Abstract
Keywords
Triazine Phenanthrene Cancer Antiproliferative effect Molecular docking
Introduction
Despite the availability of various cancer therapeutic agents, we continue to face complications and a lack of efficacy of available drugs. The Discovery of potential novel chemotherapeutics with improved efficacy and fewer side effects is an enormous challenge (1, 2).
DNA is still one of the most important targets for anticancer drug discovery (3, 4). DNA intercalating agents are likely to reversibly bind DNA and interfere with the essential functions of the cancer cells, such as transcription and replication (5-7). DNA organic intercalators mostly contain planar polycyclic aromatic rings (such as anthracene, acridine, anthraquinone and phenanthridine cores) helping the compound accommodate between base pairs, and also possess some polar functions providing hydrogen bond interactions with nucleic acids (7-9). Structures of some antitumor agents as DNA intercalators are depicted in Figure 1.
Additionally, B-cell lymphoma-2 (Bcl-2) family proteins are important regulators of apoptosis which are generally overexpressed in many cancer cells. Therefore, another trend for the treatment of cancer is finding inhibitors targeting the Bcl-2 proteins. The Bcl-2 family is divided into three groups based on their primary function 1) anti-apoptotic proteins (Bcl-2, Bcl-xL, Bcl-W, Mcl-1, Bfl-1/A1), 2) pro-apoptotic pore-formers (Bax, Bak, Bok) and 3) pro-apoptotic BH3-only proteins (Bad, Bid, Bik, Bim, Bmf, Hrk, Noxa, Puma, etc.). All Bcl-2 family proteins contain a BH3 domain; one of four BH domains involves in interactions between these proteins. The anti-apoptotic and pore-forming proteins contain all four BH domains (multi-BH domain proteins) and adopt a highly conserved tertiary structure forming a hydrophobic BH3 domain-binding groove that acts as a receptor for BH3 domains of other family members. The BH3-only proteins are subdivided into activator and sensitizer proteins, containing only the BH3 domain except for Bid are unstructured in solution (10-13).
1,2,4-Triazine derivatives have attracted great interest owing to their cytotoxic activities against different cancer cells (14, 15). In recent years, some studies on cytotoxic acenaphtho and phenanthrene-based fused scaffolds, as DNA intercalators and Bcl-2 inhibitors, have been performed (Figure 2). Zhang et al. reported that acenaphtho-pyrrole derivatives (such as compound I) demonstrated cytotoxic effects by DNA intercalation mechanism with an IC50 of 10-7–10-8 M against diverse cancer cell lines and introduced compound II as a promising Bcl-2 inhibitor (16-18). Additionally, Song et al. presented compound III with an acenaphtho-pyrrole skeleton as a potent pan-Bcl-2-inhibitor (19). Mohammadi et al. found out that 9-(alkylthio)-acenaphtho-1,2,4-triazine analogs, IV, exhibited moderate to good cytotoxic activities and suggested that these compounds could be potential Bcl-2 inhibitors by molecular docking analysis (20). Wang et al. evaluated the cytotoxicity and DNA binding property of some phenanthrene-imidazole derivatives and reported that compound V had a high binding affinity with calf thymus DNA (21).
This studydesigned a novel cytotoxic phenanthro-triazine-3-thiol derivatives with dual activity as DNA intercalators and Bcl-2 inhibitors. After synthesis, the cytotoxicity of the compounds was evaluated against cancer cell lines. Furthermore, to confirm the potential DNA intercalation and Bcl-2 inhibitory activities of phenanthro-triazine scaffolds, molecular docking analysis was performed.
Methods and materials
Chemistry
Apparatus and reagents
Melting points were measured on a hot stage apparatus (Electrothermal, Essex, UK) and were uncorrected. 1H spectra were recorded on Brucker 500 spectrometer, using TMS as an internal standard, and Chemical shifts (δ) are reported as ppm. IR spectra were obtained on a Perkin-Elmer spectrometer (KBr disk) (Perkin-Elmer, Waltham, MA). Mass spectra were recorded with an Agilent spectrometer (Agilent technologies 9575c inert MSD, USA). All commercially available solvents and reagents were of analytical grade and obtained from Merck and Aldrich.
Procedure for the synthesis of phenanthro [9,10-e][1,2,4]triazine-3-thiol (P1)
The phenanthrene-9,10-dione (4.16 g, 20 mmol) was added to 80 mL of acetic acid, and the mixture was heated to about 100 oC with stirring for about half an hour. Thiosemicarbazide (3.65 g, 40 mmol) was added, and the mixture was refluxed for about three hours. The reaction mixture cooled to room temperature and then poured into ice-cold water. The solid product was filtered and washed with cold water. Yield: 67.5%, Rf = 0.56 (EtOAc/ PetEther 1:1), mp: 122-124 °C. 1H NMR (500 M HZ, CDCl3): δH 14.65 ppm (s, 1H, NH), 8.74-8.39 (m, 2H, phenanthrene-H), 8.19-8.06 (m, 5H, phenanthrene-H), 7.78-7.37 (m, 9H, phenanthrene-H), 6.72 (s, 1H, SH). MS (EI) m/z (%) 263 (38.6), 235 (77.2), 176.1 (100), 150 (10.53). IR (KBr): υ = 3145 (CH-aromatic), 3413 (NH), 3242 cm-1 (SH).
General procedure for the synthesis of phenanthro[9,10-e][1,2,4]triazine-3-thiol derivatives (P2-P16)
The phenanthro[9,10-e][1,2,4]triazine-3-thiol (0.263 g, 1 mmol) was added to a stirred hot ethanolic solution of sodium hydroxide (0.1 M). After cooling to room temperature, corresponding alkyl/benzyl halide was added, and the mixture was stirred for about 2-24 h at 25 °C. Completion of the reaction was checked by thin-layer chromatography (TLC). The solvent was reduced under vacuum; the precipitate was filtered and washed with ethanol and cold water. Finally, the dried precipitate was purified by recrystallization or chromatography to give the final pure derivative (Table 1).
3-(methylthio)phenanthro[9,10-e][1,2,4]tri-azine (P2)
Compound P2 was prepared by the described procedure using methyl iodide (3 mmol, 0.19 mL) and the obtained precipitate was recrystallized from ethyl acetate. Yield: 36.1%, Rf = 0.67 (EtOAc/ PetEther 1:1), mp: 157-160 °C. 1H NMR (500 M HZ, CDCl3): δH 9.36 (d, 1H, J = 7.2 Hz, phenanthrene-H), 9.15 (d, 1H, J = 7.6 Hz, phenanthrene-H), 8.53 (s, 2H, phenanthrene-H), 7.88-7.72 (m, 4H, phenanthrene-H), 2.89 ppm (s, 3H, CH3). MS (EI) m/z (%) 277.1 (43.9), 249.2 (79), 235 (46.5), 203.1 (7), 176.1 (100), 150.1 (14). IR (KBr): υ = 3060 (CH-aromatic), 2927 cm-1 (CH-aliphatic).
3-(ethylthio)phenanthro[9,10-e][1,2,4]tri-azine (P3)
Compound P3 was prepared by the described procedure using ethyl iodide (3 mmol, 0.24 mL) and the obtained precipitate was recrystallized from ethyl acetate. Yield: 54.91%, Rf = 0.67 (EtOAc/ PetEther 1:1), mp: 131-133 °C. 1H NMR (500 M HZ, CDCl3): δH 9.4 (d, 1H, J = 7.5 Hz, phenanthrene-H), 9.2 (d, 1H, J = 7.5 Hz, phenanthrene-H), 8.6 (t, 2H, J = 8 Hz, phenanthrene-H), 7.92-7.74 (m, 4H, phenanthrene-H), 3.5 (q, 2H, J = 7.2 Hz, CH2-CH3), 1.6 ppm (t, 3H, J = 7.2 Hz, CH2-CH3). MS (EI) m/z (%) 291.1 (40.35), 263.1 (28), 235.1 (100), 203.1 (10), 176.1 (75.44), 150.1 (10.5). IR (KBr): υ = 3065 (CH-aromatic), 2928 cm-1 (CH-aliphatic).
3-(propylthio)phenanthro[9,10-e][1,2,4]tri-azine (P4)
Compound P4 was prepared by the described procedure using 1-bromopropane (3 mmol, 0.29 mL), the obtained precipitate was dissolved in appropriate solvent and purified using silica gel column chromatography with petroleum ether-ethyl acetate as the mobile phase. Yield: 49.12%, Rf = 0.69 (EtOAc/ PetEther 3:7), mp: 118-120 °C. 1H NMR (500 MHZ, CDCl3): δH 9.37 (d, 1H, J = 7.0 HZ, phenanthrene-H), 9.15 (d, 1H, J = 7.6 HZ, phenanthrene-H), 8.55 (t, 2H, J = 7.9 HZ, phenanthrene-H), 7.90-7.30 (m, 4H, phenanthrene-H), 3.48 (t, 2H, J = 7.2 HZ, CH2-CH2-CH3), 2.0 (sext, 2H, -CH2-CH2-CH3), 1.22 ppm (t, 3H, J = 7.3 HZ, -CH2-CH2-CH3). MS (EI) m/z (%) 305.1 (28), 279.1 (36.9), 263.1 (47.4), 235.1 (100), 203.1 (14), 176.1 (77.2), 150.1 (14). IR (KBr): υ = 3071 (CH-aromatic), 2960, 2929 cm-1 (CH-aliphatic).
3-(butylthio)phenanthro[9,10-e][1,2,4]tri-azine (P5)
Compound P5 was prepared by the described procedure using 1-bromobutane (3 mmol, 0.29 mL), the obtained precipitate was purified using silica gel column chromatography with petroleum ether-ethyl acetate as the mobile phase and then recrystallized from chloroform. Yield: 14%, Rf = 0.66 (EtOAc/ PetEther 3:7), mp: 81-83 °C. 1H NMR (500 MHZ, CDCl3): δH 9.31 (d, 1H, J = 7.4 HZ, phenanthrene-H), 9.06 (d, 1H, J = 7.4 HZ, phenanthrene-H), 8.47 (m, 2H, phenanthrene-H), 7.85-7.67 (m, 4H, phenanthrene-H), 3.48 (t, 2H, J = 7.3 HZ, CH2-(CH2)2-CH3), 1.96 (pent, 2H, CH2-CH2-CH2-CH3), 1.66 (sext, 2H, CH2-CH2-CH2-CH3), 1.07 ppm (t, 3H, J = 7.3 HZ, CH2-CH2-CH2-CH3). MS (EI) m/z (%) 319.2 (50.9), 291.1 (19.3), 263.1 (52.6), 235.1 (100), 203.1 (64.9), 176.1 (75.4), 150.1 (14). IR (KBr): υ = 3071 (CH-aromatic), 2963 cm-1 (CH-aliphatic).
3-(isobutylthio)phenanthro[9,10-e][1,2,4]tri-azine (P6)
Compound P6 was prepared by the described procedure using 1-bromopropane2-methylpropan (3 mmol, 0.33 ml), the obtained precipitate was purified using silica gel column chromatography with petroleum ether-ethyl acetate as the mobile phase and then recrystallized from chloroform. Yield: 17.8%, Rf = 0.67 (EtOAc/ PetEther 2:8), mp: 93-96 °C. 1H NMR (500 MHZ, CDCl3): δH 9.4 (d, 1H, J = 7.9 HZ, phenanthrene-H), 9.2 (d, 1H, J = 7.9 Hz, phenanthrene-H), 8.6 (t, 2H, J = 9 HZ, phenanthrene-H), 7.93-7.75 (m, 4H, phenanthrene-H), 3.4 (d, 2H, J = 6.8 HZ, CH2(CH(CH3)2)), 2.3-2.1 (m , 1H, CH2(CH(CH3)2)), 1.23 ppm (d, 6H, J = 6.8 Hz, (CH3)2). MS (EI) m/z (%) 319.2 (10.5), 291.1 (17.5), 263.1 (75.4), 235.1 (100), 203.1 (10.5), 176.1 (49), 150.1 (8.8). IR (KBr): υ = 3060 (CH-aromatic), 2963, 2926 cm-1 (CH-aliphatic).
3-(phenanthro[9,10-e][1,2,4]triazin-3-ylthio)propanenitrile (P7)
Compound P7 was prepared by the described procedure using 3-bromopropionitrile (3 mmol, 0.25 mL) and the obtained precipitate was recrystallized from ethyl acetate. Yield: 25.3%, Rf = 0.27 (EtOAc/ PetEther 3:7), mp: 128-130 °C. 1H NMR (500 MHZ, CDCl3): δH 9.4 (d, 1H, J = 7.8 HZ, phenanthrene-H), 9.17 (d, 1H, J = 7.8 HZ, phenanthrene-H), 8.62 (t, 2H, J = 8.6 HZ, phenanthrene-H), 7.93-7.75 (m, 4H, phenanthrene-H), 3.76 (t , 2H, J = 7.2 HZ, CH2-CH2-CN), 3.15 ppm (t, 2H, J = 7.2 HZ, CH2-CH2-CN). MS (EI) m/z (%) 316.2 (33.3), 288.1 (100), 260.1 (17.5), 235.1 (21.9), 201.1 (4.4), 176.1 (73.7), 150.1 (12.3). IR (KBr): υ = 3064 (CH-aromatic), 2923 (CH-aliphatic), 2245 cm-1 (CN).
3-((2-methoxyethyl)thio)phenanthro[9,10-e][1,2,4]triazine (P8)
Compound P8 was prepared by the described procedure using 2-methoxyethyl chloride (3 mmol, 0.29 ml), the obtained precipitate was purified using silica gel column chromatography with petroleum ether-ethyl acetate as the mobile phase and then recrystallized from ethyl acetate. Yield: 16.2%, Rf = 0.49 (EtOAc/ PetEther 3:7), mp: 100-102 °C. 1H NMR (500 MHZ, CDCl3): δH 9.25 (d, 1H, J = 7.8 HZ, phenanthrene-H), 9.0 (d, 1H, J = 7.8 HZ, phenanthrene-H), 8.42-8.39 (m, 2H, phenanthrene-H), 7.82-7.63 (m, 4H, phenanthrene-H), 3.91 (t, 2H, J = 6.5 Hz, CH2-CH2-OCH3), 3.69 (t, 2H, J = 6.5 Hz, CH2-CH2-OCH3), 3.5 ppm (s, 3H, OCH3). MS (EI) m/z (%) 321 (10.5), 293 (7), 263 (98.3), 23 (100), 203 (73.7), 176 (63.2), 150 (17.5). IR (KBr): υ = 3065 (CH-aromatic), 2962, 2927 cm-1 (CH-aliphatic).
3-(cyclopentylthio)phenanthro[9,10-e][1,2,4]triazine (P9)
Compound P9 was prepared by the described procedure using cyclopentyl bromide (3 mmol, 0.32 mL), the obtained precipitate was purified using silica gel column chromatography with petroleum ether-ethyl acetate as the mobile phase and then recrystallized from ethyl acetate. Yield: 11.6%, Rf = 0.73 (EtOAc/ PetEther 2:8), mp: 170-172 °C. 1H NMR (500 MHZ, CDCl3): δH 9.34 (d, 1H, J = 7.8 HZ, phenanthrene-H), 9.11 (d, 1H, J = 8 HZ, phenanthrene-H), 8.50 (t, 2H, J = 7.7 HZ, phenanthrene-H), 7.87-7.69 (m, 4H, phenanthrene-H), 4.41 (quint, 1H, Hₐ-cyclopenthyl), 2.51-2.46 (m, 2H, H-cyclopenthyl), 1.95-1.82 ppm (m, 6H, H-cyclopenthyl). MS (EI) m/z (%) 331.1 (29.9), 303.1 (22.8), 279.1 (8.8) 262.1 (29.9), 235. 1(100), 208.1 (91.2), 176.1 (63.2), 149 (52.6). IR (KBr): υ = 3032 (CH-aromatic), 2959 cm-1 (CH-aliphatic).
3-(cyclohexylthio)phenanthro[9,10-e][1,2,4]triazine (P10)
Compound P10 was prepared by the described procedure using cyclohexyl bromide (3 mmol, 0.37 mL), the obtained precipitate was purified using silica gel column chromatography with petroleum ether-ethyl acetate as the mobile phase and then recrystallized from ethyl acetate. Yield: 15.8%, Rf = 0.49 (EtOAc/ PetEther 2:8), mp: 144-147 °C. 1H NMR (300 MHZ, CDCl3): δH 9.27 (d, 1H, J = 6 HZ, phenanthrene-H), 9.05 (d, 1H, J = 6 HZ, phenanthrene-H), 8.45 (t, 2H, J = 6 HZ, phenanthrene-H), 7.78-7.61 (m, 4H, phenanthrene-H), 4.13 (quint, 1H, Hₐ-cyclohexyl), 1.84-1.80 (m, 2H, H-cyclohexyl), 1.65-1.50 ppm (m, 4H, H-cyclohexyl), 1.37-1.18 ppm (m, 4H, H-cyclohexyl). MS (EI) m/z (%) 345.2 (28), 317.2 (14), 264.1 (31.6), 235.1 (100), 203.1 (17.5), 176.1 (42), 150.1 (7). IR (KBr): υ = 3060 (CH-aromatic), 2962, 2924 cm-1 (CH-aliphatic).
3-(benzylthio)phenanthro[9,10-e][1,2,4]tri-azine (P11)
Compound P11 was prepared by the described procedure using benzyl bromide (2 mmol, 0.340 g), the obtained precipitate was purified using silica gel column chromatography with petroleum ether-ethyl acetate as the mobile phase and then recrystallized from ethyl acetate. Yield: 41.4%, Rf = 0.65 (EtOAc/PetEther 1:4), mp: 167-170 °C. 1H NMR (300 M Hz, CDCl3): δH 9.122 (d, 1H, J = 5.7 Hz, phenanthrene-H), 8.887 (d, 1H, J = 7.2 Hz, phenanthrene-H), 8.275-8.245 (m, 2H, Ar-H), 7.655-7.172 (m, 9H, Ar-H), 4.608 ppm (s, 2H, CH2 -Phenyl). MS (EI) m/z (%) 353.1 (58.2), 190.1 (100), 91.1 (61.8). IR (KBr): ν 3025 (CH-aromatic), 2963 cm-1 (CH-aliphatic).
3-((4-bromobenzyl)thio)phenanthro[9,10-e][1,2,4]triazine (P12)
Compound P12 was prepared by the described procedure using 4-bromobenzyl bromide (2 mmol, 0.500 g), the obtained precipitate was purified using silica gel column chromatography with petroleum ether-ethyl acetate as the mobile phase and then recrystallized from ethyl acetate. Yield: 48.8%, Rf = 0.62 (EtOAc/PetEther 1:4), mp: 171-175 °C. 1H NMR (300 M HZ, CDCl3): δH 9.304-9.273 (dd, 1H, J = 7.5, 1.8 Hz, phenanthrene-H), 9.065 (d, 1H, J = 8.1 Hz, phenanthrene-H), 8.508-8.461 (t, 2H, J = 7.2 Hz, Ar-H), 7.841-7.627 (m, 4H, Ar-H), 7.389 (s, 4H, Ar-H), 4.598 ppm (s, 2H, CH2 -Phenyl). MS (EI) m/z (%) 90.1 (18.3), 190.1 (100), 170.9 (57.9), 433.0 (56.2). IR (KBr): ν 3062 (CH-aromatic), 2960 cm-1 (CH-aliphatic).
3-((4-nitrobenzyl)thio)phenanthro[9,10-e][1,2,4]triazine (P13)
Compound P13 was prepared by the described procedure using 4-nitrobenzyl bromide (2 mmol, 0.430 g). The obtained precipitate was purified using silica gel column chromatography with petroleum ether-ethyl acetate as the mobile phase and then recrystallized from ethyl acetate. Yield: 16.6%, Rf = 0.46 (EtOAc/PetEther 1:4), mp: 198-202 °C.1H NMR (300 M HZ, CDCl3): δH 9.356 (d, 1H, J = 7.8 Hz, phenanthrene-H), 9.105 (d, 1H, J = 7.8 Hz, phenanthrene-H), 8.589-8.540 (t, 2H, J = 7.2 Hz, Ar-H), 8.196 (d, 2H, J = 8.4 Hz, Ar-H), 7.896-7.702 (m, 6H, Ar-H), 4.787 ppm (s, 2H, CH2 -Phenyl). MS (EI) m/z (%) 398.1 (44.3), 322.3 (66.9), 190.0 (100). IR (KBr): ν 3076 (CH-aromatic), 2923 (CH-aliphatic), 1524, 1354 (ArNO2), 872 cm-1 (C-NO2).
3-((4-fluorobenzyl)thio)phenanthro[9,10-e][1,2,4]triazine (P14)
Compound P14 was prepared by the described procedure using 4-fluorobenzyl chloride (2 mmol, 0.288 g), the obtained precipitate was purified using silica gel column chromatography with petroleum ether-ethyl acetate as the mobile phase and then recrystallized from ethyl acetate. Yield: 61.2%, Rf = 0.60 (EtOAc/PetEther 1:4), mp: 157-160 °C. 1H NMR (300 M HZ, CDCl3): δH 9.171-9.141 (dd, 1H, J = 7.2, 1.5 Hz, phenanthrene-H), 8.910 (d, 1H, J = 7.8 Hz, phenanthrene-H), 8.326-8.288 (m, 2H, Ar-H), 7.692-7.438 (m, 6H, Ar-H), 6.976-6.919 (m, 2H, Ar-H), 4.581 ppm (s, 2H, CH2 -Phenyl). MS (EI) m/z (%) 371.1 (79.3), 343.1 (35.1), 190.1 (73.9), 109.0 (100) IR (KBr): ν 3070 (CH-aromatic), 2963 cm-1 (CH-aliphatic).
3-((4-methylbenzyl)thio)phenanthro[9,10-e][1,2,4]triazine (P15)
Compound P15 was prepared by the described procedure using 4-methylbenzyl chloride (2 mmol, 0.280 g), the obtained precipitate was purified using silica gel column chromatography with petroleum ether-ethyl acetate as the mobile phase and then recrystallized from ethyl acetate. Yield: 34.7%, Rf = 0.65 (EtOAc/PetEther 1:4), mp: 138-141 °C. 1H NMR (300 M HZ, CDCl3): δH 9.013-8.990 (m, 1H, phenanthrene-H), 8.766-8.724 (t, 1H, J = 7.2 Hz, phenanthrene-H), 8.112-8.085 (m, 2H, Ar-H), 7.574-7.409 (m, 2H, Ar-H), 4.561 & 4.526 (2s, 2H, CH2-Phenyl), 2.227 ppm (s, 3H, CH3-Phenyl). MS (EI) m/z (%) 91.1 (32.1), 105.5 (100), 190.1 (96.2), 367.1 (89.1) IR (KBr): ν 3074 (CH-aromatic), 2926 cm-1 (CH-aliphatic).
3-((4-methoxybenzyl)thio)phenanthro[9,10-e][1,2,4]triazine (P16)
Compound P16 was prepared by the described procedure using 4-methoxybenzyl chloride (2 mmol, 0.312 g), the obtained precipitate was purified using silica gel column chromatography with petroleum ether-ethyl acetate as the mobile phase and then recrystallized from ethyl acetate. Yield: 31.2%, Rf = 0.57 (EtOAc/PetEther 1:4), mp: 152-154 °C. 1H NMR (300 M Hz, CDCl3): δH 9.230-9.199 (dd, 1H, J = 6.9, 1.5 Hz, phenanthrene-H), 9.006-8.975 (dd, 1H, J = 8.1, 1.2 Hz, phenanthrene-H), 8.371-8.334 (m, 2H, Ar-H), 7.777-7.572 (m, 4H, Ar-H), 7.501-7.472 (d, 2H, J = 8.7 Hz, ArH), 6.891-6.862 (d, 2H, J = 8.7 Hz, Ar-H), 4.646 (s, 2H, CH2-Phenyl), 3.777 ppm (s, 2H, O-CH3). MS (EI) m/z (%) 383.1 (44.8), 190.0 (31.1), 121.0 (100). IR (KBr): ν 3068 (CH-aromatic), 2952 (CH-aliphatic), 1249, 1045 cm-1 (C-O-C).
Biological section
Cell lines and cell culture
This study used two cell lines, including MOLT-4 (human acute lymphoblastic leukemia) and MCF-7 (human breast adenocarcinoma). MOLT-4 was grown in suspension, while MCF-7 was grown in monolayer culture. The MOLT-4 cell line was purchased from the National Cell Bank of Iran, Pasteur Institute, Tehran, Iran, while MCF-7 cells were obtained from the Iranian Biological Resource Center (IBRC), Tehran, Iran. The cells were cultured in RPMI 1640 supplemented with 10% fetal bovine serum (FBS) and 100 units/mL penicillin-G and 100 µg/mL streptomycin at 37 °C in humidified air containing 5% CO2. RPMI-1640, FBS, trypsin and phosphate-buffered saline (PBS) were purchased from Biosera (Ringmer, UK). 3-(4,5-Dimethylthiazol- 2-yl)-2,5-diphenyltetrazolium bromide (MTT) was obtained from Sigma-Aldrich (St. Louis, MO, USA). Standard chemotherapeutic agents, dimethylsulfoxide (DMSO) and penicillin/streptomycin were purchased from EBEWE Pharma (Unterach, Netherlands), Merck (Darmstadt, Germany) and Invitrogen (San Diego, CA, USA), respectively.
MTT assay
Cells were seeded into 96-well plates with a density of 5×104 cells/ml (100 µl in each well) and incubated for 24 h at 37 °C. Afterward, the synthesized compounds were added to each well (in triplicates) at three different concentrations. Doxorubicin and cisplatin were used as positive controls. The plates were further incubated for 72 h, and then 80 μL of the medium was removed, and the same volume of an MTT solution was added at a final concentration of 0.5 mg/mL. Plates were incubated for 4 h at 37 °C to allow the formazan crystals to be formed. Then,200 μL DMSO was added to each well to dissolve the formazan crystals. Absorbance was determined at 570 nm with background correction at 655 nm using a Bio-Rad microplate reader (Model 680). The percentage of viability inhibition compared to control was assessed for each concentration of the compound, and IC50 values were computed with CurveExpert software version 1.34 for Windows.
Molecular docking analysis
Computational docking, an extremely useful tool to achieve deep insight into drug-target interactions, has played a crucial role in drug discovery. The synthesized derivatives were subjected to molecular docking studies using AutoDock Tools and AutoDock software in this study.
Molecular docking studies with DNA
The X-ray crystal structure of B-DNA (PDB ID: 1Z3F) was obtained from the Protein Data Bank. The ligand structures were drawn and minimized under Semi-empirical AM1 methods using HyperChem 7. Molecular docking studies were done using the AutoDock Tools 1.5.7rc1 and AutoDock 4.2.5.1 docking programs. The energy calculations were made using genetic algorithms (LGA) with the number of GA runs set to 100.
Molecular docking studies with Bcl-2 protein
The crystal structure of Bcl-2 (PDB ID: 3ZLN) was acquired from RCSB Protein Data Bank (http://www.rcsb.org).Innate ligand and waters were removed, hydrogen atoms were added, non-polar hydrogens were merged, and Gasteiger charges were added using AutoDock Tools to prepare the protein for docking. 3D structures of ligands were sketched and minimized under Molecular Mechanics MM+ and then Semi-empirical AM1 methods using HyperChem software. A grid of 60, 60, and 60 points in x = -17.788, y = -12.682 and z = 10.891 directions with 0.375 Å grid spacing was built. The docking process was performed by AutoDock 4.2. To find the conformers with the lowest binding energy, Lamarckian genetic algorithm (LGA) was used, and the number of GA runs was set to 100. The other parameters were left at program default values. Docking procedure validity was examined by re-docking the structure co-crystallized ligand into the receptor (self-docking) using the protocol mentioned above.
Results and Discussion
Design strategy
The target compounds were designed by a molecular hybridization strategy based on the structures of some DNA intercalators and Bcl-2 inhibitors (I-V and ethidium illustrated in Figure 2) previously reported in the literature. As shown in Figure 2, the molecular hybridization of phenanthrene and 1,2,4-triazine rings yielded the scaffold which contains three parts: 1) a phenanthrene scaffold as a planar polycyclic central core to intercalate between DNA base-pairs and to provide π-π interactions with the key amino acids in the active site of Bcl-2, 2) a 1,2,4-triazine ring which is fused to phenanthrene as a site to establish H-bond interactions with DNA nucleic acids and Bcl-2 target and 3) a variety of benzyl-thio and alkyl-thio side chains to improve the interactions and promote the physicochemical properties. To the best of our knowledge, there have been no literature reports regarding fused phenanthrene-triazine skeleton bearing alkyl/benzyl-thio substitutions as cytotoxic agents with potential dual DNA intercalating and Bcl-2 inhibitory activity so far.
Synthesis
The synthetic routes of P1-P16 compounds are outlined in Scheme 1 and Table 1. The reaction of phenanthrene-9,10-dione in refluxing acetic acid with thiosemicarbazide afforded phenanthrotriazine-3-thiol (P1). Substitution reaction of different halides with intermediate P1 in an ethanolic solution of sodium hydroxide furnished phenanthro-triazine-3-thiol derivatives (P2-P16). The chemical structures were characterized by IR, MS, 1H NMR analysis.
Cytotoxicity
The compounds were tested for their in-vitro cytotoxicity against MOLT-4 (human acute lymphoblastic leukemia) and MCF-7 (human breast adenocarcinoma) cell lines using the MTT assay. MOLT-4 and MCF-7 are frequently used cell lines for screening anticancer agents included in the NCI-60 panel of cell lines suggested by the National Cancer Institute for in-vitro tests. In addition, these cell lines express easily detectable levels of Bcl-2 and Bcl-xl and hence are valuable tools for the study of the inhibitors of these targets (22-24). The chemotherapeutic drugs doxorubicin and cisplatin were used as the positive controls. The results of the MTT assay expressed as IC50 values of synthesized compounds are listed in Table 2. The phenanthro-triazine-3-thiol derivative P1 exhibited considerable cytotoxicity against MOLT-4 with an IC50 value of 7.1 ± 1.1 μM and also showed a moderate effect against MCF-7 cells with an IC50 value of 26.6 ± 1.6 μM. P11 (bearing phenyl substitution) demonstrated good cytotoxic activities against MCF-7 with IC50 values of 15.4 ± 2.9, while P15 (bearing 4-methylphenyl substitution) had an IC50 of 19.4 ± 2.5 μM against MOLT-4 cells. Compounds P7, P8 and P14, containing propanenitrile, 2-methoxyethyl, and 4-fluorophenyl moieties, respectively, exhibited moderate cytotoxic activities against MCF-7 cells with IC50 values in the range of 34.3-39.7 μM.
Molecular docking studies
Molecular docking studies with DNA duplex with the sequence of d(CGATCG)2
A molecular docking study was employed to identify the binding interactions and estimate the binding affinity of the most active derivatives to DNA duplex with sequence d(CGATCG)2. 3D representations of docking results of compounds P1, P11 and P15 with DNA are depicted in Figures 3-5, and the dock poses of the compounds are illustrated in Figure 6. Considering the values of free energies of binding mentioned in Table 3, it could be deduced that the compounds were properly accommodated between the DNA base pairs. Moreover, as shown in Figures 3-6, the ligands unwound DNA by establishing some interactions with the GC base pairs. The molecular docking analysis revealed that in all three derivatives, the phenantrene-triazine core has contributed in hydrophobic and Pi-Pi interactions with the base pairs. Additionally, in P1, the hydrogen atom attached to the sulfur atom provided a hydrogen bond interaction with deoxyguanosine 6 ( DG6) while P15, 4-methylphenyl substitution established an additional Pi-Pi interaction with deoxycytidine 5 (DC5).
Molecular docking studies with Bcl-2 protein
In-silico molecular docking was performed to gain information on the binding modes of the most active compounds (P1, P11, and P15) in the Bcl-2 active site. All the three phenanthro-triazine-3-thiol derivatives were well accommodated into the active site of Bcl-2 (as depicted in Figure 7) and showed good binding energies ranging from -9.96 to -12.58 kcal/mol. The Bcl-2 residues proposed to interact with the ligand molecules are represented in Figures 8-10. The corresponding free energies of binding and the molecular interactions are summarized in Table 4. Molecular docking analysis revealed that the derivatives might inhibit Bcl-2 protein mainly via Pi interactions. The tow types of Pi interaction including Pi-alkyl and Pi-Pi stacking as well as hydrogen bonding mainly govern the binding modes of phenanthro-triazine-3-thiol derivatives in the binding site of the protein. In the case of P1, two hydrogen bonds were observed between the hydrogen attached to sulfur and Ser106 and Arg102 residues. Moreover, the P1 derivative interacts with residues Leu108, Arg102, Arg139, Ala142, Leu130, and especially Phe105 through Pi-alkyl, Pi-Pi stacking and Pi-sigma with its triazine and phenanthrene moieties. The same interactions occured in the P11 and P15 derivatives. By comparing the residues which are responsible to free energy binding of three derivatives, it is understandable that the phenyl moiety in the P11 derivative interacting with Leu108, Arg102, Leu130 and Phe146 through Pi-alkyl and Pi-Pi stacking interactions can make a drastic impact on the binding energy, ΔG, of that ligand (-8.86 versus -10.98 kcal/mol). It is also referred that the same trend is predicted for P15 by forming Pi-Pi stacking, Pi-alkyl and alkyl-alkyl interactions between its methylphenyl moiety and Phe146, Leu130 and Ala149 which lead to better binding free energy (-8.86 versus -11.73 kcal/mol). By analyzing the contributed residues, it can be concluded that hydrophobic residues primarily contribute to the binding of three derivatives with Bcl-2. Result for re-docking the co-crystallized ligand into the receptor (self-docking) is depicted in Figure 11 and the interactions are listed in Table 4. The root mean square deviation (RMSD) between the best pose of co-crystallized ligand docked into the binding site and the one in the crystal structure was 0.61 Å. In the case of the innate ligand, hydrogen bonding and charg-charg interactions played the key role, as the carboxylate moiety established strong interactions with Asn136 and Arg139 residues. İn addition, benzothiazol and hydrazin moieties contributed in forming two other hydrogen bonds with Leu108 and Ser106. Resıdues Ala149, Phe146, Leu108, Leu130, Phe105 and Arg139 involved in hydrophobic interactions, as seen in docking of compounds P1, P11 and P15.
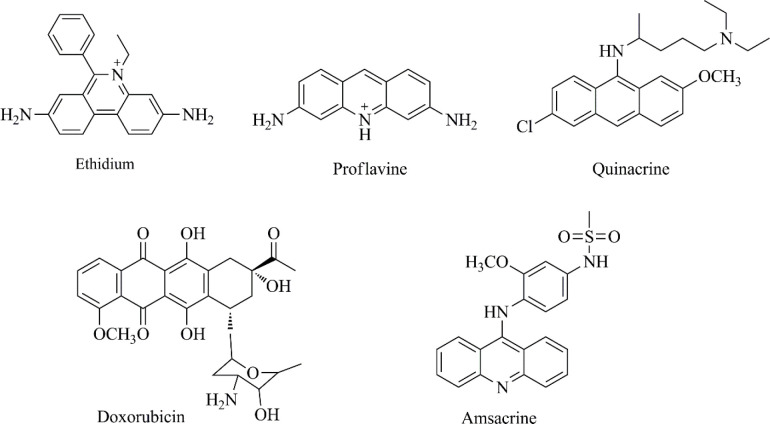
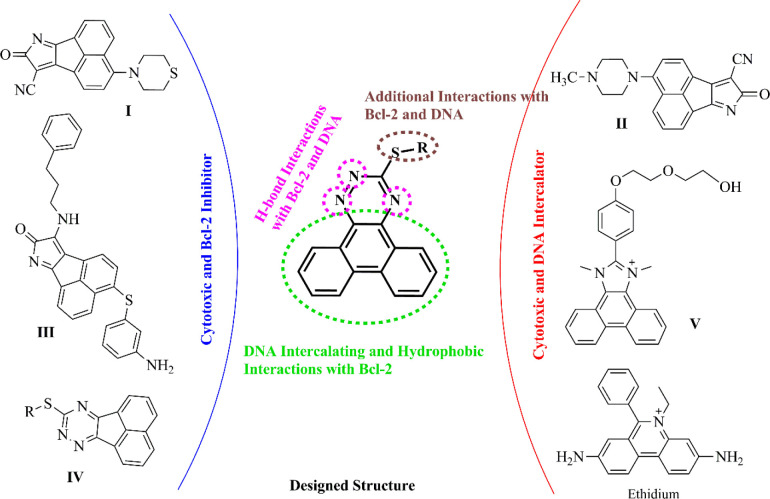
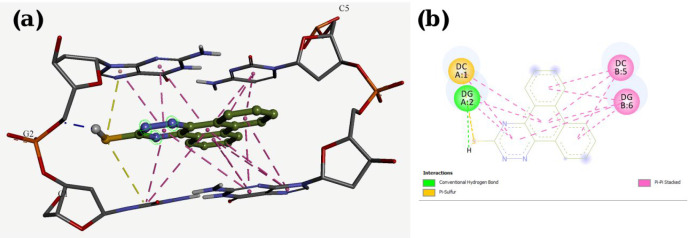
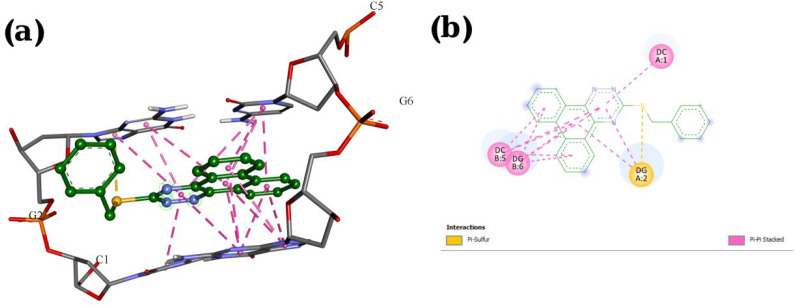
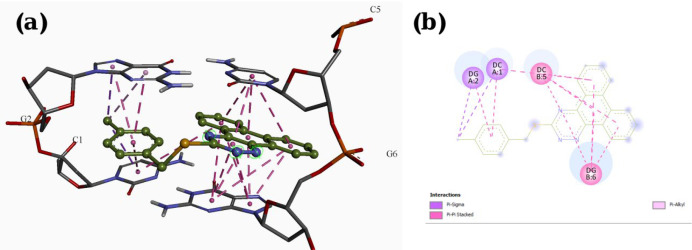
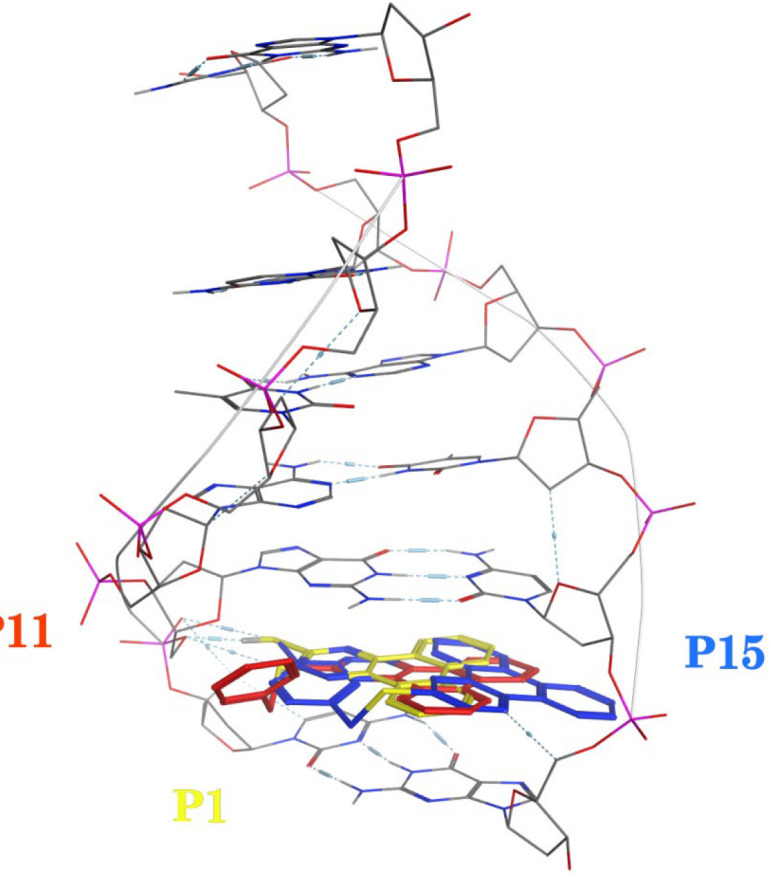
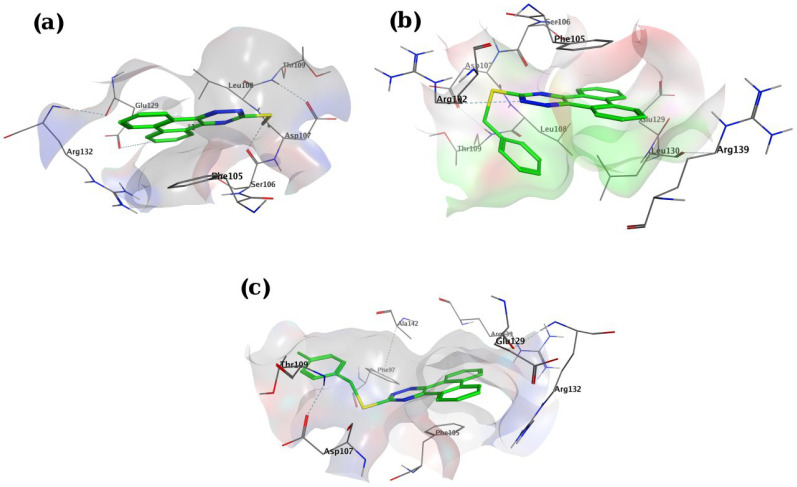
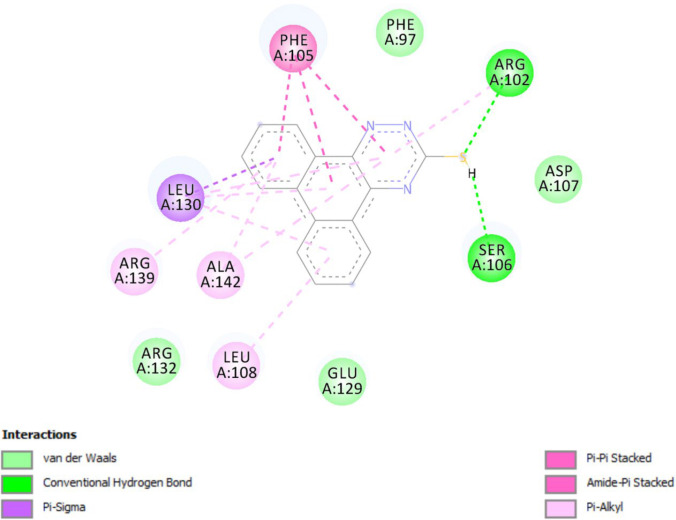
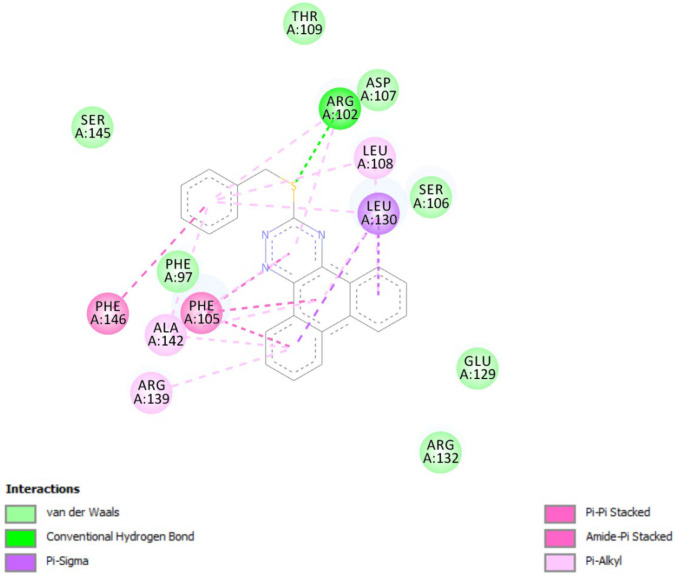
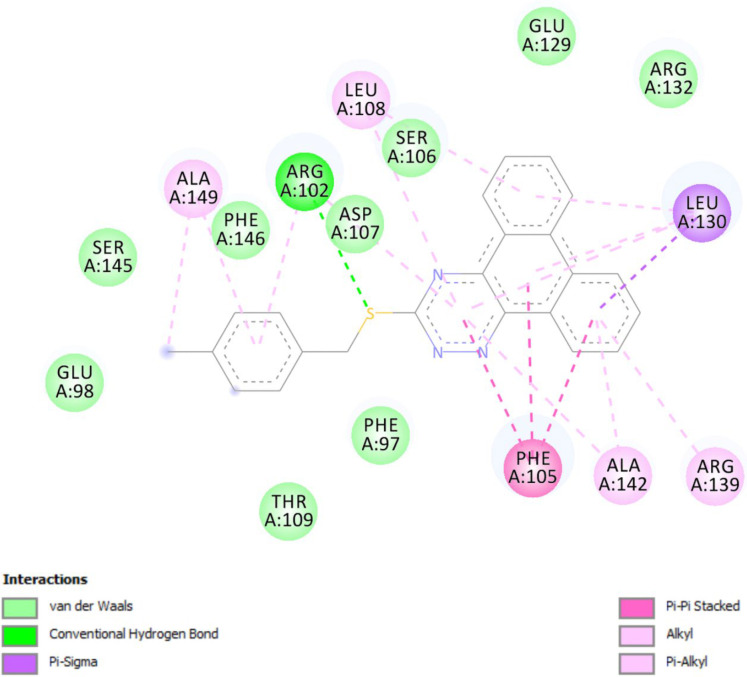
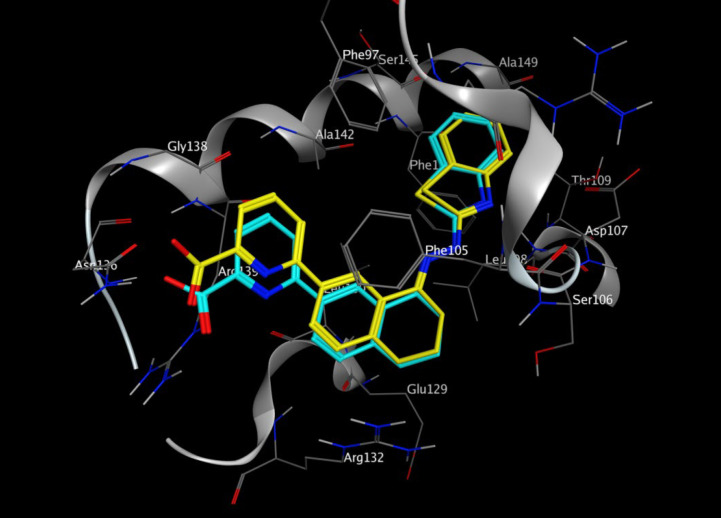
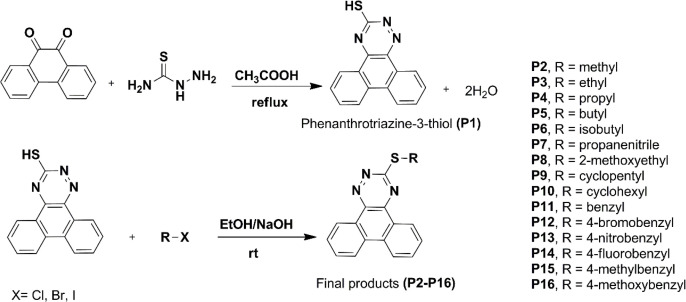
Chemical structure of synthesized compounds P1-P16
Cytotoxic activity of synthesized compounds against human cancer cell lines evaluated by MTT reduction assay
Compound | IC50 (μM) ± SEMa | IC50 (μM) ± SEM | |||
---|---|---|---|---|---|
MOLT-4 | MCF-7 | Compound | MOLT-4 | MCF-7 | |
P1 | 7.1 ± 1.1 | 26.6 ± 1.6 | P10 | ˃ 50 | ˃ 50 |
P2 | ˃ 25 | ˃ 10 | P11 | ˃ 25 | 15.4 ± 2.9 |
P3 | ˃ 10 | ˃ 10 | P12 | ˃ 10 | ˃ 10 |
P4 | ˃ 100 | ˃ 100 | P13 | ˃ 50 | ˃ 50 |
P5 | ˃ 100 | ˃ 100 | P14 | 25 | 34.3 ± 2.1 |
P6 | ˃ 50 | ˃ 50 | P15 | 19.4 ± 2.5 | ˃ 25 |
P7 | ˃ 50 | 39.7 ± 6.1 | P16 | ˃ 25 | ˃ 25 |
P8 | ˃ 50 | 33.5 ± 8.8 | Doxorubicin | 0.016 ± 0.004 | 0.050 ± 0.008 |
P9 | ˃ 25 | ˃ 100 | Cisplatin | 3.6 ± 0.6 | 9.5 ± 1.4 |
Docking results of compounds with DNA (PDB ID: 1Z3F).
Distance (Å) | Nucleic acid | Moiety of ligand | Interactions | Ki | ΔG (kcal/mol) | Compound |
DC1, DC2DG5, DG6 | Phenanthrene | π -π | 152.16 | -9.19 | P1 | |
DC1, DC2DG5, DG6 | Phenanthrene | π -π | 118.64 | -9.45 | P2 | |
DC1, DC2DG5, DG6 | Phenanthrene | π -π | 105.14 | -9.52 | P3 | |
3.066 | DC1, DC2DG5, DG6 | S | H-bond, | 120.09 | -9.44 | P4 |
DC1, DC2DG5, DG6 | Phenanthrene | π -π | 71.09 | -9.75 | P5 | |
DC1, DC2DG5, DG6 | Phenanthrene | π -π | 68.85 | -9.77 | P6 | |
2.760 | DC1, DC2DG5, DG6 | S | H-bond, | 86.47 | -9.64 | P7 |
2.775 | DC1, DC2DG5, DG6 | O | H-bond, | 110.88 | -9.49 | P8 |
DC1, DC2DG5, DG6 | Phenanthrene | π –π | 20.23 | -10.50 | P9 | |
2.760 | DC1, DC2DG5, DG6, | N | H-bond, | 25.23 | -10.37 | P10 |
DC1, DC2DG2, DG6 | Phenanthrene | π –π | 94.52 | -9.58 | P11 | |
DC1, DC2DG5, DG6, | Phenanthrene | π –π | 9.11 | -10.97 | P12 | |
DC1, DC2DG5, DG6, | Phenanthrene | π –π | 52.36 | -9.93 | P13 | |
DC1, DC2DG5, DG6 | Phenanthrene | π –π | 23.47 | -10.41 | P14 | |
DC1, DC2DG5, DG6 | Phenanthrene | π –π | 11.61 | -10.83 | P15 | |
3.127 | DC1, DC2DG5, DG6, | S | H-bond, | 21.89 | -10.45 | P16 |
Interaction data of the most potent cytotoxic derivatives and the cocrystallized ligand (6-[(8E)-8-(1,3-benzothiazol-2-ylhydrazinylidene)-6,7-dihydro-5H-naphthalen-2-yl]pyridine-2-carboxylic acid) with Bcl-2 protein (PDB ID: 3ZLN).
Conclusion
Phenanthro-triazine-3-thiol derivatives have been synthesized and evaluated for their cytotoxic activity against MOLT-4 and MCF-7 cancer cell lines. Compounds P1, P11 and P15 were found to be the most potent cytotoxic agents. The docking study revealed that these derivatives unwounded the DNA double helix mainly via hydrophobic interactions with the GC base pairs. Additionally, the active compounds were well accumulated in the active site of Bcl-2 through interactions with Leu108, Ser106, Phe105, Ala142, Leu30, Ala149 and Phe146. Therefore, P1, P11, and P15 could be proposed as effective cytotoxic agents with potential DNA intercalation and Bcl-2 inhibitory activities. Further biological evaluations are necessary to confirm our findings.
Acknowledgements
References
-
1.
Espinosa E, Zamora P, Feliu J, Barón MG. Classification of anticancer drugs—a new system based on therapeutic targets. Cancer Treat. Rev. 2003;29:515-23. [PubMed ID: 14585261].
-
2.
Ranjbar S, Edraki N, Khoshneviszadeh M, Foroumadi A, Miri R, Khoshneviszadeh M. Design, synthesis, cytotoxicity evaluation and docking studies of 1, 2, 4-triazine derivatives bearing different arylidene-hydrazinyl moieties as potential mTOR inhibitors. Res. Pharm. Sci. 2018;13:1-11. [PubMed ID: 29387106].
-
3.
Hurley LH. DNA and its associated processes as targets for cancer therapy. Nat. Rev. Cancer. 2002;2:188-200. [PubMed ID: 11990855].
-
4.
Palchaudhuri R, Hergenrother PJ. DNA as a target for anticancer compounds: methods to determine the mode of binding and the mechanism of action. Curr. Opin. Biotechnol. 2007;18:497-503. [PubMed ID: 17988854].
-
5.
Wheate NJ, Brodie CR, Collins JG, Kemp S, Aldrich-Wright JR. DNA intercalators in cancer therapy: organic and inorganic drugs and their spectroscopic tools of analysis. Mini-Rev. Med. Chem. 2007;7:627-48. [PubMed ID: 17584161].
-
6.
Suryadi J, Bierbach U. DNA metalating–intercalating hybrid agents for the treatment of chemoresistant cancers. Chem. Eur. J. 2012;18:12926-34. [PubMed ID: 22987397].
-
7.
Rescifina A, Zagni C, Varrica MG, Pistarà V, Corsaro A. Recent advances in small organic molecules as DNA intercalating agents: Synthesis, activity, and modeling. Eur. J. Med. Chem. 2014;74:95-115. [PubMed ID: 24448420].
-
8.
Neto BA, Lapis AA. Recent developments in the chemistry of deoxyribonucleic acid (DNA) intercalators: principles, design, synthesis, applications and trends. Molecules. 2009;14:1725-46. [PubMed ID: 19471193].
-
9.
Salem OM, Vilková M, Janočková J, Jendželovský R, Fedoročko P, mrich J, Kožurková1 M. Synthesis, spectral characterization, DNA binding ability and anti-cancer screening of new acridine-based derivatives. Med. Chem. Res. 2017:1-13. [PubMed ID: 28111514].
-
10.
Ashkenazi A, Fairbrother WJ, Leverson JD, Souers AJ. From basic apoptosis discoveries to advanced selective BCL-2 family inhibitors. Nat. Rev. Drug Discov. 2017;16:273-84. [PubMed ID: 28209992].
-
11.
Radha G, Raghavan SC. BCL2: A Promising Cancer Therapeutic Target. Biochim. Biophys. Acta. Rev. Cancer. 2017;1:309-14.
-
12.
Um HD. Bcl-2 family proteins as regulators of cancer cell invasion and metastasis: a review focusing on mitochondrial respiration and reactive oxygen species. Oncotarget. 2016;7:5193-203. [PubMed ID: 26621844].
-
13.
Delbridge A, Grabow S, Strasser A, Vaux DL. Thirty years of BCL-2: translating cell death discoveries into novel cancer therapies. Nat. Rev. Cancer. 2016;16:99-109. [PubMed ID: 26822577].
-
14.
Yurttaş L, Demirayak Ş, Ilgın S, Atlı Ö. In vitro antitumor activity evaluation of some 1, 2, 4-triazine derivatives bearing piperazine amide moiety against breast cancer cells. Bioorg. Med. Chem. 2014;22:6313-23. [PubMed ID: 25438754].
-
15.
Karczmarzyk Z, Wysocki W, Urbańczyk-Lipkowska Z, Kalicki P, Bielawska A, Bielawski K, Ławecka J. Synthetic approaches for sulfur derivatives containing 1, 2, 4-Triazine moiety: their activity for in-vitro screening towards two human cancer cell lines. Chem. Pharm. Bull. 2015;63:531-7.
-
16.
Zhang Z, Yang H, Wu G, Li Z, Song T, qian Li X. Probing the difference between BH3 groove of Mcl-1 and Bcl-2 protein: Implications for dual inhibitors design. Eur. J. Med. Chem. 2011;46:3909-16. [PubMed ID: 21684048].
-
17.
Zhang Z, Jin L, Qian X, Wei M, Wang Y, Wang J, Yang Y, Xu Q, Xu Y, Liu F. Novel Bcl‐2 Inhibitors: Discovery and Mechanism Study of Small Organic Apoptosis‐Inducing Agents. Chem. Biol. Chem. 2007;8:113-21.
-
18.
Zhang Z, Yang Y, Zhang D, Wang Y, Qian X, Liu F. Acenaphtho [1, 2-b] pyrrole derivatives as new family of intercalators: Various DNA binding geometry and interesting antitumor capacity. Bioorg. Med. Chem. 2006;14:6962-70. [PubMed ID: 16828559].
-
19.
Song T, Li X, Chang X, Liang X, Zhao Y, Wu G, Xie S, Su P, Wu Z, Feng Y, Zhang Z. 3-Thiomorpholin-8-oxo-8H-acenaphtho [1, 2-b] pyrrole-9-carbonitrile (S1) derivatives as pan-Bcl-2-inhibitors of Bcl-2, Bcl-x L and Mcl-1. Bioorg. Med. Chem. 2013;21:11-20. [PubMed ID: 23206987].
-
20.
Mohammadi MK, Firuzi O, Khoshneviszadeh M, Razzaghi-Asl N, Sepehri S, Miri R. Novel 9-(alkylthio)-Acenaphtho [1, 2-e]-1, 2, 4-triazine derivatives: synthesis, cytotoxic activity and molecular docking studies on B-cell lymphoma 2 (Bcl-2). DARU J. Pharml. Sci. 2014;22:2.
-
21.
Wang S, Li H, Chen C, Zhang J, Li S, Qin X, Li X, Wang K. Cytotoxicity and DNA binding property of phenanthrene imidazole with polyglycol side chains. Bioorg. Med. Chem. lett. 2012;22:6347-51. [PubMed ID: 22989531].
-
22.
Sumantran VN, Ealovega MW, Nunez G, Clarke MF, Wicha MS. Overexpression of Bcl-XS sensitizes MCF-7 cells to chemotherapy-induced apoptosis. Cancer Res. 1995;55:2507-10. [PubMed ID: 7780958].
-
23.
Elia J, Petit K, Huvelin JM, Tannoury M, Diab-Assaf M, Carbonnelle D, Nazih H. Acetone Fraction of the Red Marine Alga Laurencia papillosa Reduces the Expression of Bcl-2 Anti-apoptotic Marker and Flotillin-2 Lipid Raft Marker in MCF-7 Breast Cancer Cells. Iran. J. Pharm. Res. 2020;19:321-30.
-
24.
Mandal M, Kumar R. Bcl-2 expression regulates sodium butyrate-induced apoptosis in human MCF-7 breast cancer cells. Cell Growth Differ. 1996;7:311-8. [PubMed ID: 8838861].