deer antler protein on gentamicin-induced nephrotoxicity in-vitro and in-vivo. Cell Physiol. Biochem. 2018;50:841-50. [PubMed ID: 30355934].
-
9.
Hayward RS, Harding J, Molloy R, Land L, Longcroft-Neal K, Moore D. Adverse effects of a single dose of gentamicin in adults: a systematic review. Br. J. Clin. Pharmacol. 2018;84:223-38. [PubMed ID: 28940715].
-
10.
Martínez-Salgado C, Eleno N, Morales AI, Pérez-Barriocanal F, Arévalo M, López-Novoa JM. Gentamicin treatment induces simultaneous mesangial proliferation and apoptosis in rats. Kidney Int. 2004;65:2161-71. [PubMed ID: 15149329].
-
11.
Vicente-Vicente L, Casanova AG, Hernandez-Sanchez MT, Pescador M, Lopez-Hernandez FJ, Morales AI. A systematic meta-analysis on the efficacy of pre-clinically tested nephroprotectants at preventing aminoglycoside nephrotoxicity. Toxicology. 2017;377:14-24. [PubMed ID: 27940129].
-
12.
Boozari M, Hosseinzadeh H. Natural medicines for acute renal failure: A review. Phytother. Res. 2017;31:1824-35. [PubMed ID: 29027276].
-
13.
Sun K, Li X, Liu JM, Wang JH, Li W, Sha Y. A novel sulphur glycoside from the seeds of Descurainia sophia (L ). J. Asian Nat. Prod. Res. 2005;7:853-6. [PubMed ID: 16308204].
-
14.
Khan M, Wang N. Descurainia sophia ( ): a weed with multiple medicinal uses. Punjab. Univ. J. Zool. 2012;27:45-51.
-
15.
Zarshenas MM, Petramfar P, Firoozabadi A, Moein MR, Mohagheghzadeh A. Types of headache and those remedies in traditional persian medicine. Pharmacogn. Rev. 2013;7:17-26. [PubMed ID: 23922452].
-
16.
Zhou N, Sun YP, Zheng XK, Wang QH, Yang YY, Bai ZY. A Metabolomics-based strategy for the mechanism exploration of traditional Chinese medicine: Descurainia sophia seeds extract and fractions as a case study. Evid. Based Complementary Altern. Med. 2017;2017:2845173.
-
17.
Lee YJ, Kim NS, Kim H, Yi JM, Oh SM, Bang OS. Cytotoxic and anti-inflammatory constituents from the seeds of Descurainia sophia. Arch. Pharm. Res. 2013;36:536-41. [PubMed ID: 23435946].
-
18.
Nimrouzi M, Zarshenas MM. Phytochemical and pharmacological aspects of Descurainia sophia Webb ex Prantl: modern and traditional applications. Avicenna J. Phytomed. 2016;6:266-72. [PubMed ID: 27462549].
-
19.
Gui J, Zhu M, Bai X, Li B, Gao M, Ma H. Effect of methanol-ethyl acetate partitioned fractions from Descurainia sophia on proliferation and apoptosis of human non-small cell lung cancer H1975 cells. Nan fang yi ke da xue xue bao. 2019;39:169-74. [PubMed ID: 30890504].
-
20.
Khodarahmi E, Asghari GH, Hassanzadeh F, Mirian M, Khodarahmi GA. Cytotoxic evaluation of volatile oil from Descurainia sophia seeds on MCF-7 and HeLa cell lines. Res. Pharm. Sci. 2015;10:169-76. [PubMed ID: 26487894].
-
21.
Baek SJ, Chun JM, Kang TW, Seo YS, Kim SB, Seong B. Identification of epigenetic mechanisms involved in the anti-asthmatic effects of Descurainia sophia seed extract based on a multi-omics approach. Molecules. 2018;23:2879.
-
22.
Luo Y, Sun Z, Hu P, Wu Y, Yu W, Huang S. Effect of aqueous extract from Descurainia sophia (L ) webb ex prantl on ventricular remodeling in chronic heart failure rats. Evid. Based Complementary Altern. Med. 2018;2018:1904081.
-
23.
Choopani R, Ghourchian A, Hajimehdipoor H, Kamalinejad M, Ghourchian F. Effect of Descurainia sophia ( ) webb ex prantl on adult functional constipation: a prospective pilot study. J. Evid. Based Complementary Altern. Med. 2017;22:646-51. [PubMed ID: 28401774].
-
24.
Aghili Khorasani M. Makhzan ul-Advia. Bavardaran Press Research institute for Islamic and Complementary Medicine. Iran University of Medical Sciences; 2001. 122 p.
-
25.
Moshaie-Nezhad P, Faed Maleki F, Hosseini S, Yahyapour M, Iman M, Khamesipour A. Hepatoprotective and antioxidant effects of Hedera helix extract on acetaminophen induced oxidative stress and hepatotoxicity in mice. Biotech. Histochem. 2019:1-7.
-
26.
Moshaie-Nezhad P, Iman M, Faed Maleki F, Khamesipour A. Hepatoprotective effect of Descurainia sophia seed extract against paracetamol-induced oxidative stress and hepatic damage in mice. J. Herbmed. Pharmacol. 2018;7:267-72.
-
27.
Kashani LMT, Hatami A, Safaei A, Shirzad M, Ahmadian-Attari MM. Different traditional methods of Nux-Vomica detoxification have therapeutic rationales. Jundishapur J. Nat. Pharm. Prod. 2016;11:e25360.
-
28.
Rafieian-Kopaei M, Baradaran A, Rafieian M. Plants antioxidants: From laboratory to clinic. J. Nephropathol. 2013;2:152-3. [PubMed ID: 24475444].
-
29.
Salama SA, Arab HH, Maghrabi IA. Troxerutin down-regulates KIM-1, modulates p38 MAPK signaling, and enhances renal regenerative capacity in a rat model of gentamycin-induced acute kidney injury. Food Funct. 2018;9:6632-42. [PubMed ID: 30511081].
-
30.
Anandan R, Subramanian P. Renal protective effect of hesperidin on gentamicin-induced acute nephrotoxicity in male Wistar albino rats. Redox Rep. 2012;17:219-26. [PubMed ID: 22889751].
-
31.
Alirezaei AH, Shirzadeh Barough A, Azizi T, Shirzadeh Barough S, Ghorbanihaghjo A, Rashtchizadeh N, Babaie H, Delazar A. Anti-inflammatory effects of grape seed extract in hemodialysis patients; a pilot study. J. Renal. Inj. Prev. 2017;6:184-7.
-
32.
Abdelrahman RS. Protective effect of apocynin against gentamicin-induced nephrotoxicity in rats. Hum. Exp. Toxicol. 2018;37:27-37. [PubMed ID: 28116922].
-
33.
Balakumar P, WitnessKoe WE, Gan YS, JemayPuah SM, Kuganesswari S, Prajapati SK. Effects of pre and post-treatments with dipyridamole in gentamicin-induced acute nephrotoxicity in the rat. Regul. Toxicol. Pharmacol. 2017;84:35-44. [PubMed ID: 27993652].
-
34.
Hajihashemi S, Hamidizad Z, Rahbari A, Ghanbari F, Motealeghi ZA. Effects of Cobalamin (Vitamin B12) on Gentamicin Induced. Drug Res. 2017;67:710-8.
-
35.
Sugarman A, Brown RS, Silva P, Rosen S. Features of gentamicin nephrotoxicity and effect of concurrent cephalothin in the rat. Nephron. 1983;34:239-47. [PubMed ID: 6877460].
-
36.
Wagoner MP, Yang Y, McDuffie JE, Klapczynski M, Buck W, Cheatham L. Evaluation of temporal changes in urine-based metabolomic and kidney injury markers to detect compound induced acute kidney tubular toxicity in Beagle dogs. Curr. Top. Med. Chem. 2017;17:2767-80. [PubMed ID: 28714420].
-
37.
Uchino H, Fujishima J, Fukuoka K, Iwakiri T, Kamikuri A, Maeda H, Nakama K. Usefulness of urinary biomarkers for nephrotoxicity in cynomolgus monkeys treated with gentamicin, cisplatin, and puromycin aminonucleoside. J. Toxicol. Sci. 2017;42:629-40. [PubMed ID: 28904298].
-
38.
Gebremichael Y, Lu J, Shankaran H, Helmlinger G, Mettetal J, Hallow KM. Multiscale mathematical model of drug-induced proximal tubule injury: linking urinary biomarkers to epithelial cell injury and renal dysfunction. Toxicol. Sci. 2018;162:200-11. [PubMed ID: 29126144].
-
39.
Zhou X, Qu Z, Zhu C, Lin Z, Huo Y, Wang X, Wang J, Li B. Identification of urinary microRNA biomarkers for detection of gentamicin-induced acute kidney injury in rats. Regul. Toxicol. Pharmacol. 2016;78:78-84. [PubMed ID: 27074385].
-
40.
Moreira Galdino P, Nunes Alexandre L, Fernanda Pacheco L, de Souza Lino Junior R, de Paula JR, Rodrigues Pedrino G. Nephroprotective effect of Rudgea viburnoides (Cham ) Benth leaves on gentamicin-induced nephrotoxicity in rats. J. Ethnopharmacol. 2017;201:100-7. [PubMed ID: 28242383].
-
41.
Sadidi M, Bakhtiyari M, Alirezaei A. Effects of the Portulaca oleracea Extract on Gentamicin-Induced Nephrotoxicity in Male Rats. Iran Red Crescent Med J. 2019. In Press.
-
42.
Askari H, Haghi-Aminjan H, Hafizi SM, Alirezaei AH. The protective effects of Ocimum basilicum extract against gentamicin-induced nephrotoxicity in male rats; an anti-inflammatory, anti-oxidative and antiapoptotic action. Immunopathol Persa. 2019;5:e13.
-
43.
Fukuda Y, Eklof AC, Malmborg AS, Aperia A. Calcium supplementation and thyroid hormone protect against gentamicin-induced inhibition of proximal tubular Na+,K(+)-ATPase activity and other renal functional changes. Acta Physiol Scand. 1992;145:93-8. [PubMed ID: 1322021].
-
44.
Matsuda O, Beck FX, Dorge A, Thurau K. Electrolyte composition of renal tubular cells in gentamicin nephrotoxicity. Kidney Int. 1988;33:1107-12. [PubMed ID: 3404811].
-
45.
da Silva RM, Ko GM, Silva RF, Vieira LC, de Paula RV, Marumo JT. Essential Elements as Biomarkers of Acute Kidney Injury and Spontaneous Reversion. Biol. Trace Elem. Res. 2018;182:303-8. [PubMed ID: 28770412].
-
46.
Calvo FB, Junior DS, Rodrigues CJ, Krug FJ, Marumo JT, Schor N. Variation in the distribution of trace elements in renal cell carcinoma. Biol Trace Elem Res. 2009;130:107.
-
47.
Wang X, Chen H, Chang C, Jiang M, Wang X, Xu L. Study the therapeutic mechanism of Amomum compactum in gentamicin-induced acute kidney injury rat based on a back propagation neural network algorithm. Journal of chromatography B, Analyt Technol Biomed Life Sci. 2017;1040:81-8.
-
48.
Promsan S, Jaikumkao K, Pongchaidecha A, Chattipakorn N, Chatsudthipong V, Arjinajarn P. Pinocembrin attenuates gentamicin-induced nephrotoxicity in rats. Can J Physiol Pharmacol. 2016;94:808-18. [PubMed ID: 27245556].
-
49.
Park JS, Lim CJ, Bang OS, Kim NS. Ethanolic extract of Descurainia sophia seeds sensitizes A549 human lung cancer cells to TRAIL cytotoxicity by upregulating death receptors. BMC Complement Altern Med. 2016;16:115. [PubMed ID: 27039295].
-
50.
Oliveira CS, Rodrigues AM, Nogueira GB, Nascimento MA, Punaro GR, Higa EM. Moderate aerobic exercise on the recovery phase of gentamicin-induced acute kidney injury in rats. Life Sci. 2017;169:37-42. [PubMed ID: 27984075].
-
51.
Otunctemur A, Ozbek E, Dursun M, Sahin S, Besiroglu H, Ozsoy OD. Protective effect of hydrogen sulfide on gentamicin-induced renal injury. Ren Fail. 2014;36:925-31. [PubMed ID: 24679071].
-
52.
Teslariu O, Pasca AS, Mititelu-Tartau L, Schiriac CE, Gales C, Saftencu PM. The protective effects of zinc in experimental gentamicin induced acute renal failure in rats. J. Physiol. Pharmacol. 2016;67:751-7. [PubMed ID: 28011955].
-
53.
Stojiljkovic N, Ilic S, Veljkovic M, Todorovic J, Mladenovic M. alpha-Tocopherol Reduces Morphological Changes and Oxidative Stress during Gentamicin-Induced Acute Renal Failure. Bull Exp Biol Med. 2018;164:442-5. [PubMed ID: 29500805].
-
54.
Oliveira JFP, Cipullo JP, Burdmann EA. Nefrotoxicidade dos aminoglicosídeos. Braz J Cardiovasc Surg. 2006;21:444-52.
-
55.
Casanova AG, Vicente-Vicente L, Hernandez-Sanchez MT, Pescador M, Prieto M, Martinez-Salgado C. Key role of oxidative stress in animal models of aminoglycoside nephrotoxicity revealed by a systematic analysis of the antioxidant-to-nephroprotective correlation. Toxicology. 2017;385:10-7. [PubMed ID: 28472626].
-
56.
Kim BY, Lee J, Kim NS. Helveticoside is a biologically active component of the seed extract of Descurainia sophia and induces reciprocal gene regulation in A549 human lung cancer cells. BMC genomics. 2015;16:713. [PubMed ID: 26384484].
-
57.
Yi JM, Kim YA, Lee YJ, Bang OS, Kim NS. Effect of an ethanol extract of Descurainia sophia seeds on Phase I and II drug metabolizing enzymes and P-glycoprotein activity in-vitro. BMC Complement Altern Med. 2015;15:441. [PubMed ID: 26683337].
-
58.
Shirazi MK, Azarnezhad A, Abazari MF, Poorebrahim M, Ghoraeian P, Sanadgol N, Bokharaie H, Heydari S, Abbasi A, Kabiri S, Aleagha MN, Enderami SE, Dashtaki AS, Askari H. The role of nitric oxide signaling in renoprotective effects of hydrogen sulfide against chronic kidney disease in rats: Involvement of oxidative stress, autophagy and apoptosis. J cell physiol. 2019;234:11411-23. [PubMed ID: 30478901].
-
59.
Askari H, Abazari MF, Ghoraeian P, Torabinejad S, Nouri Aleagha M, Mirfallah Nassiri R. Ameliorative effects of hydrogen sulfide (NaHS) on chronic kidney disease-induced brain dysfunction in rats: implication on role of nitric oxide (NO) signaling. Metab Brain Dis. 2018;33:1945-54. [PubMed ID: 30090953].