Abstract
Keywords
Introduction
Intracellular targeting has always been a challenge in drug delivery systems designing (1). Peptide-based delivery systems have been an efficient strategy that transport the desired macromolecules across the cell membrane with low cytotoxicity and high efficiency (2, 3). Considering their nano-scale size (7 to 30 amino acids in length), cationic or amphipathic peptides offer excellent potential to be employed as an efficient carriers for small chemotherapeutics to overcome multi-drug resistance (4-10). TAT and Antp are well-known cell-penetrating peptides (CPPs) derived from human immunodeficiency virus-1 (HIV-1) and the transcription factor of Drosophila melanogaster, respectively (11-13). Chimeric peptides, such as transportan 10 (TP10), are categorized as the second type of well-known peptides (14), and the third type includes the synthetic peptides family, of which polyarginine peptides have been mostly investigated (15, 16). Lately, synthetic peptides with tailorable sequences have gained much attention towards drug delivery systems, as they implement the natural CPPs functions. Amongst the investigated CPPs in experimental models, amphipathic peptides with hydrophobic and charged residues exhibit more potential as an appropriate drug carrier (17, 18). Moreover, amphipathic CPPs appear to be more effective in delivering small molecules due to their high biocompatibility and bioactivity in biological systems (12, 19, 20). However, there might be a few immune responses developed during the treatment that can neutralize the clinical effects of therapeutic peptides or be associated with destructive immune impacts (21, 22). As the immune system plays the primary protective role of the body against all foreign toxic and pathogenic particles, it can pose a significant challenge upon the entry of novel therapeutic carriers. Interactions between new therapeutics and subsets of the immune system induce cell signaling cascades, which may consequently induce the immune factors and immunomodulation (23). The immunogenicity of the CPPs can be affected by their different physicochemical properties such as size, surface charge, amino acids sequence, hydrophilicity, morphology, and the type of conjugated cargo (24). Based on recent reports, the modified CPPs have shown no immediate immune responses (25, 26). Moschos et al. has evaluated the p38 siRNA delivery using Penetratin and HIV-TAT to the murine lung cells. They could show that Penetratin complexed with siRNA induced an innate immune response, whereas siRNA complexed with HIV-TAT, or Penetratin alone, did not show any immunogenic behavior (27). Therefore, designing efficient drug delivery vectors to transduce the therapeutic agents through the cell membrane is the ultimate goal; however, their possible cytotoxicity and immunogenic response induction and the resulted undesired side effects should be considered as their severe limitations. In the present study, we design and fabricate several synthetic amphipathic peptides and their conjugations with a chemotherapy drug, Methotrexate (MTX), and evaluate their in-vitro concentration- and time-dependent cytotoxicity and undesired immunogenic effects on the Raji cell line.
Experimental
Materials and methods
Materials
Fmoc-Arg(Pbf)-OH, Fmoc-Trp(Boc)-OH, Fmoc-Glu(OtBu)-OH, Fmoc-Lys(Boc)-OH, Fmoc-Asn(Trt)-OH, and Fmoc-Gly-OH were obtained from AAPPTec (Louisville, KY). The coupling agents including O-(benzotriazole-1-yl)-N, N, N′, N′-tetramethyluronium tetrafluoroborate (TBTU), Hydroxy/benzotriazole (HoBt) and N, N-Diisopropylethylamine (DIPEA), scavengers (ethanedithiol, phenol, and triisopropylsilane (TIS)), and cleavage reagents (piperidine and trifluoroacetic acid (TFA)) were purchased from Sigma (St. Louis, MO). 3-(4,5-dimethylthiazol-2-yl)-2,5-diphenyltetrazolium bromide (MTT), RPMI 1640, fetal bovine serum (FBS), trypsin, ethylenediaminetetraacetic acid (EDTA), and penicillin/streptomycin were purchased from Invitrogen (Carlsbad, CA). Raji cell line was obtained from the Pasteur Institute, Tehran, Iran. All other organic reagents were of analytical grade and purchased from Sigma-Aldrich (St. Louis, MO, USA).
Peptide synthesis
Eight linear peptides, including W4K4, W4R4, [WK]4, [WR]4, WRNGRWR, WRNGR, NGRWR, and [R]6 were synthesized by solid-phase synthesis (SPS) method on 2-chlorotrityl chloride (CTC) resin in a stepwise manner (2). Fmoc-L-amino acids were coupled to the resin in the presence of coupling reagents using TBTU (2 equivalent), HoBt (2 equivalent), and DIPEA (2 equivalent) in N, N-dimethylformamide (DMF) after mixing for 2 h, followed by resin swelling. The Fmoc deprotection process was carried out by piperidine in DMF (20% v/v). After the sequence completion, the side chain deprotection and cleavage step were carried out using TFA/TIS/phenol/distilled water (88:2:5:5, v/v/v/v) for 2 h. In the end, the peptide-coupled resins were filtered, and the solvent was evaporated to obtain dry crude peptides.
Peptide-MTX conjugation
Regarding the carboxylic acid end groups of MTX molecules, the peptide-MTX conjugation process was similar to that explained for peptide synthesis (28). Briefly, the obtained resin-coupled CPPs were conjugated to MTX (3 equivalent to the amount of resin), using DIPEA (2.5 equivalent), HoBt (2.5 equivalent), and TBTU (2.5 equivalent) to form peptide-MTX conjugates after 48 h under stirring. Then, the reaction completion was confirmed by the Kaiser test. Finally, the peptide-MTX conjugates were cleaved from the resin using TFA/TIS/phenol/distilled water (88:2:5:5, v/v/v/v) 2 h, precipitated in cold diethyl ether, and washed three times. The supernatant solution was analyzed at 365 nm using an ultraviolet-visible (UV-Vis) spectrophotometer (Shimadzu, Japan) to calculate the MTX loading efficiency according to the equation below:
Synthesis of peptide-E8 nanoparticles
First, 2.5 mg of the selected peptide (NGRWR, [WK]4 or [WK]4-MTX) was dissolved in 50 µL dimethyl sulfoxide (DMSO). Then poly-glutamate (E8) was added in a 1:5 ratio (peptide: E8) under ultrasonication for 2 h to form a salt bridge between peptide and E8 and create the nanoparticles. The obtained solution was filtered through a 0.2 μm syringe filter and lyophilized.
Characterization
The size and morphology of NGRWR peptide and NGRWR-E8 nanoparticles (as representatives) were examined by scanning electron microscopy (SEM, Mira3 FEG-SEM Tescan 5.0 kV). Samples were prepared by drop-casting of a 5 mM aqueous solution (20 µL) onto the mica surface. Then, they were lyophilized, coated with gold, and analyzed with a high volume mode of SEM. Zetasizer Nano ZS (Malvern Instruments, Worcestershire, UK) was employed to examine the mean hydrodynamic diameter, size distribution, and zeta potential of NGRWR peptide and NGRWR-E8 nanoparticles. To this end, the samples were diluted in distilled water (pH 7.4), and the experiment was repeated three times per sample at 25 °C.
Cell culture and MTT assay
Human lymphoma cell line (Raji) was cultured in 75 cm2 cell culture flasks containing RPMI 1640 medium (Gibco), which was supplemented with 10% FBS, 2 mM L-glutamine, 100 units/mL penicillin, and 100 µg/mL streptomycin (29). The cell culture was carried out in a humidified atmosphere of 5% CO2, 95% air, and 37 °C. The cell viability of CPPs and peptide-MTX conjugates was determined by MTT assay in Raji cells. Hence, 4 × 104 Raji cells/well were seeded into a 96-well plate and cultured in the condition mentioned above for 24 h. Then, cells were treated with different CPPs including W4K4, W4R4, [WK]4, [WR]4, WRNGRWR, WRNGR, NGRWR, and [R]6 (5, 10, 25 and 50 mM) and peptide-MTX conjugates (25 and 50 nM of MTX) for 24, 48 and 72 h. The cell viability was determined by measuring the amount of MTT transformed to formazan salt using an ELISA reader at 570 nm (Bioteck Instruments, Winooski, VT, USA). The results were the means of three independent experiments performed in duplicate.
Immunosorbent assay
Cells were incubated with selected CPPs (5 µM), their conjugations with MTX (25 nM of MTX), [WK]4-E8 nanoparticles (5 µM), [WK]4-MTX-E8 nanoparticles (25 nM of MTX), and free MTX (25 nM) for 48 h. As a positive control, cells were incubated with 10 μg/mL of lipopolysaccharide (LPS) to induce IL-6 and TNF-α release. Then, the supernatant was collected and IL-6 and TNF-α secretion was evaluated using standard ELISA kits.
Statistical analysis
Statistical analysis was performed by GraphPad Prism v6.07 (Graph Pad Software, CA, USA). In the cytotoxicity and immunogenicity studies, one-way ANOVA was employed, followed by a multi-comparison test, where p < 0.05 was quoted as significance.
Results
Synthesis and characterization
The CPPs were synthesized by the SPS method on CTC resin in a stepwise manner. Table 1 summarizes the properties of obtained CPPs as well as E8. W4R4-MTX possessed the highest loading efficiency (97%) and drug to peptide percentage (24.02%). The lowest loading efficiency (36%) and drug to peptide percentage (8.76%) was seen for NGRWK-MTX conjugates. SEM was applied to evaluate the size and morphology of NGRWR peptide and NGRWR-E8 nanoparticles. Figure 1 indicates the spherical morphology of NGRWR peptide with a size of ~100 nm. On the other hand, the salt bridge between peptide sequence and E8 resulted in the rod-like structure of NGRWR-E8 nanoparticles with not much change in particle size compared to the free peptide. Based on results obtained from Zetasizer (Figure 2), the size distribution of NGRWR peptide was measured to be 26 nm (43%), 37 nm (20%), 50 nm (16%), and 50-250 nm (21%) with a zeta potential of +11 mv.
Furthermore, the size distribution of NGRWR-E8 nanoparticles was in the range of 28-280 nm, where 45% of particles were below 50 nm with a zeta potential of +6 mV. The integration of E8 in nanoparticles is the main reason for their lower surface charge compared to peptide alone. The relatively high surface charge in both samples and the resulted electrostatic repulsion justify their good solution stability.
Cell viability assay
MTT assay was employed to evaluate the cytotoxic behavior of different CPPs and peptide-MTX conjugates in Raji cells. Based on results, cells treated with W4K4, W4R4, [WK]4, WRNGRWR, WRNGR, NGRWR, and R6 peptides exhibited no significant cytotoxicity (p 0.05) at concentrations of 5, 10, 25, and 50 μM after 24, 48, and 72 h (Figure 3). However, 5 μM of [WR]4 reduced the cell viability down to 40% after 24 h (Figure 3a). Moreover, cells were treated with peptide-MTX conjugates to establish the cytotoxic profile of peptide-drug conjugations for future experiments.
It was shown that 48 h after treatments with drug complexes of W4R4, W4K4, [WK]4, WRNGRWR, WRNGR, and NGRWR, there was no significant cytotoxic effect at a concentration of 25 nM MTX (Figure 4). As there was a time-dependent change in cell viability of [WR]4-MTX, NGRWK-MTX and R6-MTX, thus we removed them from the following experiments. Therefore, W4R4, W4K4, [WK]4, WRNGRWR, WRNGR, and NGRWR peptides and their conjugations with MTX were the only candidates for the following in-vitro immunogenic experiments.
In-vitro immunogenicity assay
ELISA test was applied to investigate the immunogenicity of CPPs and peptide-MTX conjugates in the Raji cell line. We observed no significant effect on IL-6 (Figure 5a) or TNF-α (Figure 5b) release profile of cells treated with CPPs either alone or in conjugation with MTX (P > 0.05). A modest increase in cytokine activity triggered by W4R4-MTX and WRNGRWR-MTX was not considered statistically significant. Indicated influence on the release of IL-6 and TNF-α was insignificant compared to LPS (as a positive control for Raji cells) after 48 h of incubation.
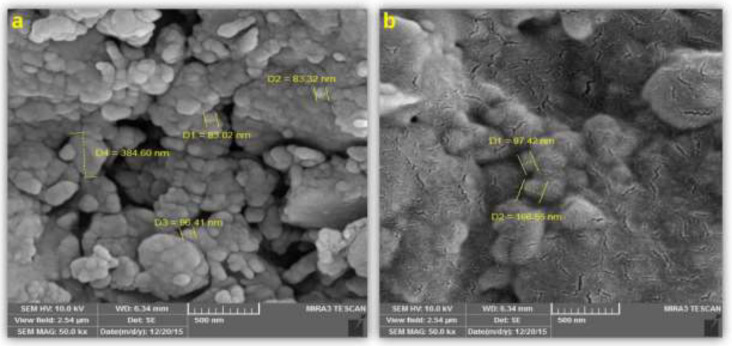
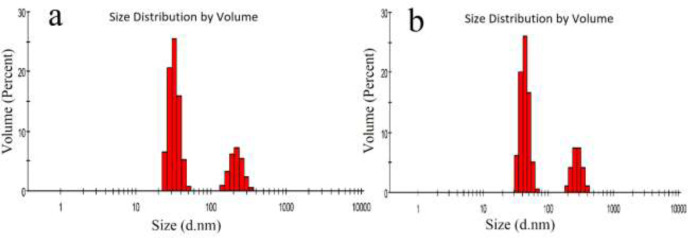
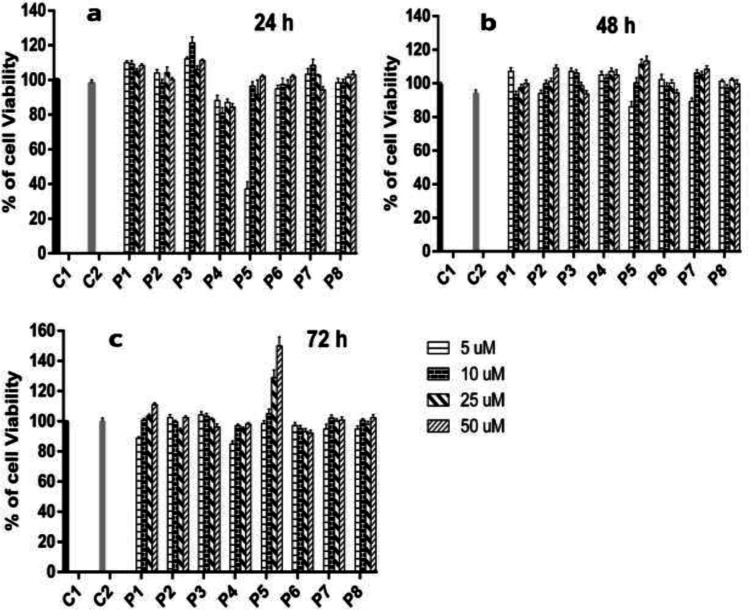
![Cell viability rates of W4R4-MTX (4a), [WK]4-MTX (4b), WRNGRWR-MTX (4c) and NGRWR-MTX (4d) after 24, 48 and 72 hr at concentrations of 25 and 50 nM of MTX. *P < 0.05, **P < 0.01, ***P < 0.001, ****P < 0.0001](https://services.brieflands.com/cdn/serve//315db/c404ac36d007c7c09239f1e483c9b71aa0ac9073/ijpr-20-506-g004.jpg)
![Cytokine release amount (pg/mL) in Raji cells was measured by ELISA assay. IL-6 (a) and TNF-α (b) release in cell culture supernatants were measured after 48 h of incubation with four peptides, their conjugations with MTX, [WK]4-E8 nanoparticles, [WK]4-MTX-E8 nanoparticles, and MTX. LPS (10 μg/mL) was used as a positive control, and untreated well was used as the negative control. The values represent the mean of at least three independent experiments performed in duplicate (mean ± SEM) decrease in viability was considered significant at P ≤ 0.05](https://services.brieflands.com/cdn/serve//315db/6a157b8545696fd9393b326570253d35eb7a8dd3/ijpr-20-506-g005.jpg)
The peptide sequences and properties
CPPs | Amino acid sequences | Charge at pH 7.3 | Molecular weight (g/mole) | MTX loading to CPPs |
---|---|---|---|---|
W4K4 | WWWWKKKK | +4 | 1275.54 | %93 |
W4R4 | WWWWRRRR | +4 | 1378.62 | %97 |
[WK]4 | WKWKWKWK | +4 | 1275.54 | %87 |
[WR]4 | WRWRWRWR | +4 | 1378.62 | %88 |
WRNGRWR | WRWNGRWRW | +3 | 1416.61 | %95 |
WRNGR | WRWRWRNGR | +4 | 1386.59 | %72 |
NGRWR | NGRWRWRWR | +4 | 1386.59 | %73 |
R6 | RRRRRR | +6 | 955.19 | %42 |
E8 | EEEEEEEE | -8 | 1050.94 | - |
Discussion
Nanocarriers are rapidly growing towards modifying several restrictions of conventional drug delivery systems for cancer therapy (12, 16). Peptide transporters are among the most well-known nano-drug delivery systems, which firstly was described in the 1988s and later became known as CPPs (1). CPPs offer exciting potential for effective delivery of biological molecules to the cytoplasm, which is otherwise impermeable to the cell membrane (30). However, their unwanted cytotoxic effects can limit clinical exposure to these nanocarriers. This study was performed to evaluate the cytotoxic and immunogenic behavior of several synthetic CPPs on the CPP-mediated transfer of MTX in-vitro.
Even though CPPs are presented as short sequences with no significant effect on the biological system, numerous studies have been done examining the biological impacts of various CPPs, including cationic and amphipathic ones (21). Amongst which, amphipathic sequences have demonstrated more toxicity on cell metabolism compared to cationic ones. For instance, as an amphipathic peptide, TP10 was found to affect cellular metabolism more than cationic Penetratin, and TAT (31). However, it has been shown that TP10 and its chemically modified derivatives induced no significant cytotoxicity and immunotoxicity at the concentrations of 10 μM and 5 μM in-vitro and 5 mg/kg of animal in-vivo (32). In previous reports, several amphipathic peptides were successfully applied to traverse the cell membrane with no significant cytotoxicity (1, 33). There have been other reports on the cytotoxic impacts of amphipathic peptides that proposed novel synthetic peptides as novel drug carriers for chemotherapeutics with the least cytotoxicity in-vitro. According to another study, there was a time- and dose-dependent toxicity with synthetic amphipathic peptides comprising tryptophan and arginine residues (34). Another group examined the in-vitro immunotoxicity of HIV-TAT, antennapedia, and transportan in A549 (alveolar), A431 (epidermal), and Caco-2 (intestinal) epithelial cells after 72 h. Consequently, there was no significant enhancement detected in inducing inflammatory cytokines in epithelial cells, indicating that these commonly used CPPs are passive carriers (34). Similarly, a cationic polyarginine peptide and penetration, at the dose of 1000 equivalent to their IC50, exhibited no toxic effect. However, it has been reported that TAT peptide could affect cell metabolism and cause some mild swelling in the rabbit’s cornea after 7 days (14, 31 and 32). Although the recent cytotoxic and immunogenic evaluations of different peptide sequences have been indicated no significant cell metabolism induction and biological responses, comprehensive investigations yet to be carried out. On the other hand, several advanced technologies such as nano-vectors (polymeric and liposomal), electroporation, genomics, and proteomics have been applied to overcome the challenges associated with cancer treatments (34, 35). Furthermore, the application of CPPs to achieve an efficient delivery system is critical in the field of chemotherapy. MTX is a folate antagonist that has been used for the treatment of various malignancies (36). The dose-dependent side effects of standard chemotherapeutics like MTX made them unsuitable for long-term therapies. It is essential to explore the potential applications of CPPs to deliver the therapeutic dosage of drugs to the cytoplasm of desired cells. The present study has addressed a significant safety concern regarding the application of CPPs for the efficient delivery of these drugs. In the present study, we evaluated the possible cytotoxic and immunogenic behavior of a new range of novel peptide sequences and their drug conjugates to elicit their possible role in the triggering of immune subsets or biological responses. Putting all together, we did not observe any significant cell viability reduction in Raji cells treated with each type of CPPs, as well as no activation of cytokine release after 48 h incubation with selected CPPs and peptide-MTX conjugates. Up to 50 μM, peptides illustrated no cytotoxic effect on Raji cells, while there was an exception for [WR]4 at 5µM after 24 h (Figure 3). Moreover, the conjugation of NGRWK, R6, and [WR]4 with MTX presented intensive cytotoxicity by reducing the cell viability down to 40%. Therefore, we removed cytotoxic peptide-MTX conjugates in the further immunogenic assessment. None of the measured cytokines (IL-6 or TNF-α) were overexpressed in the Raji cells after incubation with selected CPPs, peptide-MTX conjugates, or nanoparticles. Meanwhile, as expected, cells incubated with LPS as positive control illustrated cytokine level enhancement 100-times higher than the control group (Figure 5).
Conclusion
In summary, we have demonstrated that the W4R4, W4K4, [WK]4, WRNGRWR, WRNGR, and NGRWR peptides and their conjugations with MTX have no cytotoxic and immunogenic effects at estimated concentrations. The obtained evidence supports the potential of designed CPPs as promising carriers and holds a significant agreement for their safe applications. However, long-term immunogenicity and in-vivo cytotoxicity investigations must be carried out to confirm the data.
Acknowledgements
References
-
1.
Farkhani SM, Johari-ahar M, Zakeri-Milani P, Shahbazi-Mojarrad J, Valizadeh H. Enhanced cellular internalization of CdTe quantum dots mediated by arginine-and tryptophan-rich cell-penetrating peptides as efficient carriers. Artif. Cells Nanomed. Biotechnol. 2015;44:1424-28. [PubMed ID: 25884240].
-
2.
Mohammadi S, Zakeri-Milani P, Golkar N, Farkhani SM, Shirani A, Shahbazi-Mojarrad J, Nokhodchi A, Valizadeh H. Synthesis and cellular characterization of various nano-assemblies of cell penetrating peptide-epirubicin-polyglutamate conjugates for the enhancement of antitumor activity. Artif. Cells Nanomed. Biotechnol. 2018;46:1572-85. [PubMed ID: 28933182].
-
3.
Esfandiari Mazandaran K, Mirshokraee SA, Didehban K, Houshdar Tehrani MH. Design, synthesis and biological evaluation of Ciprofloxacin- peptide conjugates as anticancer agents. Iran. J. Pharm. Sci. 2019;18:1823-30.
-
4.
Derossi D, Joliot AH, Chassaing G, Prochiantz A. The third helix of the Antennapedia homeodomain translocates through biological membranes. J. Biol. Chem. 1994;269:10444-50. [PubMed ID: 8144628].
-
5.
Mäe M, Langel Ü. Cell-penetrating peptides as vectors for peptide, protein and oligonucleotide delivery. Curr. Opin. Pharmacol. 2006;6:509-14. [PubMed ID: 16860608].
-
6.
Vives E, Brodin P, Lebleu B. A truncated HIV-1 Tat protein basic domain rapidly translocates through the plasma membrane and accumulates in the cell nucleus. J. Biol. Chem. 1997;272:16010-17. [PubMed ID: 9188504].
-
7.
Pooga M, Hällbrink M, Zorko M. Cell penetration by transportan. FASEB J. 1998;12:67-77. [PubMed ID: 9438412].
-
8.
Rothbard JB, Garlington S, Lin Q, Kirschberg T, Kreider E, McGrane PL, Wender PL, Khavari PL. Conjugation of arginine oligomers to cyclosporin A facilitates topical delivery and inhibition of inflammation. Nat. Med. 2000;6:1253-57. [PubMed ID: 11062537].
-
9.
El-Andaloussi S, Holm T, Langel U. Cell-penetrating peptides: mechanisms and applications. Curr. Pharm. Des. 2005;11:3597-3611. [PubMed ID: 16305497].
-
10.
Baharloui M, Mirshokraee SA, Monfared A, Houshdar Tehrani MH. Design and Synthesis of Novel Triazole-based Peptide Analogues as Anticancer Agents. Iran. J. Pharm. Sci. 2019;18:1299-1308.
-
11.
Andaloussi SE, Lehto T, Ma ¨ger I, Rosenthal-Aizman K, Oprea I, Simonson OE, Sork H, Ezzat K, Copolovici DM, Kurrikoff K, Viola JR, Zaghloul E, Sillard R, Johansson H, Hassane FS, Guterstam P, Suhorutsenko J, Moreno P, Oskolkov N, Halldin J, Tedebark U, Metspalu T, Lebleu B, Lehtio J, Smith E, Langel U. Design of a peptide-based vector, PepFect6, for efficient delivery of siRNA in cell culture and systemically in vivo. Nucleic Acids Res. 2011;39:3972-87. [PubMed ID: 21245043].
-
12.
Suhorutsenko J, Oskolkov N, Arukuusk P, Kurrikoff K, Eriste E, Copolovici DM, Langel U. Cell-penetrating peptides, PepFects, show no evidence of toxicity and immunogenicity in-vitro and in vivo. Bioconjug. Chem. 2011;22:2255-62. [PubMed ID: 21978260].
-
13.
Nasrolahi Shirazi A, Oh D, Tiwari RK, Sullivan B, Gupta A, Boyhun GD, Parang K. Peptide amphiphile containing arginine and fatty acyl chains as molecular transporters. Mol. Pharm. 2013;10:4717-27. [PubMed ID: 24215132].
-
14.
Jeong JH, Kim SH, Kim SW, Park TG. Intracellular delivery of poly (ethylene glycol) conjugated antisense oligonucleotide using cationic lipids by formation of self-assembled polyelectrolyte complex micelles. J. Nanosci. Nanotechnol. 2006;6:2790-5. [PubMed ID: 17048484].
-
15.
Nasrolahi Shirazi A, Tiwari RK, Oh D, Banerjee A, Yadav A, Parang K. Efficient delivery of cell impermeable phosphopeptides by a cyclic peptide amphiphile containing tryptophan and arginine. Mol. Pharm. 2013;10:2008-20. [PubMed ID: 23537165].
-
16.
Mohammadi S, Shahbazi Mojarrad J, Zakeri-Milani P, Shirani A, Farkhani SA, Samadi N, Valizadeh H. Synthesis and In-vitro Evaluation of Amphiphilic Peptides and Their Nanostructured Conjugates. Adv. Pharm. Bull. 2015;5:41-9. [PubMed ID: 25789218].
-
17.
Baker M, Reynolds HM, Lumicisi B, Bryson CJ. Immunogenicity of protein therapeutics: the key causes, consequences and challenges. Self/nonself. 2010;1:314-22. [PubMed ID: 21487506].
-
18.
Balalaie S, Malakoutikhah M, Meritxell M, Fathi V, Giralt E, Haghighatnia Y, Hamdan F, Arabanian A. Efficient synthesis of norbuprenorphines coupled with enkephalins and investigation of their permeability. Iran. J. Pharm. Sci. 2019;18:1277-87.
-
19.
Najafi-Hajivar S, Zakeri-Milani P, Mohammadi H, Niazi M, Soleymani-Goloujeh M, Baradaran B, Valizadeh H. Overview on experimental models of interactions between nanoparticles and the immune system. Biomed. Pharmacother. 2016;83:1365-78. [PubMed ID: 27580456].
-
20.
Milani D, Athiyah U, Hariyadi DM, Pathak YV. Surface Modification of Nanocarriers for Specific Cell Targeting for Better Therapeutic Effect. Surface Modification of Nanoparticles for Targeted Drug Delivery: Springer, Switzerland. 2019:355-68.
-
21.
Carter E, Lau CY, Tosh D, Ward SG, Mrsny RJ. Cell penetrating peptides fail to induce an innate immune response in epithelial cells in-vitro: implications for continued therapeutic use. Eur. J. Pharm. Biopharm. 2013;85:12-19. [PubMed ID: 23958314].
-
22.
Moschos SA, Jones SW, Perry MM, Williams AE, Erjefalt JS, Turner JJ, Barnes PJ, Sproat BS, Gait MJ, Lindsay MA. Lung delivery studies using siRNA conjugated to TAT (48-60) and penetratin reveal peptide induced reduction in gene expression and induction of innate immunity. Bioconjug. Chem. 2007;18:1450-9. [PubMed ID: 17711319].
-
23.
Zhang G, Zeng X, Li P. Nanomaterials in cancer-therapy drug delivery system. J. Biomed. Nanotechnol. 2013;9:741-50. [PubMed ID: 23802404].
-
24.
Siahmansouri H, Somi MH, Babaloo Z, Baradaran B, Jadidi-Niaragh F, Atyabi F, Mohammadi H, Ahmadi M, Yousefi M. Effects of HMGA2 siRNA and doxorubicin dual delivery by chitosan nanoparticles on cytotoxicity and gene expression of HT‐29 colorectal cancer cell line. J. Pharm. Pharmacol. 2016;68:1119-30. [PubMed ID: 27350211].
-
25.
Snyder EL, Dowdy SF. Cell penetrating peptides in drug delivery. Pharm. res. 2004;21:389-93. [PubMed ID: 15070086].
-
26.
Verdurmen WP, Brock R. Biological responses towards cationic peptides and drug carriers. Trends Pharmacol. Sci. 2011;32:116-24. [PubMed ID: 21167610].
-
27.
Kilk K, Mahlapuu R, Soomets U, Langel U. Analysis of in-vitro toxicity of five cell-penetrating peptides by metabolic profiling. Toxicology. 2009;265:87-95. [PubMed ID: 19799958].
-
28.
Zakeri-Milani P, Farkhani A, Shirani A, Mohammadi S, Shahbazi Mojjarad J, Akbari J, Valizadeh H. Cellular uptake and anti-tumor activity of gemcitabine conjugated with new amphiphilic cell penetrating peptides. EXCLI J. 2017;16:650-62. [PubMed ID: 28694765].
-
29.
Farshbaf M, Salehi R, Annabi N, Khalilov R, Akbarzadeh A, Davaran S. pH- and thermo-sensitive MTX-loaded magnetic nanocomposites: synthesis, characterization, and in-vitro studies on A549 lung cancer cell and MR imaging. Drug Dev. Ind. Pharm. 2018;44:452-62. [PubMed ID: 29098882].
-
30.
Shirani A, Shahbazi Mojarrad J, Farkhani SM. The Relation Between Thermodynamic and Structural Properties and Cellular Uptake of Peptides Containing Tryptophan and Arginine. Adv. Pharm. Bull. 2015;5:161-8. [PubMed ID: 26236653].
-
31.
Akkarawongsa R, Cullinan AE, Zinkel A, Clarin J, Brandt CR. Corneal toxicity of cell-penetrating peptides that inhibit herpes simplex virus entry. J. Ocul. Pharmacol. 2006;22:279-89.
-
32.
Vivès E, Schmidt J, Pèlegrin A. Cell-penetrating and cell-targeting peptides in drug delivery. Biochim. Biophys. Acta Rev.Cancer. 2008;1786:126-38.
-
33.
Kim WJ, Christensen LV, Jo S, Yockman JW, Jeong JH, Kim YH, Kim SW. Cholesteryl oligoarginine delivering vascular endothelial growth factor siRNA effectively inhibits tumor growth in colon adenocarcinoma. Mol. Ther. 2006;14:343-50. [PubMed ID: 16765648].
-
34.
Hemmatzadeh M, Mohammadi H, Karimi M, Musavishenas MH, Baradaran B. Differential role of microRNAs in the pathogenesis and treatment of Esophageal cancer. Biomed. Pharmacother. 2016;82:509-19. [PubMed ID: 27470391].
-
35.
Rennert R, Neundorf I, Beck-Sickinger AG. Calcitonin-derived peptide carriers: mechanisms and application. Adv. Drug Deliv. Rev. 2008;60:485-98. [PubMed ID: 18160173].
-
36.
Genestier L, Paillot R, Quemeneur L, Izeradjene K, Revillard JP. Mechanisms of action of methotrexate. Immunopharmacol. 2000;47:247-57.