Abstract
Keywords
Biofilm Combination therapy Carbapenem-resistant Pseudomonas aeruginosa synergic
Introduction
Pseudomonas aeruginosa is the most frequent cause of healthcare-associated infections in vulnerable patients, particularly in burns cases. P. aeruginosa is the most common bacteria isolated from burn infection (1, 2). The frequency of mortality among infected burn patients is three times higher than non-infected burn patients (3). Control of P. aeruginosa infections is challenging due to inherently or acquired resistance to different antibiotics and virulence features such as biofilm formation (4, 5). Carbapenems are the most important therapeutic option in the treatment of severe Gram-negative infections. However, the increasing resistance among clinical P. aeruginosa isolates has become a major limitation for carbapenem usage (6). The mortality rate of carbapenem-resistant P. aeruginosa infection is high, and these isolates are more likely to have cross-resistance to other common antimicrobial agents. P. aeruginosa has multifactorial mechanisms of resistance to carbapenem including the production of carbapenemase, reduced or mutation of the outer membrane porin (OprD), decreased levels of the drug accumulation due to the overexpression of efflux-pumps over and the overexpression of AmpC beta-lactamases (7). Biofilms are surface-attached layers of bacteria in an extracellular polymeric compound that may form on human tissues as well as on a diversity of surfaces, such as prosthetic devices, venous catheters, and cardiac pacemakers. Planktonic forms of bacteria are free-living bacteria characterized by un-adhered to surfaces and to each other. Bacteria in a biofilm are much more resistant to antibiotics than to planktonic status. Biofilm formation is one of the most important virulence factors in P. aeruginosa (8). Biofilm elimination usually needs higher doses and continued drug therapy. However, this often does not successfully eliminate biofilm-caused infections (9). Until now, a new drug has not been introduced to control antibiotic-resistant and biofilm-forming P. aeruginosa isolates. It seems unlikely that any new option will be established soon, clinicians may become obliged to administrate old drugs, such as colistin, regardless of their side effects. Therefore, there is a reappearance of attention in the use of combination antimicrobial therapy (10). Combination therapy is one of the most effective strategies for managing the biofilm-forming and antibiotic-resistant P. aeruginosa isolates (11, 12). The high frequency of biofilm-forming and resistance of P. aeruginosa isolates to wide spectrum antimicrobial agents in burn patients demonstrate that written control programs require treating infection in patients (2, 8 and 10). Combination therapy usually is administrated due to synergic effects to improve the clinical outcome of infections caused by strains that are commonly susceptible to one of the individual antibiotics. Combination therapy also decreases toxicity and might prevent the emergence of antibiotics resistant isolates. The resistance to antibiotics influences the selection of such drugs and their potential for reaching to synergistic effects in combination (8). The combination therapy for P. aeruginosa usually includes a β-lactam plus other class of antibiotics. The increasing frequency of carbapenem-resistant may decrease the efficiency of carbapenem in monotherapy and combination therapy against P. aeruginosa. Synergy testing provides the evidence of the interaction of two or more antimicrobial agents in combination against bacterial isolates (9, 11). For example, although the fosfomycin is not recommended for the susceptibility testing of P. aeruginosa by the Clinical and Laboratory Standards Institute (CLSI), the results of several studies demonstrated that fosfomycin can increase the antimicrobial effects of the antimicrobial agents (13). Because of the impact geographical characteristics on the susceptibility pattern of carbapenem-resistant and biofilm-producing P. aeruginosa, the results of such study can be applied in the treatment of P. aeruginosa isolates from Tabriz, Iran. The aim of the present study was to evaluate in-vitro synergy between some combinations of antibiotics, including imipenem-based combination and others (fosfomycin, colistin, and gentamicin) combinations against planktonic as well as biofilm forms of carbapenem-resistant P. aeruginosa isolates (with different mechanisms of carbapenem-resistance) from burn patients.
Experimental
Bacterial isolates
The present study was performed on the carbapenem-resistant and biofilm-forming isolates of P. aeruginosa. In this study, forty non-duplicated P. aeruginosa isolates were collected from the burn patients and identified by the standard microbiological tests at the Microbiology Department of Tabriz University of Medical Sciences during 2017-2018. The inclusion criteria were carbapenem-resistant and biofilm-forming P. aeruginosa. Of 40 isolates, eight carbapenem-resistant, as well as biofilm-forming P. aeruginosa was selected. The sample size was determined based on an expected frequency of resistance of 7% (a priori estimate of frequency according to a pilot study result), an accepted error of 4% (required precision of the estimate), and 95% level of confidence, giving a required sample size of 40 samples.
Mechanisms of resistance to carbapenem
To screen carbapenem-resistant P. aeruginosa, the disk diffusion method was used. The AmpC mediated resistance was detected by the agar plate supplemented with cloxacillin (250µg/mL AmpC β-lactamase inhibitor). At least a twofold decrease in ceftazidime Minimum Inhibitory Concentration (MIC) in the presence of cloxacillin was considered as the AmpC mediated resistance (7). The Phenylalanine-Arginine Beta-Naphthylamide (PaβN) was used as an efflux pump inhibitor at a concentration of 40 μg/mL. The MICs of imipenem and meropenem were determined in the presence of PaβN. At least two-fold reduced MIC in the presence of PAβN considered as efflux pumps mediated resistance (14). Multiplex PCR was performed for the detection of carbapenemase encoding genes. The presence of blaIMP, blaVIM, blaSPM, blaNDM, blaKPCblaOXA-48, blaBIC, blaAIM, blaGIM, blaSIM, and blaDIM was evaluated by the PCR according to a previous study (15). The expression of mexB and oprD was detected by the real-time quantitative reverse transcription PCR (RT-PCR) and specific primers for rpsL, oprD, and mexB genes as recommended previously (7). The transcription level of rpsL housekeeping gene was used as standardized expression levels. RNA extraction from bacterial isolates was performed by an RNA extraction kit (SinaClon Co., Tehran, Iran) according to the manufacture guideline. The reactions were carried out using the Rotor-Gene Real-time PCR device (Corbett Research, Sydney, Australia; Model RG 3000) in duplicate runs by the SYBR premix EX TaqII, Tli RNaseH plus (Takara Bio Inc.). Gene’s expression was considered as ratios of the target gene and the housekeeping gene (rpsL) according to a relative quantification assay as described previously (7, 14).
Quantitative detection of biofilm
The microtiter plate test (MPT) was performed for the quantitative assessment of biofilm formation. Three colonies of the tested organism were suspended in 5 mL of Tryptic Soy Broth (TSB) and incubated for 20 h at 37 °C. After incubation, the culture was vortexed and then diluted 1:100 in TSB supplemented by 0.25% glucose. A 200 µL of this bacterial solution was inoculated in 96 well microplates and incubated for 20 h at 37 °C. The content of the wells was then removed. The wells were carefully washed three times with distilled water and air-dried. The staining was carried by 200 µL of 0.9% crystal violet solution for 15 min. Thereafter, crystal violet was removed, and wells washed with water. The attached crystal violet was solubilized by 95% ethanol and the optical density (OD) of the adherent biofilm was determined twice by the microtiter plate reader at wavelength 450-630 nm. TSB with 0.25% glucose was considered as a negative control (nc). All tested isolates according to a degree of biofilm formation were classified into three groups based on OD value: OD ≤ OD (nc) = non-biofilm producer (-), ODc ≤ 2OD (nc) = weak biofilm producer, 2OD (nc) < OD ≤ 4OD (nc) = moderate biofilm producer, 4OD (nc) < OD = strong biofilm producer (15).
Antibiotic susceptibility testing
The antibiotic susceptibility patterns were determined for eight screened carbapenem-resistant and biofilm-forming P. aeruginosa. The disk diffusion method was performed according to the CLSI guidelines (17). Antibiotic disks used in this study included ceftazidime, colistin, levofloxacin, cefepime, piperacilin/ tazobactam, aztreonam, ciprofloxacin, and gentamicin. MDR was considered as acquired non-susceptibility to at least one agent in three or more antimicrobial classes (18). The MICs of colistin, imipenem, gentamicin, and fosfomycin were determined by the broth microdilution according to the CLSI guidelines. Pseudomonas aeruginosa ATCC 27853 was used as the positive control of antimicrobial susceptibility testing (19).
BIC determination
The biofilm inhibitory concentration (BIC) was determined to study the antibiofilm effect of tested antibiotics alone and in the combination. About 100 µg of bacterial suspension equal to 0.5 McFarland in the nutrient broth was transferred to each well of a flat-bottomed 96-well microtiter plate. Biofilm formation was prompted by dipping the pegs of a modified polystyrene microtiter lid into this biofilm growth plate and incubating at 37 °C for 20 h. Peg lids were washed three times in sterile water, placed onto flat-bottomed microtiter plates containing serial concentrations of imipenem, colistin, gentamicin, and fosfomycin only and also in combination with each other in CAMHB per well, and incubated for 20 h at 37 °C. After the incubation time, the peg lid was washed in sterile water and placed into antibiotic-free CAMHB in a flat-bottomed microtiter plate. To transfer biofilms from the pegs to wells, each plate was centrifuged at 805 ×g for 20 min. The peg lid was changed by a usual cover. The OD at 650 nm was determined on a microtiter plate before and after incubation at 37 °C for 6 h. The BIC was defined as the lowest concentration of an antimicrobial agent that lid in an OD 650 variation at or below 10% of the mean of two positive control well readings (21).
FIC determination
The antibacterial effects of antimicrobial agent combinations were detected using the checkerboard assay and determination of FICI (Fractional Inhibitory Concentration Index). For the checkerboard assay, each drug’s MIC was determined alone and in combinations against each isolate in one 96-well microplate. A well without antimicrobial agent was considered as control of bacterial growth. The concentration ranges of each antibiotic in combination ranged from 1 to 32 times the MIC. Dilutions of drugs A and B were prepared with a twofold dilution. The FICI was determined as follows:
FICI =
The FICIs were interpreted as follows: synergy, FICI of ≤0.5, additively FICI of > 0.5 to ≥ 1; no interaction (indifference), FICI of >1 to ≤ 4; antagonism, FICI of >4 (22).
Statistical analysis
The data were analyzed using the Statistical Package for the Social Sciences (SPSS) software version 20. A comparison of the data among various groups was performed by Non-parametric tests. P-values ≤ 0 .05 were considered as a statistical significance.
Results
We evaluated 40 clinical isolates of P. aeruginosa collected from burn patients. Among 40 P. aeruginosa isolates, 21 (53%) and 25 (63%) were identified as carbapenem-resistant and biofilm-forming isolates, respectively. Among carbapenem-resistant isolates, 13 (62%) were biofilm-forming bacteria. MIC50 and MIC 90 of imipenem were 32 and 32 µg/mL, respectively. Among the biofilm-forming isolates, a strong, moderate, and weak degree of biofilm-forming ability were observed in 15, 6, 4 isolates, respectively. Multifactorial resistance mechanisms were observed in a higher frequency than carbapenem-resistant caused by one resistance mechanism (61.9% versus 38.1%). The co-presence of OprD decreased expression and mexB overexpression were observed as the most common (28.57%) mechanism of carbapenem resistance due to a multifactorial resistance mechanism. AmpC overproduction was detected in 7 (33.3%) isolates. In all AmpC overproduction isolates, they had the other resistance mechanisms such as OprD mediated resistance (1 isolate, 4.7%), mexB overexpression (4 isolates, 19.1%) and, both mexB and OprD mediated resistance (2 isolates, 9.5%). Multifactorial resistance mechanisms were included OprD decreased expression (14.28%), the efflux pump overexpression (14.28%) and carbapenemase (9.5%). Table 1 shows the various mechanisms of carbapenem resistance among our isolates. Eight carbapenem-resistant P. aeruginosa with different resistance mechanisms and biofilm-forming isolates were selected for the study. Six isolates have a mono-factorial mechanism of resistance to carbapenem and two isolates were resistant due to a multifactorial mechanism. Interestingly, all eight carbapenem-resistant P. aeruginosa were MDR. The characteristics and antibiotics resistance profile of the selected isolates are shown in Table 2. The MICs of colistin, imipenem, gentamicin, and fosfomycin are shown in Figure 1. The highest antimicrobial synergic effects (FICI ≥ 0.5) have been detected for the combination of colistin/fosfomycin and gentamicin/fosfomycin (5 of 8 isolates). Whiles lowest synergic effect against the planktonic form of bacteria has been observed in the case of gentamicin/imipenem and colistin/gentamicin (1 of 8 isolates). The antagonism effect was not observed in the present study. There was no significant statistical association between the synergistic effects of drug combinations against the planktonic form with the type of resistance mechanisms to carbapenems. Table 3 shows the antimicrobial effect of different antibiotics alone and in the combination against the planktonic form of bacteria. The antibiofilm effect of antibiotics was tested alone or in combination against biofilm-producing isolates. None of the antibiotics alone eradicate biofilm form at MIC or sub-MIC of each antibiotic agent. Figure 1 presents the MIC50 and BIC50 of antibiotics. In comparison, colistin/fosfomycin, imipenem/fosfomycin, colistin/imipenem, gentamicin/fosfomycin, and gentamicin/imipenem showed a synergic effect for 3, 2, 2, 2, and 1 isolates, respectively. There was no significant statistical association between the synergistic effect of antibiotics combination against bacterial biofilm and the ability of biofilm formation (strong, moderate, and weak types). The combination of gentamicin/colistin did not show any synergistic effect. Table 3 shows the BIC of antibiotics against the biofilm form of bacteria.
Discussion
The emergence and distribution of carbapenem-resistant strains may considerably compromise their usefulness (10). The results of our study showed a high prevalence of carbapenem-resistant (52.5%) P. aeruginosa isolates among burn patients. Another study from Iran also reported a high prevalence (94.7%) of carbapenem-resistant P. aeruginosa isolates from burn patients (23). The frequency of carbapenem-resistant P. aeruginosa is significantly different among various settings due to differences in infection control, antibiotic use, geographic area, and the various treatment procedures (16). Multifactorial carbapenem resistance mechanisms were detected in the higher frequency than carbapenem resistance caused by one mechanism. In the present study, the most frequent mechanism of resistance to carbapenems was the overexpression of efflux pumps detected phenotypically or genetically among 71.4% of isolates. The overexpression of efflux pumps has been reported as the most common mechanism of resistance to carbapenems among P. aeruginosa (7, 24).
Antimicrobial combination therapy is one of the most effective options for the control of resistance to antibiotic isolates. Synergy assessment has clarified the interaction of two drugs in combination against bacterial isolates. In the present study, the interactions of imipenem, colistin, fosfomycin, and gentamicin have been evaluated for the eradication of planktonic and biofilm forms of carbapenem-resistant P. aeruginosa isolates in-vitro condition. According to the microbroth dilution, 4 of 8 isolates were colistin susceptible (MIC ≤ 2 µg/mL) and 4 of 8 isolates were colistin intermediate (2 µg/mL < MIC < 8 µg/mL). The emerging of colistin-resistant P. aeruginosa isolates has been reported in other studies (8, 25). Colistin is commonly considered as the last resort for infections caused by carbapenem-resistant P. aeruginosa isolates. In clinical practice, the combination therapy is commonly used to increase its antibacterial effect, despite the consequent increase in toxicity (26). In the present study, the combination of colistin and fosfomycin showed the synergistic and additive effect against 5/8 and 2/8 isolates, respectively. The synergic effect of colistin and fosfomycin was observed among 3 colistin intermediate isolates. In-vitro activity of fosfomycin in combination with colistin against carbapenem-resistant Gram-negative bacteria has been evaluated by other studies. Similar to our study, Di et al. reported the combination of colistin with fosfomycin had a synergistic and partial synergistic effect in 49.43% of the isolates, and no antagonism was observed (27). Some studies have also reported that fosfomycin improves the treatment outcomes, prevents antimicrobial resistance, and decreases the toxicity induced by the different antibiotics (28). Souli et al. found 5 mg/L colistin combined with 100 mg/L fosfomycin resulted in a bactericidal effect against 65% of carbapenem-resistant Klebsiellapneumonia isolates (29). In combination, the fosfomycin and colistin MIC for most of the isolates were significantly lower than the plasma concentrations that can be achieved for both agents (30). The combination of fosfomycin with colistin has been studied in infections due to the foreign-body model and is suggested as a promising treatment option for implant-associated infections by Gram-negative bacilli (31). According to the results of the checkerboard method, fosfomycin plus gentamicin has a synergistic effect against 5 of 8 isolates. Okazaki et al. reported that fosfomycin in combination with gentamicin had an efficacy rate of 70% against MDR P. aeruginosa isolates (32). Fosfomycin may increase the cellular uptake of aminoglycosides, resulting in increased inhibition of protein synthesis and ultimately bacterial killing (13). The synergy effect of aminoglycosides plus fosfomycin not only showed in-vitro but also increased the therapeutic effect in a rat model. This combination offers an effective treatment strategy against some drug-resistant bacteria (21). In the present study, the combination of fosfomycin and colistin, fosfomycin and imipenem showed a significant synergy (50%) and additive effect (50%) against the planktonic forms of imipenem-resistant P. aeruginosa. The in-vitro effects of fosfomycin in combination with other antibiotics have been studied against clinical isolates of P. aeruginosa with different antibiotic resistance patterns in the different studies. According to the results of Okazaki et al., fosfomycin plus carbapenems had an appropriate efficacy rate (76.6% with meropenem and 73.3% with imipenem) against MDR P. aeruginosa (32). In contrast, Tessier reported that fosfomycin/imipenem had an additive and indifference effect on 37% and 63% of isolates, respectively (34). Samonis et al. reported the synergic effect for the combination of fosfomycin with imipenem and meropenem on 46.7%, 53.3% of isolates, respectively (35). It is speculated that fosfomycin may offer alternative permeability routes for antibiotics into the bacteria by destroying the outer membrane and increases the antimicrobial effects (32,33). Therefore, even if an MDR P. aeruginosa isolate is highly resistant to an antibiotic, it may be sensitive to the same antibiotic when administered in combination with fosfomycin. Antibiotic combinations must be carefully considered to minimize the selection of strains with double resistance. It has been shown that the probability emergence of mutants resistant to the combination of fosfomycin with imipenem is significantly high but is not detectible for combinations of fosfomycin with tobramycin, amikacin, meropenem, ciprofloxacin, and colistin (35).
In this study, the imipenem and colistin combination has a synergic or additive effect against 3 and 4 isolates, respectively. The synergistic or additive effect of colistin plus imipenem was previously reported against imipenem-resistant and colistin-resistant subpopulations of P. aeruginosa (38). The combinations of gentamicin/colistin and gentamicin/imipenem had synergic for 1/8 of isolates. The synergistic effect of imipenem in the combination with aminoglycosides has been reported for 10% of imipenem-resistant and 8% of MDR P. aeruginosa isolates (39, 40). Considering the increased prevalence of multidrug-resistant organisms, synergism testing becomes a potentially powerful tool to help in the selection of appropriate antibiotic therapy (41). P. aeruginosa, the form of biofilms, is significantly resistant to eradication by antibiotic therapy. Conventional antibiotic susceptibility testing surveys the efficiency of antibiotics against the planktonic form of organisms under aerobic conditions. Thus, the determination of an antibiotic’s BIC may help treat infections caused by biofilm-producing bacteria (8). None of the imipenem, colistin, gentamicin, and fosfomycin was inhibited biofilm formation alone at MIC or sub MIC of each antibiotic agent in the present study. A synergetic or additive effect was detected between colistin/fosfomycin, imipenem/fosfomycin, gentamicin/fosfomycin, gentamicin/imipenem, and imipenem/colistin. For colistin/gentamicin, only the additive effect was observed in 5 of 8 isolates. Other studies have shown the synergistic effect of the various antibiotics for biofilm eradication. However, due to the high level of different antibiotics BIC, even with the potential of synergistic interactions, the use of these antibiotics may be associated with the toxicity effect in the patients. While synergistic interaction between several combinations was observed in the present study, clinical observation to support these results may be conflicting. In some studies, a significant association between in-vitro synergy assessment (by the time-kill or checkerboard assay) and the clinical outcome was not found. Additionally, the results of the checkerboard synergy test may not be correlated with the other method. Therefore, the results of the present study are better to be confirmed by others in-vitro synergy testing and clinical studies.
The various mechanisms of resistance to carbapenem in 21 carbapenem-resistant P. aeruginosa isolated from the burn patients according to monofactorial resistance mechanisms or multifactorial resistance mechanisms
Mechanism of Resistance | Number (%) | |
---|---|---|
Monofactorial | Carbapenemase | 2 (9.5%) (one for blaIMP and one for blaVIM) |
OprD | 3 (14%) | |
Efflux Pump | 3 (14%) | |
Multifactorial | OprD/AmpC | 1 (4.7%) |
Efflux Pump/AmpC | 4 (19.5%) | |
OprD/Efflux Pump | 6 (28.5%) | |
OprD/Efflux Pump /AmpC | 2 (9.5) |
The different resistance mechanisms and antibiotics resistance profile in 8 carbapenem and biofilm-forming isolates
Isolates | Mechanism of carbapenem-resistant | Degree of biofilm formation | Resistant to antibiotics |
---|---|---|---|
1 | Carbapenemase | S | CAZ, FEP, LVX, AZT, CIP, GEN |
2 | Carbapenemase | S | CAZ, LVX, CIP, GEN |
3 | Efflux Pump | W | CAZ, FEP, LVX, PTZ, AZM, CIP, GEN |
4 | Efflux Pump | S | CAZ, FEP, AZM, GEN |
5 | OprD | W | FEP, LVX, , PTZ, AZM CIP |
6 | OprD | S | CAZ, FEP, LVX, PTZ, AZM, CIP, GEN |
7 | OprD/Efflux Pump | M | CAZ, FEP, LVX, PTZ, AZM, CIP |
8 | Efflux Pump/AmpC | M | FEP, LVX, PTZ, AZM, CIP |
The MICs and BICs of antibiotics alone and the combination against biofilm and planktonic form of tested bacteria
Isolates, | Inhibitory concentration of tested antibiotics | The combination effects of tested antibiotics | |||||||||||||||||||
---|---|---|---|---|---|---|---|---|---|---|---|---|---|---|---|---|---|---|---|---|---|
FOS | IMI | COL | GEN | COL/FOS | IMI/FOS | GEN/FOS | GEN/IMI | IMI/COL | COL/GEN | ||||||||||||
BIC | MIC | BIC | MIC | BIC | MIC | BIC | MIC | Bio | Pln | Bio | Pln | Bio | Pln | Bio | Pln | Bio | Pln | Bio | Pln | ||
1 | 512 | 128 | 512 | 32 | 64 | 2 | 1024 | 32 | A | S | S | S | NI | S | A | A | NI | A | NE | S | |
2 | 512 | 64 | 512 | 64 | 128 | 4 | 512 | 128 | NI | S | A | S | S | S | NI | A | NI | A | A | A | |
3 | 1024 | 32 | 256 | 16 | 128 | 2 | 512 | 256 | NI | NE | NI | A | NI | A | NI | A | A | S | NE | A | |
4 | 1024 | 64 | 256 | 16 | 64 | 1 | 1024 | 16 | A | A | NI | A | NI | A | NI | S | S | A | A | A | |
5 | 1024 | 256 | 512 | 64 | 128 | 4 | 512 | 4 | S | S | S | S | A | S | NI | A | NI | S | A | NI | |
6 | 1024 | 32 | 1024 | 128 | 128 | 4 | 512 | 32 | NI | S | NI | S | NI | S | NI | A | NI | NI | NI | A | |
7 | 512 | 16 | 256 | 32 | 128 | 2 | 512 | 4 | S | S | NI | A | NI | S | S | A | S | A | A | A | |
8 | 512 | 64 | 512 | 32 | 128 | 4 | 512 | 8 | S | A | NI | A | S | NI | NI | A | NI | S | A | A |
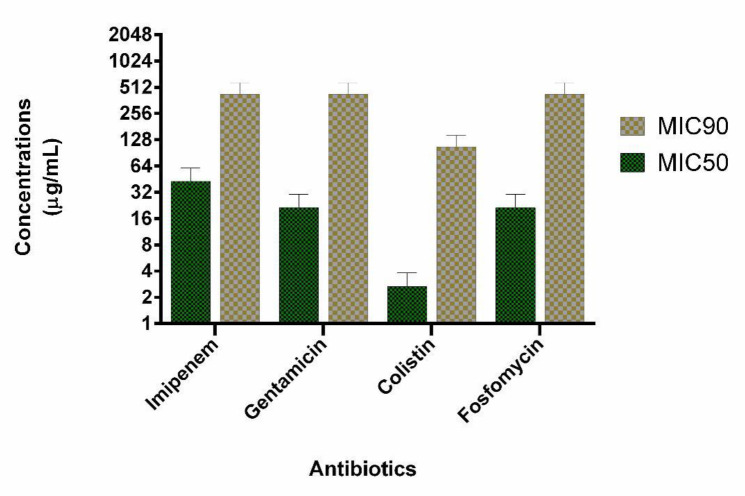
Conclusion
The results of the present study show that the combination of antimicrobial agents has different effects on biofilm and the planktonic forms. However, a separate determination of inhibitory effects of the antibiotic in the combination against the planktonic and biofilm may be applicable for guiding antibiotic therapy. The combination therapy using fosfomycin and colistin, gentamicin, or imipenem may be helpful in eradicating the planktonic form of carbapenem-resistant P. aeruginosa. The high concentrations of colistin/fosfomycin, imipenem/fosfomycin, gentamicin/fosfomycin, gentamicin/imipenem, and imipenem/colistin combinations in biofilm-forming bacteria show the synergistic effect.
Acknowledgements
References
-
1.
Tredget EE, Shankowsky HA, Rennie R, Burrell RE, Logsetty S. Pseudomonas infections in the thermally injured patient. Burns. 2004;30:3-26. [PubMed ID: 14693082].
-
2.
Gupta P, Chhibber S, Harjai K. Efficacy of purified lactonase and ciprofloxacin in preventing systemic spread of Pseudomonas aeruginosa in murine burn wound model. Burns. 2015;41:153-62. [PubMed ID: 25015706].
-
3.
Fournier A, Voirol P, Krähenbühl M, Bonnemain C-L, Fournier C, Pantet O, Pagani JL, Revelly JP, Dupuis-Lozeron E, Sadeghipour F. Antibiotic consumption to detect epidemics of Pseudomonas aeruginosa in a burn centre: A paradigm shift in the epidemiological surveillance of Pseudomonas aeruginosa nosocomial infections. Burns. 2016;42:564-70. [PubMed ID: 26708236].
-
4.
Joo EJ, Kang CI, Ha YE, Kang SJ, Park SY, Chung DR, Peck KR, Lee NY, Song JH. Risk factors for mortality in patients with Pseudomonasaeruginosa bacteremia: clinical impact of antimicrobial resistance on outcome. Microb. Drug. Resist. 2011;17:305-12. [PubMed ID: 21381966].
-
5.
Memar MY, Yekani M, Alizadeh N, Baghi HB. Hyperbaric oxygen therapy: Antimicrobial mechanisms and clinical application for infections. Biomed. Pharmacother. 2019;109:440-7. [PubMed ID: 30399579].
-
6.
Britt NS, Ritchie DJ, Kollef MH, Burnham CAD, Durkin MJ, Hampton NB, Micek ST. Importance of site of infection and antibiotic selection in the treatment of carbapenem-resistant Pseudomonas aeruginosa sepsis. Antimicrob.Agents Chemother. 2018;62:e02400-17. [PubMed ID: 29378722].
-
7.
Rodríguez-Martínez J-M, Poirel L, Nordmann P. Molecular epidemiology and mechanisms of carbapenem resistance in Pseudomonasaeruginosa. Antimicrob. Agents Chemother. 2009;53:4783-8. [PubMed ID: 19738025].
-
8.
Ghorbani H, Memar MY, Sefidan FY, Yekani M, Ghotaslou R. In-vitro synergy of antibiotic combinations against planktonic and biofilm Pseudomonasaeruginosa. GMS . Hyg. Infect. Control. 2017;12. Doc17.
-
9.
Wu H, Moser C, Wang HZ, Høiby N, Song ZJ. Strategies for combating bacterial biofilm infections. Int. J. Oral. Sci. 2015;7:1-7. [PubMed ID: 25504208].
-
10.
Memar MY, Pormehrali R, Alizadeh N, Ghotaslou R, Bannazadeh Baghi H. Colistin, an option for treatment of multiple drug resistant Pseudomonasaeruginosa. Physiol. Pharmacol. 2016;20:130-6.
-
11.
Vestergaard M, Paulander W, Marvig RL, Clasen J, Jochumsen N, Molin S, Jelsbak L, Ingmer H, Folkesson A. Antibiotic combination therapy can select for broad-spectrum multidrug resistance in Pseudomonas aeruginosa. Int. J.Antimicrob. Agents. 2016;47:48-55. [PubMed ID: 26597931].
-
12.
Nakamura I, Yamaguchi T, Tsukimori A, Sato A, Fukushima S, Matsumoto T. New options of antibiotic combination therapy for multidrug- resistant Pseudomonas aeruginosa. Eur. J. Clin. Microbiol. Infect. Dis. 2015;34:83-7. [PubMed ID: 25070493].
-
13.
MacLeod DL, Velayudhan J, Kenney TF, Therrien JH, Sutherland JL, Barker LM, Baker WR. Fosfomycin enhances the active transport of tobramycin in Pseudomonas aeruginosa. Antimicrob. Agents Chemother. 2012;56:1529-38. [PubMed ID: 22232284].
-
14.
Goli HR, Nahaei MR, Rezaee MA, Hasani A, Kafil HS, Aghazadeh M, Sheikhalizadeh V. Contribution of mexAB-oprM and mexXY (-oprA) efflux operons in antibiotic resistance of clinical Pseudomonas aeruginosa isolates in Tabriz, Iran. Infect. Genet. Evol. 2016;45:75-82. [PubMed ID: 27562333].
-
15.
Poirel L, Walsh TR, Cuvillier V, Nordmann P. Multiplex PCR for detection of acquired carbapenemase genes. Diagn. Microbiol. Infect. Dis. 2011;70:119-23. [PubMed ID: 21398074].
-
16.
Ghadaksaz A, Fooladi AAI, Hosseini HM, Amin M. The prevalence of some Pseudomonas virulence genes related to biofilm formation and alginate production among clinical isolates. J. Appl. Biomed. 2015;13:61-8.
-
17.
Patel J, Cockerill F, Alder J, Bradford P, Eliopoulos G, Hardy D, Hindler J, Jenkins S, Lewis J, Miller L. Performance standards for antimicrobial susceptibility testing; twenty-fourth informational supplement CLSI standards for antimicrobial susceptibility testing. 30th ed. Clinical and Laboratory Standards Institute, Pittsburgh. 2014;34:1-226.
-
18.
Magiorakos AP, Srinivasan A, Carey R, Carmeli Y, Falagas M, Giske C, Harbarth S, Hindler J, Kahlmeter G, Olsson-Liljequist B. Multidrug-resistant, extensively drug-resistant and pandrug-resistant bacteria: an international expert proposal for interim standard definitions for acquired resistance. Clin. Microbiol.Infect. 2012;18:268-81. [PubMed ID: 21793988].
-
19.
Wayne P. CLSI. Methods for dilution antimicrobial susceptibility tests for bacteria that grow aerobically; approved standard, 10th ed. CLSI document M07-A10. Clinical and Laboratory Standards Institute. USA. 2018:27-30.
-
20.
Wayne P. CLSI performance standard of antimicrobial susceptibility testing: twenty-fourth international supplement. CLSI Document M100-S24, Clinical and Laboratory Standard Institute. . USA; 2020. p. 42-45.
-
21.
Moskowitz SM, Foster JM, Emerson J, Burns JL. Clinically feasible biofilm susceptibility assay for isolates of Pseudomonas aeruginosa from patients with cystic fibrosis. J. Clin. Microbiol. 2004;42:1915-22. [PubMed ID: 15131149].
-
22.
Cai Y, Fan Y, Wang R, An MM, Liang BB. Synergistic effects of aminoglycosides and fosfomycin on Pseudomonas aeruginosa in-vitro and biofilm infections in a rat model. J. Antimicrob. Chemother. 2009;64:563-6. [PubMed ID: 19561148].
-
23.
Moazami-Goudarzi S, Eftekhar F. Assessment of carbapenem susceptibility and multidrug-resistance in Pseudomonas aeruginosa burn isolates in Tehran. Jundishapur J. Microbiol. 2013;6:162-5.
-
24.
Quale J, Bratu S, Gupta J, Landman D. Interplay of efflux system, ampC, and oprD expression in carbapenem resistance of Pseudomonasaeruginosa clinical isolates. Antimicrob. Agents. Chemother. 2006;50:1633-41. [PubMed ID: 16641429].
-
25.
Denton M, Kerr K, Mooney L, Keer V, Rajgopal A, Brownlee K, Arundel P, Conway S. Transmission of colistin-resistant Pseudomonas aeruginosa between patients attending a pediatric cystic fibrosis center. Pediatr. Pulmonol. 2002;34:257-61. [PubMed ID: 12205566].
-
26.
Petrosillo N, Ioannidou E, Falagas M. Colistin monotherapy vs combination therapy: evidence from microbiological, animal and clinical studies. Clin. Microbiol. Infect. 2008;14:816-27. [PubMed ID: 18844682].
-
27.
Di X, Wang R, Liu B, Zhang X, Ni W, Wang J, Liang B, Cai Y, Liu Y. In-vitro activity of fosfomycin in combination with colistin against clinical isolates of carbapenem-resistant Pseudomas aeruginosa. J. Antibiot. 2015;68:551-5.
-
28.
Nakamura T, Hashimoto Y, Kokuryo T, Inui KI. Effects of fosfomycin and imipenem/cilastatin on nephrotoxicity and renal excretion of vancomycin in rats. Pharm. Res. 1998;15:734-8. [PubMed ID: 9619782].
-
29.
Souli M, Galani I, Boukovalas S, Gourgoulis MG, Chryssouli Z, Kanellakopoulou K, Panagea T, Giamarellou H. In-vitro interactions of antimicrobial combinations with fosfomycin against KPC-2-producing Klebsiellapneumoniae and protection of resistance development. Antimicrob. Agents Chemother. 2011;55:2395-7. [PubMed ID: 21321144].
-
30.
Roussos N, Karageorgopoulos DE, Samonis G, Falagas ME. Clinical significance of the pharmacokinetic and pharmacodynamic characteristics of fosfomycin for the treatment of patients with systemic infections. Int. J.Antimicrob. Agents. 2009;34:506-15. [PubMed ID: 19828298].
-
31.
Corvec S, Tafin UF, Betrisey B, Borens O, Trampuz A. Activity of fosfomycin, tigecycline, colistin and gentamicin against extended spectrum beta-lactamase (ESBL)-producing E coli in a foreign-body infection model. Antimicrob. Agents. Chemother. 2013;57:1421-42. [PubMed ID: 23295934].
-
32.
Okazaki M, Suzuki K, Asano N, Araki K, Shukuya N, Egami T, Higurashi Y, Morita K, Uchimura H, Watanabe T. Effectiveness of fosfomycin combined with other antimicrobial agents against multidrug-resistant Pseudomonas aeruginosa isolates using the efficacy time index assay. J. Infect. Chemothe. 2002;8:37-42.
-
33.
MacLeod DL, Velayudhan J, Kenney TF, Therrien JH, Sutherland JL, Barker LM, Baker WR. Fosfomycin enhances the active transport of tobramycin in Pseudomonas aeruginosa. Antimicrob. Agents Chemother. 2012;56:1529-38. [PubMed ID: 22232284].
-
34.
Tessier F, Quentin C. In-vitro activity of fosfomycin combined with ceftazidime, imipenem, amikacin, and ciprofloxacin against Pseudomonas aeruginosa. Eur. J. Clin. Microbiol. Infect.Dis. 1997;16:159-62. [PubMed ID: 9105845].
-
35.
Samonis G, Maraki S, Karageorgopoulos D, Vouloumanou E, Falagas M. Synergy of fosfomycin with carbapenems, colistin, netilmicin, and tigecycline against multidrug-resistant Klebsiella pneumoniae, Escherichia coli, and Pseudomonas aeruginosa clinical isolates. Eur. J.Clin. Microbiol. Infect. 2012;31:695-701.
-
36.
Hayashi I, Sakurai M, Karato A, Ichiki M, Sekine I, Ishikawa T, Shiotani J, Yoshida T, Niida M, Ogawa M. Laboratory and clinical studies on combined effects of fosfomycin plus sulbactam/cefoperazone for mixed infections of MRSA and Pseudomonas aeruginosa. Jpn. J. Antibiot. 1994;47:991-1005. [PubMed ID: 7933542].
-
37.
Rodríguez-Rojas A, Couce A, Blázquez J. Frequency of spontaneous resistance to fosfomycin combined with different antibiotics in Pseudomonas aeruginosa. Antimicrob. Agents. Chemother. 2010;54:4948-9. [PubMed ID: 20713658].
-
38.
Bergen PJ, Forrest A, Bulitta JB, Tsuji BT, Sidjabat HE, Paterson DL, Li J, Nation RL. Clinically relevant plasma concentrations of colistin in combination with imipenem enhance pharmacodynamic activity against multidrug-resistant P aeruginosa at multiple inocula. Antimicrob. Agents Chemother. 2011;55:5134-42. [PubMed ID: 21876058].
-
39.
Bustamante C, Drusano G, Wharton R, Wade J. Synergism of the combinations of imipenem plus ciprofloxacin and imipenem plus amikacin against Pseudomonas aeruginosa and other bacterial pathogens. Antimicrob. Agents Chemother. 1987;31:632-4. [PubMed ID: 3111357].
-
40.
Dundar D, Otkun M. In-vitro efficacy of synergistic antibiotic combinations in multidrug resistant Pseudomonas aeruginosa strains. Yonsei. Med. J. 2010;51:111-6. [PubMed ID: 20046523].
-
41.
Cappelletty DM, Rybak MJ. Comparison of methodologies for synergism testing of drug combinations against resistant strains of Pseudomonas aeruginosa. Antimicrob. Agents Chemother. 1996;40:677-83. [PubMed ID: 8851592].