Abstract
Keywords
Brain-derived neurotrophic factor Pathogenesis Nanomaterials Hydrogel Drug delivery Neuropsychiatric diseases
Introduction
BDNF is a neurotrophic factor is a protein that supports the function of the central nervous system. BDNF is mainly distributed in the central nervous system, and is highly expressed in the hippocampus and frontal cortex (1, 2). In addition, it is also distributed in other areas, such as the peripheral nervous system, endocrine system, and bone and cartilage tissue (3-5). During the development of the nervous system, BDNF performs important functions by influencing cell differentiation, neuron development, growth and survival, neurogenesis, synapse formation and synaptic plasticity (5, 6). BDNF can bind to tyrosine kinase B (TrkB) to activate intracellular domains, promote TrkB autophosphorylation, and finally activate the three main pathways: Ras-MAPK, PI3K, and PLC-γ resulting in the activation of the cAMP response element-binding protein (CREB) (7-9) (Figure 1). CREB promotes the survival of nerve cells and improves synaptic plasticity and neurogenesis by increasing the expression of the BDNF gene and BCL-2 anti-apoptotic protein gene (10). NF-κB (nuclearfactor-kappa B) is one of the major factors of inflammatory activation, and it is a transcriptional factor, which can induce the expression of pro-apoptotic and anti-apoptotic genes and BDNF.In addition, mature BDNF (mature-BDNF, m-BDNF) and the BDNF precursor (pro-BDNF) can bind to the low-affinity p75 neurotrophin receptor (p75NTR) to produce corresponding biological activity (11). Recently, an increasing amount of research has been conducted on BDNF, and its genetic polymorphism has become a subject that is of great interest to researchers. The BDNF gene is located on chromosome 11p13-14. If the 66th codon, guanine, in the coding region of the gene is replaced by adenine, methionine replaced valine, resulting in BDNF Val66Met polymorphism, which is closely associated with cognitive dysfunction (12). Studies have shown that a lack of BDNF can lead to mental disorders and neurodegenerative diseases (13). At present, stem cells are used to produce BDNF and for the targeted delivery of BDNF to treat certain diseases, but the main challenge is that drugs or stem cells cannot cross the blood-brain barrier (BBB). The use of appropriate nanomaterials for the targeted delivery of drugs or stem cells in which BDNF can be delivered to the lesion to release and maintain its biological activity has become an important scientific issue. Therefore, the identification of suitable carriers that can successfully target the delivery of BDNF will be necessary to develop novel strategies and a theoretical basis for the treatment of various brain diseases.
This paper reviewed the application of nano-drug delivery system loaded BDNF in the treatment of various diseases, which provided a new scientific basis for the development and application of BDNF carriers in the future.
The combined use of BDNF and TrkB enhances the autophosphorylation of TrkB and activates three different signaling pathways. The first is the PI3K/Akt-related pathway. PI3K induces the transcription of the BDNF gene by activating BDNF mRNA, and exerts anti-apoptotic and survival activities. Then, the PI3K/Akt/mTOR cascade regulates protein synthesis and cytoskeleton development, enhancing dendritic growth and branching. The second pathway is the MAPK/Ras pathway, which regulates the signal transduction pathway of protein synthesis and activates Erk/CREB related pathways. The third is the PLC-γ-dependent pathway, which activates calmodulin kinase, and PKC, increasing levels of DAG expression and calcium ion concentration, which in turn increases synaptic plasticity.
The role of BDNF in diseases
The role of BDNF in mental illness and neurodegenerative diseases
Major depressive disorder
Major depressive disorder is a common mental illness. At present, the mechanism by which combinations of antidepressant drugs and their targets produce clinical antidepressant effects is unclear. Studies have shown that BDNF expression is downregulated in patients with depression and that antidepressant drugs can increase the level of BDNF expression (14). When used in combination with TrkB, BDNF can promote neuronal plasticity and antidepressant response, exert cholesterol sensitivity, and mediate the synaptic effect of cholesterol. In addition, typical and fast-acting antidepressants directly bind to TrkB, promoting the synaptic localization of TrkB and the activation of BDNF (15). Conventional antidepressant drugs, including ketamine, require BDNF for the regulation of the antidepressant effects. As a key sensor of antidepressant drugs, BDNF may be a biomarker that can be used to monitor depression treatment response (16). At present, it is generally believed that serotonin receptors are closely associated with the development of depression. Recent studies have found that two oligosaccharide esters (3,6’-deadenosucrose (DISS) and deadenosyl Ester A (TFSA) can be used in combination to effectively enhance the action of the serotonin-BDNF pathway to improve the antidepressant effect (17).
Bipolar disorder
Bipolar disorder is a chronic disease. Bipolar disorder patients experience significant mood changes that fluctuate between mania and depression. Studies have shown that the concentration of BDNF in the serum of patients with bipolar disorder is significantly lower than that of healthy people. After treatment, the concentration of BDNF in the peripheral blood of patients with bipolar disorder was found to be significantly higher than that of healthy people, while the concentration of BDNF in the serum of patients with bipolar disorder was in remission. There was no significant difference in the concentration of BDNF, compared with healthy people (18). Therefore, some studies have suggested that the concentration of BDNF in peripheral serum can be used as an index to determine the condition of patients with bipolar disorder and that the change of the concentration of BDNF in peripheral serum be used as criteria to determine clinical efficacy during the onset of bipolar disorder (19). BDNF levels in bipolar disorder patients were significantly lower than that of the normal control group, which included a healthy control group and a unipolar depression group (20). Valvassori et al. (20) found that the expression of the BDNF gene and its protein in the frontal cortex in an animal model of ouabain-induced bipolar disorder was downregulated. A recent study compared BDNF levels of newly diagnosed bipolar disorder patients with unaffected first-degree relatives and healthy control groups, and found that BDNF levels were downregulated in early-stage bipolar disorder patients but not in unaffected first-degree relatives (21), which points to a new direction for future research.
Schizophrenia
Schizophrenia (SCZ) is a debilitating mental illness with complex, variable, and high genetic characteristics. Imaging studies have found that the volume of the thalamus, hippocampus formation, prefrontal and orbitofrontal cortex, and amygdala-hippocampus complex of patients with schizophrenia were reduced, accompanied by reduced interleukin 1β (IL-1β) and IL-6 expression. The expression of IL-8 and TNF-α were upregulated, while the expression of BDNF and TrkB were significantly downregulated (22). Due to the role played by BDNF in neurogenesis and synaptogenesis and its effect on the function of dopaminergic neurons, it can be used as a biomarker of schizophrenia and its treatment (23). Changes in BDNF expression and Val66Met (rs6562) polymorphism are involved in the pathogenesis of schizophrenia. A new study clinically evaluated 573 patients with chronic schizophrenia. The results showed that the age of onset and cognitive function of the schizophrenia patients was related to changes in clinical characteristics, with serum BDNF levels and BDNF Val66met polymorphisms influencing the age of onset of schizophrenia, cognitive function and clinical symptoms (24). Another study found that patients with schizophrenia and T2DM (type 2 diabetes) showed elevated BDNF levels and improved cognitive function. It was speculated that this change may be related to the pathophysiological processes of chronic SCZ patients with T2DM. Therefore, these results provide a new basis for future research (25).
Alzheimer’s disease
Alzheimer’s disease is a common degenerative disease of the nervous system, which mainly manifests as cognitive dysfunction, for which the cause is still unknown (26). Increasing evidence has shown that BDNF is associated with the etiology and pathogenesis of AD. Studies have found that BDNF exerts a potential protective effect based on neurotoxicity caused by the amyloid β protein (Aβ) in AD mouse models, with the serum and brain BDNF levels in mice (a mouse model of Tauopathy) found to be downregulated (27). A comparative analysis of AD patient blood, cerebrospinal fluid, and post-mortem cranial neurotrophic factor levels with the control group found that peripheral blood BDNF levels of AD patients were significantly lower than those in the control group, while BDNF levels in the hippocampus and neocortex of AD patients were significantly downregulated (28). Recent studies have shown that Aβ can downregulate BDNF levels primarily by downregulating the expression of phosphorylated cyclic adenosine monophosphate (CAMP) response element-binding protein (CREB). Drugs or gene therapy that can target CREB-BDNF signaling may be a novel intervention method that can be used to improve the decline in AD cognitive function (29). Therefore, these findings provide a basis for further research on the biomarkers and therapeutic targets of AD.
Parkinson’s Disease
Parkinson’s disease (PD) is a common neurodegenerative disease among middle-aged and elderly individuals. The main clinical manifestations of PD are reduced movement and resting tremors. A large number of studies have found that PD patients have reduced levels of BDNF expression in the ventral substantia nigra of the brain. Current studies have found that the protein, tyrosine phosphatase 1B (PTP1B) can improve nerve damage by significantly reducing the regulatory effect of interferon γ on inflammatory cytokines and can reverse the 6-hydroxydopamine- (6-OHDA-) induced downregulation of cyclic adenosine phosphate response element-binding protein (p-CREB) and BDNF in SH-SY5Y cells (30). In PD patients, BDNF protects and repairs dopamine neurons, and the BDNFVal66Met gene polymorphism is closely associated with PD (31, 32). Although there is no evidence that BDNF expression is directly associated with PD, these studies may provide new targets and strategies for the treatment of PD.
Epilepsy
Epilepsy is a sudden and dysfunctional brain disease. Studies have found that BDNF and its binding receptor, TrkB, are upregulated in animal models and that in patients with epilepsy, especially in the temporal and hippocampal regions (33). Seizures also can upregulate protein levels of BDNF in the hippocampus and cortex in animal models of epilepsy. Previous studies have shown that BDNF and TrkB molecules are very promising therapeutic targets for the treatment of epilepsy (34, 35). BDNF is closely associated with the pathogenesis of epilepsy, and novel treatment methods that use BDNF can be developed. However, it is worth noting that BDNF may play a role in protecting neurons from harmful stimuli. Therefore, reductions in BDNF levels should be carefully considered when treating epilepsy patients.
The role of BDNF in cardiovascular and cerebrovascular diseases
Stroke results from damage to brain tissue caused by the sudden rupture of blood vessels in the brain or when blood cannot flow into the brain due to clogged blood vessels. Stroke is an acute cerebrovascular disease and is of two types, ischemic stroke, and hemorrhagic stroke. The diagnostic significance and clinical value of BDNF during the acute phase of stroke are controversial (36). Early studies conducted by Be´jot et al. (37) showed that the injection of different doses of microspheres through the carotid artery and into circulation can induce unilateral ischemic stroke in rats, and can be used to simulate the different degrees of stroke severity in stroke patients to explore the significance of circulating BDNF levels in stroke patients. Finally, it was found that circulating BDNF levels after stroke does not reflect BDNF levels during the stroke and that there is a correlation between severe stroke and high plasma BDNF levels during the acute phase. A recent study found that a Chinese herbal medicine used for the treatment of stroke, Yizhi (AOM), can significantly upregulate the expression of BDNF and paromomycin receptor kinase B (TrkB) in the hippocampus, thereby enhancing adult hippocampal neurogenesis and improving MCAO deficiency, thereby enhancing spatial learning, memory and cognitive functions in rats (37). The production of endogenous BDNF in reactive astrocytes is associated with the onset of stroke in hypertensive rats, while exogenous central BDNF causes high blood pressure in hypertensive rats, leading to an increased incidence of stroke. Due to its significant impact on mortality, BDNF may be a candidate drug for the treatment of neurovascular diseases in the future (38). A recent study showed that BDNF was significantly downregulated in patients with coronary artery calcification compared with normal control individuals. BDNF plays an important role in maintaining the stability of vascular endothelial cells and reducing atherosclerosis and can induce the occurrence of coronary atherosclerosis. Therefore, BDNF expression can be used for the prediction of certain clinical conditions (39).
Other applications
In addition to the applications mentioned above, BDNF has also shown good applicability for the treatment of other diseases, such as glaucoma (40), strabismus (41), and irritable bowel syndrome (42). In the future, BDNF can be applied in other forms for the treatment of other diseases than used at present.
Application of a drug delivery system for BDNF
In recent times, BDNF has become a research focus due to its wide range of applications. However, it cannot exert its functions quickly and accurately due to its difficulty in penetrating the blood-brain barrier. However, nano delivery systems and hydrogels can be used to overcome this challenge. Nanoparticles provide a series of unique properties for drug delivery, including high drug loading capacity, combined delivery, controllable and sustained drug release, extended stability and lifespan, and targeted delivery (45) (Figure 2). To further improve the therapeutic index, especially for local applications, nanoparticles have been increasingly combined with hydrogels to form hybrid biomaterial systems for controlled drug delivery, and are now widely used for the treatment of cancer (46, 47), spinal cord injury (48), bone transplantation, and other (49) diseases. In recent years, research on BDNF delivery using nanotechnology and hydrogels has made great progress.
Stem Cell-based BDNF Delivery System
A variety of biomaterials can be used to encapsulate stem cells as a potential cell transplantation strategy for the treatment of neurological diseases (Figure 3). However, an ideal cell delivery material and method is yet to be developed for the clinical treatment of encephalopathy. Previously, researchers used a chitosan (Chitosan) scaffold based on genipin (GP) as a cross-linking agent to fix BDNF with human umbilical cord mesenchymal stem cells (huC-MSCs) for the delivery of BDNF and found that BDNF released by CGB scaffolds (Chitosan-genipin-BDNF) could promote neuronal differentiation of neural stem cells. The CGB scaffold shows high biocompatibility with human umbilical cord mesenchymal stem cells, suggesting that the granular CGB scaffold covering human umbilical cord mesenchymal stem cells may contribute to the development of better treatment methods for traumatic brain injury (50). Subsequent studies have integrated adult pluripotency induced (MIAMI) stem cells isolated from human bone marrow and pharmacologically active microcarriers (PAMs) into an injectable non-toxic silanized hydroxypropyl methylcellulose (SiHPMC). In the hydrogel, an injectable non-toxic cell and growth factor delivery device is used for the delivery of BDNF. Both PAMs and SiHPMC hydrogel can promote the survival of transplanted cells and neuronal differentiation and can transport them into the central nervous system to enhance its tissue repair function. As a novel local delivery tool, it provides a promising treatment method that can be used to promote angiogenesis, neuroprotection and axon growth in the nervous system (51).
BDNF can enhance the proliferation and differentiation of mesenchymal stem cells into osteoblasts. Polyelectrolyte complex nanoparticles (PECNP) have been reported as a suitable drug delivery system. Loy et al. (51) studied the effect of BDNF-loaded PECNP nanoparticles on osteoblasts and found that PECNP is a drug delivery system that is suitable for bone grafts and has no negative effect on bone cell production protein in-vitro. A recent study combined dental stem cells (SCAP) from the tip of the nipple with pharmacologically active microcarriers (PAM) that release BDNF and found that BDNF improved the motor function of rats through immunomodulation and neuroprotection. Subsequently, the poly-L-lactide-glycolide copolymer was used as a solid/oil/water emulsion to coat BDNF, which was then coated with fibronectin to prepare BDNF-PAM, and plant SCAP on it. SCAP-BDNF-PAM injection into the spinal cord injury site of rats can improve the blood-brain barrier score, reduce the expression of inducible nitric oxide synthase, and increase the expression of βⅢ tubulin, GAP43, and 5-HT. These results confirmed the applicability and versatility of PAM as a drug and cell combined drug delivery system in the field of regenerative medicine, and also confirmed the potential therapeutic potential of BDNF-PAMS for the treatment of spinal cord injury (52). Researchers have also embedded human mesenchymal stem cells (hMSCs) into silk fibroin-based hydrogels to produce excess BDNF. BDNF-hMSCs can be transplanted through nasal septal cell transplantation to treat brain injury in rats and was found to significantly reduce the death of hip neurons after injury and promote the recovery of nerve function. Overall, the stem cell transplantation method has laid a good foundation for the clinical applications of BDNF for the treatment of brain injury (53).
Other BDNF delivery systems
To overcome the challenge of the inability of BDNF to pass through the blood-brain barrier, researchers have begun to identify ways to improve the permeability of BDNF. At present, studies have used BDNF and the BBB modulator (BBBM) peptide, ADTC5, to deliver recombinant brain-derived neurotrophic factors to the brains of healthy mice and experimental autoimmune encephalomyelitis (EAE) mice through intravenous injection. The results show that ADTC5 can promote BDNF transmission to the brain of healthy SJL/ELITE mice, and trigger TrkB receptor phosphorylation in the brain. EAE mice treated with BDNF+ADTC5 can inhibit EAE recurrence better than mice treated with BDNF, ADTC5, or the vehicle alone (54). Surfactant-coated polylactic acid-glycolic acid (PLGA) nanoparticles can deliver a variety of molecules across the blood-brain barrier through receptor-mediated endocytosis. Studies have also found that PLGA nanoparticles coated with poloxamer (PX) can effectively deliver BDNF to the brain, improve neurological and cognitive deficits in mice with traumatic brain injury (TBI), and thus exert a neuroprotective effect (55). Lu et al. (56) developed a self-assembled peptide nanofiber hydrogel that delivered both vascular endothelial growth factor (VEGF) and BDNF for peripheral nerve reconstruction. In-vitro cell experiments that compared the use of VEGF or BDNF mimic peptide epitopes alone, found that functionalized peptide hydrogel scaffolds effectively promoted Schwann cell promyelination and endothelial cell adhesion. The use of functionalized self-assembling peptide nanofiber hydrogels to construct the artificial neurovascular microenvironment in diseased areas has great potential in promoting nerve tissue engineering and other forms of tissue regeneration. Therefore, the study of nano delivery systems that can target and release BDNF for a long period is of high importance. Schmidt (56) et al. loaded porous silicon nanoparticles (nano single nucleotide polymorphisms) with a diameter of fewer than 100 nanometers with BDNF and tested the efficiency of a long-term delivery system for neurotrophins, and the results showed a good level of delivery. Lopes et al. (58) used polymer nanoparticles based on thiolated trimethyl chitosan (TMCSH) to mediate the delivery of targeted genes to peripheral neurons through peripheral and minimally invasive intramuscular administration. The addition of non-toxic carboxyl fragment of tetanus neurotoxin (HC) onto nanoparticles was used to achieve neuron targeting and to explore the potential application of plasmid DNA encoding brain-derived neurotrophic factors in peripheral nerve injury models. The study found that TMCSH-HC/BDNF nanoparticle therapy promoted the release and significant expression of BDNF in nerve tissues. The results showed that functional recovery after injury was enhanced and key protective factors were improved.
Currently, BDNF and biomaterials have been widely used for the treatment of many diseases, in particular spinal cord injuries. Some studies have created novel BDNF-loaded cationic nanobubbles (CNBs) by combining MAP-2 antibodies (mAbMAP-2/BDNF/CNBs) for low-intensity focused ultrasound (LIFU) targeted gene therapy. Research has shown that mAbMAP-2/BDNF/CNBs can specifically target neurons, and ultrasound targeting transfection of BDNF overexpressing neurons can effectively inhibit neuronal apoptosis. In a rat model of acute spinal cord injury, mAbMAP-2/BDNF/CNs significantly increased the expression of BDNF, reduced tissue damage and neuron loss, while improving the permeability of the blood-brain barrier (57). Ghosh (58) and others have developed a novel hydrogel-based system that can be loaded with polysaccharide-BDNF particles self-assembled through electrostatic interactions, which can be safely delivered to spinal cord injury sites, while the dosage and administration time can be controlled. A study suggested that local BDNF hydrogel delivery is a very effective and safe strategy that can be used to restore diaphragm function after spinal cord injury. The same research study produced a gelatin-genipin hydrogel system impregnated with ionic liquid that can be injected into the spinal cord injury site of rats. It was found that the survival rate of neurons increased significantly along with the volume of activated microglia and damage was reduced, thereby reducing the level of secondary damage (59). Some researchers have used poly (lactic-glycolic acid copolymer) (PLGA) as a carrier to encapsulate BDNF and create an injectable gel, which can continuously deliver BDNF to the spinal cord injury site for repair (60).
At present, researchers have successfully immobilized insulin-like growth factor-1 (IGF-1) and (BDNF) on biodegradable graphene oxide (GO) composite PLGA (PLGA/GO) to create spinning nanofibers and study their effect on nerve regeneration. The results show that IGF-1 and BDNF loaded on PLGA/GO nanofibers can not only protect NSC from oxidative stress induced by H2O2 but can also promote NSC proliferation and neuronal differentiation. PLGA/GO is an effective carrier for the delivery of IGF-1 and BDNF, and that IGF-1 and BDNF immobilized on PLGA/GO nanofibers can function as neural implants, showing a high potential for the treatment of spinal cord injury (61). Another study evaluated the release potential of BDNF from IKVAV functionalized peptide amphiphilic (PA) self-assembled nanofibers and hydrogels, and used IKVAV-PA hydrogels loaded with BDNF to perform treatment on mice with severe spinal cord injury. The treatment results showed that IKVAV-PA is arranged into a nanofiber structure and can continuously release BDNF while retaining the biological activity of BDNF. Injection of IKVAV-PA hydrogel containing BDNF can increase axon retention and decrease levels of astrocyte proliferation after spinal cord injury without any inflammatory response. These results suggest that biofunctional peptide-based hydrogel can be used as an injectable scaffold for BDNF delivery to promote regeneration following spinal cord injury (62). Researchers have also developed a self-assembled peptide nanofiber hydrogel based on the self-assembled backbone Ac- (RADA)4-NH2 (RAD), which uses laminin-derived motifs IKVAV (IKV) and BDNF. The mimetic peptide epitope RGIDKRHWNSQ (RGI) performs the dual functions of peripheral nerve regeneration and enhanced remyelination, as well as motor function recovery (63).
The latest study conducted on BDNF application used polymer-based polyethylene glycol-polyaspartic acid (DET) nano micelles and its derivatives to administer BDNF-encoded mRNA into the brain, which significantly increased transient whole brain expression of BDNF. The results showed an increase in the survival rate of hippocampal neurons after ischemia (TGI) and a rapid increase in BDNF content in the hippocampus after administration. The BNDF-mRNA introduced through the use of multiple types of nano glue can effectively treat ischemic neuronal death (64). Due to the limitation of the BBB for drug delivery to the central nervous system (CNS) and the safety of intracerebral administration, the intranasal route of administration has recently received extensive attention. Some researchers have dissolved BDNF in PBS and administered it as a non-invasive nasal injection to AD11 mice, and have found that it can effectively improve the memory performance of the experimental mice. This increases the potential therapeutic use of BDNF for the treatment of neurological diseases and enhances its potential administration through non-invasive intranasal pathways as a brain delivery strategy for BDNF and other neurotrophic factors (65). This study used minimally invasive nose bank (Mind) technology to deliver the entire therapeutic dose directly to the sub-olfactory submucosal space through nasal administration, which overcomes dose variability and efficiency-related challenges faced during traditional local transnasal administration. The study found that implanting a reservoir containing AntagoNAT (AT), which can downregulate the expression of which can down regulate the expression of BDNF, significantly increased the central nervous system distribution of AT and continue to upregulate BDNF. In the future, this technology can be used at treatment centers as a potential treatment method for neurological diseases (66).
Previously, researchers had used hyaluronic acid hydrogel loaded with BDNF for nerve repair in patients at the chronic phase after stroke, and found that BDNF can be released locally from the infarct cavity. In two mouse stroke models, the duration of the spread of BDNF delivered through a hydrogel from the stroke cavity to the surrounding tissues of the infarction was longer than that of a simple injection of BDNF, and the administration of the BDNF hydrogel was able to promote the recovery of motor function (67). Jimbo et al. (70) used high molecular weight hyaluronic acid (HMW-HA) and BDN to study the regenerative effect of BDNF on bifurcation defects in non-human primate models. The study found that the periodontal tissue regeneration level and the formation of acellular cementum were significantly higher in the BDNF and HMW-HA combined treatment group than that in the BDNF alone group.
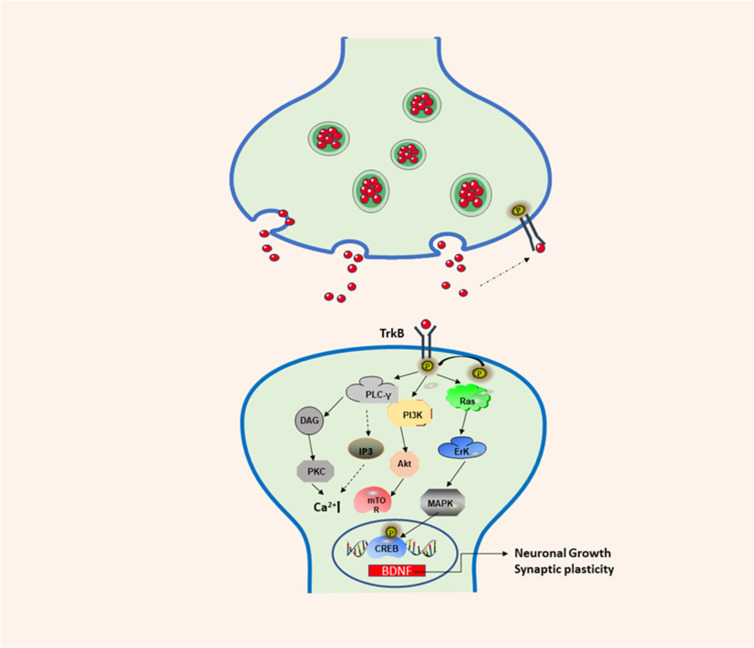
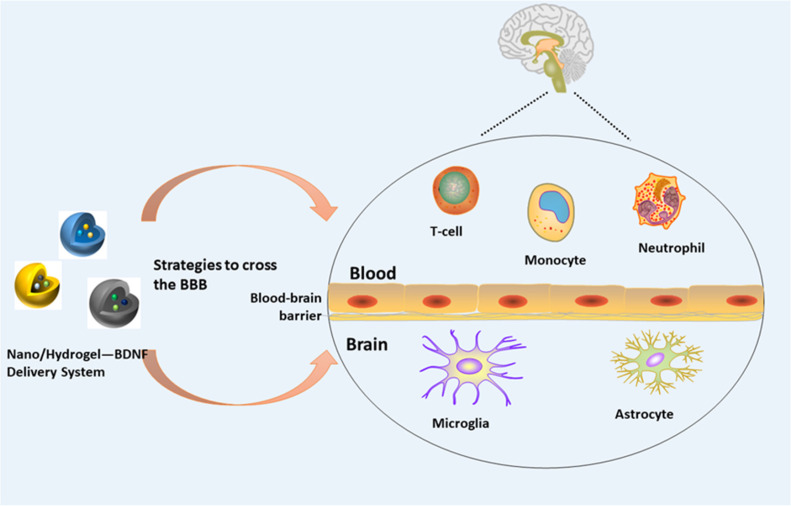
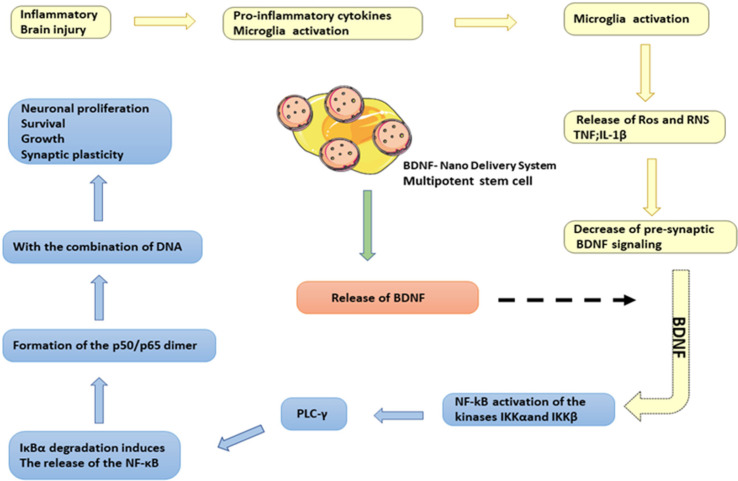
The performance of brain-derived neurotrophic factors in diseases
Type of disease | BDNF | references |
---|---|---|
Major depressive disorder | The levels of BDNF in the serum and brain of patients with depression are downregulated; BDNF can be used in combination with TrkB; traditional antidepressants regulate the level of BDNF | (14-16) |
Bipolar disorder | The level of BDNF in the serum is downregulated during the attack period; the level of BDNF expression at gene and protein levels is also downregulated in the frontal cortex | (18, 43) |
Schizophrenia | The expression of the BDNF gene is downregulated in the hippocampus, thalamus, and frontal cortex of patients; BDNFVal66Met gene polymorphism is involved in the pathogenesis | (22, 24) |
Alzheimer's disease | The level of BDNF in the serum is downregulated; the level of BDNF mRNA in the hippocampus is also downregulated | (27, 28) |
Parkinson | The BDNF content of the ventral substantia nigra of the brain is downregulated in patients; BDNFVal66Met gene polymorphism is closely associated with PD | (30-32) |
Epilepsy | Seizures increase protein levels of BDNF in the hippocampus and cortex | (34, 35) |
Cardiovascular | The diagnostic significance and clinical value of BDNF during the acute phase of stroke are still controversial; the severe stroke is associated with high plasma BDNF levels during the acute phase; exogenous central BDNF expression increases blood pressure in hypertensive rats; BDNF levels are significantly downregulated in patients with coronary artery calcification | (37-39, 44) |
Delivery systems for BDNF using hydrogel/nanomaterials
Disease application | Hydrogel/Nanomaterial | BDNF system | References |
---|---|---|---|
Traumatic brain injury and recovery after injury | Chitosan-Genipin; Silk Fibroin (SF); Polylactic Acid-Glycolic Acid (PLGA); Thiolated Trimethyl Chitosan (TMCSH) | Chitosan-genipin-BDNF; | (50, 53, 55 and 68) |
Promote angiogenesis, neuroprotection and axon growth | Silanized hydroxypropyl methylcellulose (Si-HPMC) and pharmacologically active carrier (PAM); self-assembled peptide nanofiber hydrogel- (Beaver Nano RAD); | PAM-Si-HPMC-BDNF; RAD-BDNF | (51, 69) |
Rat motor function | Polyelectrolyte composite nanoparticles (PECNP) | PECN-BDNF | (70) |
Spinal cord injury | Precipitation of poly-L-lactide-glycolide copolymer, and then combined with pharmacologically active microcarriers (PAM); cationic nanobubbles (CNBs); polysaccharides; polylactic acid-glycolic acid copolymers (PLGA); graphene oxide ( GO) Composite PLGA (PLGA/GO) electrospun nano; IKVAV functionalized amphiphilic PA self-assembled nano; Ac- (RADA)4-NH2 (RAD) self-assembled peptide nanofiber hydrogel | BDNF-PAMS; Combined MAP-2 antibody mAb MAP-2-BDNF-CNBs; Polysaccharide-BDNF; PLGA-BDNF; PLGA/GO-BDNF; IKVAV-BDNF; c- (RADA) 4-NH2 (RAD) -BDNF; | (52, 57, 58 and 60-63) |
Ischemic neurodeath | Polyethylene glycol-polyaspartic acid (DET) nano micelles and their derivatives | PEG-PAsp (DET)-BDNF | (64) |
Stroke | Hyaluronic acid | Hyaluronic acid-BDNF | (67) |
Periodontal regeneration | Hyaluronic acid HMW-HA | HMW-HA-BDNF | (71) |
Conclusion
Since BDNF plays a key role in the development and maturation of neurons, it has become the main therapeutic drug target for many neurological diseases. A large number of studies have shown that BDNF has shown potential therapeutic value in many neurodegenerative disease models and acute central nervous system damage. However, due to transmission challenges associated with this molecule, its clinical application is not perfect. In addition, the positive effects of psychotropic drugs can activate BDNF-mediated signal transmission. Although the BDNF gene is associated with many diseases, in addition to functional genetic variation, other molecular mechanisms may also affect the regulation of the expression of the BDNF gene, resulting in BDNF signal transduction disorders and related pathological changes. BDNF plays a key role in synaptic plasticity, partly due to changes in local protein synthesis. The activation of BDNF by TrkB can trigger multiple parallel signaling pathways, such as the RAS/ERK, phosphatidylinositol 3-kinase, and phospholipase C-γ pathways. Although BDNF can regulate certain signaling mechanisms of translation activity by regulating the initiation and expansion stages, changes in the proteome caused by BDNF have not been verified.
To overcome the challenge of poor blood-brain barrier permeability, bone marrow mesenchymal stem cells can be used as a delivery platform for BDNF to provide broad prospects for clinical neurotrophic factor repair. As in-depth research continues to be conducted on nano-bio materials, an increasing number of researchers have aimed to identify hydrogel materials and nanotechnology that can be used for the targeted delivery of BDNF, to achieve rapid, accurate, safe and continuous delivery of BDNF. At present, a variety of nanosystems, such as self-assembled nanometers, biodegradable materials, and injectable gels, have been applied for the delivery of BDNF. However, current disease models can usually only simulate certain symptoms, and cannot accurately reproduce complex human diseases. Therefore, intrathecal injection of BDNF into the brain or spinal cord of patients as an intervention is still accompanied by many challenges. The studies and investigations detailed above provide novel methods and a foundation for the application of BDNF for the treatment of various diseases in the future.
Conflicts of Interest
The authors declare that there is no conflict of interest.
Acknowledgements
References
-
1.
Murer MG, Yan Q, Raisman-Vozari R. Brain-derived neurotrophic factor in the control human brain, and in Alzheimer’s disease and Parkinson’s disease. Prog. Neurobiol. 2001;63:71-124. [PubMed ID: 11040419].
-
2.
Tapia-Arancibia L, Aliaga E, Silhol M, Arancibia S. New insights into brain BDNF function in normal aging and Alzheimer disease. Brain Res. Rev. 2008;59:201-20. [PubMed ID: 18708092].
-
3.
Conner JM, Lauterborn JC, Yan Q, Gall CM, Varon S. Distribution of brain-derived neurotrophic factor (BDNF) protein and mRNA in the normal adult rat CNS: evidence for anterograde axonal transport. J. Neurosci. 1997;17:2295-313. [PubMed ID: 9065491].
-
4.
Kerschensteiner M, Gallmeier E, Behrens L, Leal VV, Misgeld T, Klinkert WE, Kolbeck R, Hoppe E, Oropeza-Wekerle RL, Bartke I, Stadelmann C, Lassmann H, Wekerle H, Hohlfeld R. Activated human T cells, B cells, and monocytes produce brain-derived neurotrophic factor in vitro and in inflammatory brain lesions: a neuroprotective role of inflammation? J. Exp. Med. 1999;189:865-70.
-
5.
Yarrow JF, White LJ, McCoy SC, Borst SE. Training augments resistance exercise induced elevation of circulating brain derived neurotrophic factor (BDNF). Neurosci. Lett. 2010;479:161-5. [PubMed ID: 20553806].
-
6.
Lu B, Nagappan G, Lu Y. BDNF and synaptic plasticity, cognitive function, and dysfunction. Handb. Exp. Pharmacol. 2014;220:223-50. [PubMed ID: 24668475].
-
7.
Sasi M, Vignoli B, Canossa M, Blum R. Neurobiology of local and intercellular BDNF signaling. Pflug. Arch. Eur. J. Physiol. 2017;469:593-610.
-
8.
Kowiański P, Lietzau G, Czuba E, Waśkow M, Steliga A, Moryś J. BDNF: A Key Factor with Multipotent Impact on Brain Signaling and Synaptic Plasticity. Cell. Mol. Neurobiol. 2018;38:579-93. [PubMed ID: 28623429].
-
9.
Lima Giacobbo B, Doorduin J, Klein HC, Dierckx R, Bromberg E, de Vries EFJ. Brain-Derived Neurotrophic Factor in Brain Disorders: Focus on Neuroinflammation. Mol. Neurobiol. 2019;56:3295-312. [PubMed ID: 30117106].
-
10.
Minichiello L. TrkB signalling pathways in LTP and learning. Nat. Rev. Neurosci. 2009;10:850-60. [PubMed ID: 19927149].
-
11.
Barker PA. Whither proBDNF? Nat. Neurosci. 2009;12:105-6.
-
12.
Hanson IM, Seawright A, van Heyningen V. The human BDNF gene maps between FSHB and HVBS1 at the boundary of 11p13-p14. Genomics. 1992;13:1331-3. [PubMed ID: 1505967].
-
13.
Autry AE, Monteggia LM. Brain-derived neurotrophic factor and neuropsychiatric disorders. Pharmacol. Rev. 2012;64:238-58. [PubMed ID: 22407616].
-
14.
Ryan KM, Dunne R, McLoughlin DM. BDNF plasma levels and genotype in depression and the response to electroconvulsive therapy. Brain Stimul. 2018;11:1123-31. [PubMed ID: 29802070].
-
15.
Casarotto PC, Girych M, Fred SM, Kovaleva V, Moliner R, Enkavi G, Biojone C, Cannarozzo C, Sahu MP, Kaurinkoski K, Brunello CA, Steinzeig A, Winkel F, Patil S, Vestring S, Serchov T, Diniz C, Laukkanen L, Cardon I, Antila H, Rog T, Piepponen TP, Bramham CR, Normann C, Lauri SE, Saarma M, Vattulainen I, Castrén E. Antidepressant drugs act by directly binding to TRKB neurotrophin receptors. Cell. 2021;184:1299-313. [PubMed ID: 33606976].
-
16.
Björkholm C, Monteggia LM. BDNF - a key transducer of antidepressant effects. Neuropharmacology. 2016;102:72-9. [PubMed ID: 26519901].
-
17.
Yang WS, Shi ZG, Dong XZ, Liu P, Chen ML, Hu Y. Involvement of 5-HT-BDNF signaling axis in mediating synergistic antidepressant-like effects after combined administration of two oligosaccharide esters. Food Sci. Nutr. 2021;9:1180-91. [PubMed ID: 33598202].
-
18.
Munkholm K, Vinberg M, Kessing LV. Peripheral blood brain-derived neurotrophic factor in bipolar disorder: a comprehensive systematic review and meta-analysis. Mol. Psychiatry. 2016;21:216-28. [PubMed ID: 26194180].
-
19.
Lin PY. State-dependent decrease in levels of brain-derived neurotrophic factor in bipolar disorder: a meta-analytic study. Neurosci. Lett. 2009;466:139-43. [PubMed ID: 19786073].
-
20.
Rowland T, Perry BI, Upthegrove R, Barnes N, Chatterjee J, Gallacher D, Marwaha S. Neurotrophins, cytokines, oxidative stress mediators and mood state in bipolar disorder: systematic review and meta-analyses. Br. J. Psychiatry. 2018;213:514-25. [PubMed ID: 30113291].
-
21.
Petersen NA, Nielsen M, Coello K, Stanislaus S, Melbye S, Kjærstad HL, Sletved KSO, McIntyre RS, Frikke-Smith R, Vinberg M, Kessing LV. Brain-derived neurotrophic factor levels in newly diagnosed patients with bipolar disorder, their unaffected first-degree relatives and healthy controls. BJPsych Open. 2021;7:55.
-
22.
Mohammadi A, Rashidi E, Amooeian VG. Brain, blood, cerebrospinal fluid, and serum biomarkers in schizophrenia. Psychiatry Res. 2018;265:25-38. [PubMed ID: 29680514].
-
23.
Libman-Sokołowska M, Drozdowicz E, Nasierowski T. BDNF as a biomarker in the course and treatment of schizophrenia. Psychiatr Pol. 2015;49:1149-58. [PubMed ID: 26909392].
-
24.
Xu H, Wang J, Zhou Y, Chen D, Xiu M, Wang L, Zhang X. BDNF affects the mediating effect of negative symptoms on the relationship between age of onset and cognition in patients with chronic schizophrenia. Psychoneuroendocrinology. 2021;125:105121.
-
25.
Li S, Chen D, Xiu M, Li J, Zhang XY. Diabetes mellitus, cognitive deficits and serum BDNF levels in chronic patients with schizophrenia: A case-control study. J. Psychiatr. Res. 2021;134:39-47. [PubMed ID: 33360223].
-
26.
Soria Lopez JA, González HM, Léger GC. Alzheimer’s disease. Handb. Clin. Neurol. 2019;167:231-55. [PubMed ID: 31753135].
-
27.
Jiao SS, Shen LL, Zhu C, Bu XL, Liu YH, Liu CH, Yao XQ, Zhang LL, Zhou HD, Walker DG, Tan J, Götz J, Zhou XF, Wang YJ. Brain-derived neurotrophic factor protects against tau-related neurodegeneration of Alzheimer’s disease. Transl. Psychiatry. 2016;6:907.
-
28.
Du Y, Wu HT, Qin XY, Cao C, Liu Y, Cao ZZ, Cheng Y. Postmortem Brain, Cerebrospinal Fluid, and Blood Neurotrophic Factor Levels in Alzheimer’s Disease: A Systematic Review and Meta-Analysis. J. Mol. Neurosci. 2018;65:289-300. [PubMed ID: 29956088].
-
29.
Amidfar M, de Oliveira J, Kucharska E, Budni J, Kim YK. The role of CREB and BDNF in neurobiology and treatment of Alzheimer’s disease. Life Sci. 2020;257:118020.
-
30.
Feng CW, Chen NF, Chan TF, Chen WF. Therapeutic role of protein tyrosine phosphatase 1B in parkinson’s disease via antineuroinflammation and neuroprotection in-vitro and in-vivo. Parkinsons Dis. 2020;2020:8814236. [PubMed ID: 33456749].
-
31.
Palasz E, Wysocka A, Gasiorowska A, Chalimoniuk M, Niewiadomski W, Niewiadomska G. BDNF as a Promising Therapeutic Agent in Parkinson’s Disease. Int. J. Mol. Sci. 2020;21:1170.
-
32.
Ramezani M, Ruskey JA, Martens K, Kibreab M, Javer Z, Kathol I, Hammer T, Cheetham J, Leveille E, Martino D, Sarna JR, Gan-Or Z, Pfeffer G, Ismail Z, Monchi O. Association Between BDNF Val66Met Polymorphism and Mild Behavioral Impairment in Patients With Parkinson’s Disease. Front. Neurol. 2020;11:587992.
-
33.
Iughetti L, Lucaccioni L, Fugetto F, Predieri B, Berardi A, Ferrari F. Brain-derived neurotrophic factor and epilepsy: a systematic review. Neuropeptides. 2018;72:23-9. [PubMed ID: 30262417].
-
34.
Fernández-García S, Sancho-Balsells A, Longueville S, Hervé D, Gruart A, Delgado-García JM, Alberch J, Giralt A. Astrocytic BDNF and TrkB regulate severity and neuronal activity in mouse models of temporal lobe epilepsy. Cell Death Dis. 2020;11:411. [PubMed ID: 32483154].
-
35.
Lin TW, Harward SC, Huang YZ, McNamara JO. Targeting BDNF/TrkB pathways for preventing or suppressing epilepsy. Neuropharmacology. 2020;167:107734. [PubMed ID: 31377199].
-
36.
French MA, Morton SM, Pohlig RT, Reisman DS. The relationship between BDNF Val66Met polymorphism and functional mobility in chronic stroke survivors. Top Stroke Rehabil. 2018;25:276-80. [PubMed ID: 29480080].
-
37.
He Y, Chen S, Tsoi B, Qi S, Gu B, Wang Z, Peng C, Shen J. Alpinia oxyphylla Miq and Its Active Compound P-Coumaric Acid Promote Brain-Derived Neurotrophic Factor Signaling for Inducing Hippocampal Neurogenesis and Improving Post-cerebral Ischemic Spatial Cognitive Functions. Front. Cell. Dev. Biol. 2020;8:577790. [PubMed ID: 33537297].
-
38.
Hasegawa Y, Takemoto Y, Hayashi K, Kameno K, Kim-Mitsuyama S. The endogenous and exogenous brain-derived neurotrophic factor plays pivotal roles in the pathogenesis of stroke onset in high salt-loaded hypertensive rats. Exp. Gerontol. 2021;147:111286. [PubMed ID: 33609688].
-
39.
Jin H, Ji JJ, Zhu Y, Wang XD, Li YP, Shi QY, Chen YF. Brain-Derived Neurotrophic Factor, a New Predictor of Coronary Artery Calcification. Clin. Appl. Thromb. Hemost. 2021;27:1076029621989810. [PubMed ID: 33523719].
-
40.
Dekeyster E, Geeraerts E, Buyens T, Van den Haute C, Baekelandt V, De Groef L, Salinas-Navarro M, Moons L. Tackling Glaucoma from within the Brain: An Unfortunate Interplay of BDNF and TrkB. PLoS One. 2015;10:142067.
-
41.
Willoughby CL, Fleuriet J, Walton MM, Mustari MJ, McLoon LK. Adaptation of slow myofibers: the effect of sustained BDNF treatment of extraocular muscles in infant nonhuman primates. Invest. Ophthalmol. Vis. Sci. 2015;56:3467-83. [PubMed ID: 26030102].
-
42.
Konturek TJ, Martinez C, Niesler B, van der Voort I, Mönnikes H, Stengel A, Goebel-Stengel M. The Role of Brain-Derived Neurotrophic Factor in Irritable Bowel Syndrome. Front. Psychiatry. 2020;11:531385. [PubMed ID: 33519536].
-
43.
Valvassori SS, Dal-Pont GC, Varela RB, Resende WR, Gava FF, Mina FG, Budni J, Quevedo J. Ouabain induces memory impairment and alter the BDNF signaling pathway in an animal model of bipolar disorder: Cognitive and neurochemical alterations in BD model. J. Affect. Disord. 2021;282:1195-202. [PubMed ID: 33601696].
-
44.
Béjot Y, Mossiat C, Giroud M, Prigent-Tessier A, Marie C. Circulating and brain BDNF levels in stroke rats. Relevance to clinical studies.PLoS One. 2011;6:29405.
-
45.
Furtado D, Björnmalm M, Ayton S, Bush AI, Kempe K, Caruso F. Overcoming the Blood-Brain Barrier: The Role of Nanomaterials in Treating Neurological Diseases. Adv. Mater. 2018;30:1801362.
-
46.
Caldas BS, Nunes CS, Panice MR, Scariot DB, Nakamura CV, Muniz EC. Manufacturing micro/nano chitosan/chondroitin sulfate curcumin-loaded hydrogel in ionic liquid: A new biomaterial effective against cancer cells. Int. J. Biol. Macromol. 2021;180:88-96. [PubMed ID: 33657414].
-
47.
Saydé T, El Hamoui O, Alies B, Gaudin K, Lespes G, Battu S. Biomaterials for Three-Dimensional Cell Culture: From Applications in Oncology to Nanotechnology. Nanomaterials. 2021;11:481. [PubMed ID: 33668665].
-
48.
Keefe KM, Sheikh IS, Smith GM. Targeting Neurotrophins to Specific Populations of Neurons: NGF, BDNF, and NT-3 and Their Relevance for Treatment of Spinal Cord Injury. Int. J. Mol. Sci. 2017;18:548.
-
49.
Shi Z, Xu Y, Mulatibieke R, Zhong Q, Pan X, Chen Y, Lian Q, Luo X, Shi Z, Zhu Q. Nano-Silicate-Reinforced and SDF-1α-Loaded Gelatin-Methacryloyl Hydrogel for Bone Tissue Engineering. Int. J. Nanomed. 2020;15:9337-53.
-
50.
Shi W, Nie D, Jin G, Chen W, Xia L, Wu X, Su X, Xu X, Ni L, Zhang X, Zhang X, Chen J. BDNF blended chitosan scaffolds for human umbilical cord MSC transplants in traumatic brain injury therapy. Biomaterials. 2012;33:3119-26. [PubMed ID: 22264526].
-
51.
Kandalam S, Sindji L, Delcroix GJ, Violet F, Garric X, André EM, Schiller PC, Venier-Julienne MC, des Rieux A, Guicheux J, Montero-Menei CN. Pharmacologically active microcarriers delivering BDNF within a hydrogel: Novel strategy for human bone marrow-derived stem cells neural/neuronal differentiation guidance and therapeutic secretome enhancement. Acta Biomater. 2017;49:167-80. [PubMed ID: 27865962].
-
52.
Kandalam S, De Berdt P, Ucakar B, Vanvarenberg K, Bouzin C, Gratpain V, Diogenes A, Montero-Menei CN, des Rieux A. Human dental stem cells of the apical papilla associated to BDNF-loaded pharmacologically active microcarriers (PAMs) enhance locomotor function after spinal cord injury. Int. J. Pharm. 2020;587:119685. [PubMed ID: 32712253].
-
53.
Sultan MT, Choi BY, Ajiteru O, Hong DK, Lee SM, Kim HJ, Ryu JS, Lee JS, Hong H, Lee YJ, Lee H, Suh YJ, Lee OJ, Kim SH, Suh SW, Park CH. Reinforced-hydrogel encapsulated hMSCs towards brain injury treatment by trans-septal approach. Biomaterials. 2021;266:120413.
-
54.
Kopec BM, Kiptoo P, Zhao L, Rosa-Molinar E, Siahaan TJ. Noninvasive Brain Delivery and Efficacy of BDNF to Stimulate Neuroregeneration and Suppression of Disease Relapse in EAE Mice. Mol. Pharm. 2020;17:404-16. [PubMed ID: 31846344].
-
55.
Khalin I, Alyautdin R, Wong TW, Gnanou J, Kocherga G, Kreuter J. Brain-derived neurotrophic factor delivered to the brain using poly (lactide-co-glycolide) nanoparticles improves neurological and cognitive outcome in mice with traumatic brain injury. Drug Deliv. 2016;23:3520-8. [PubMed ID: 27278330].
-
56.
Schmidt N, Schulze J, Warwas DP, Ehlert N, Lenarz T, Warnecke A, Behrens P. Long-term delivery of brain-derived neurotrophic factor (BDNF) from nanoporous silica nanoparticles improves the survival of spiral ganglion neurons in vitro. PLoS One. 2018;13:194778.
-
57.
Song Z, Ye Y, Zhang Z, Shen J, Hu Z, Wang Z, Zheng J. Noninvasive, targeted gene therapy for acute spinal cord injury using LIFU-mediated BDNF-loaded cationic nanobubble destruction. Biochem. Biophys. Res. Commun. 2018;496:911-20. [PubMed ID: 29360450].
-
58.
Ghosh B, Wang Z, Nong J, Urban MW, Zhang Z, Trovillion VA, Wright MC, Zhong Y, Lepore AC. Local BDNF Delivery to the Injured Cervical Spinal Cord using an Engineered Hydrogel Enhances Diaphragmatic Respiratory Function. J. Neurosci. 2018;38:5982-95. [PubMed ID: 29891731].
-
59.
Bhattacharyya S, Dinda A, Vishnubhatla S, Anwar MF, Jain S. A combinatorial approach to modulate microenvironment toward regeneration and repair after spinal cord injury in rats. Neurosci. Lett. 2021;741:135500. [PubMed ID: 33197520].
-
60.
Huang F, Chen T, Chang J, Zhang C, Liao F, Wu L, Wang W, Yin Z. A conductive dual-network hydrogel composed of oxidized dextran and hyaluronic-hydrazide as BDNF delivery systems for potential spinal cord injury repair. Int. J. Biol. Macromol. 2021;167:434-45. [PubMed ID: 33278434].
-
61.
Pan S, Qi Z, Li Q, Ma Y, Fu C, Zheng S, Kong W, Liu Q, Yang X. Graphene oxide-PLGA hybrid nanofibres for the local delivery of IGF-1 and BDNF in spinal cord repair. Artif. Cells Nanomed. Biotechnol. 2019;47:651-64. [PubMed ID: 30829545].
-
62.
Hassannejad Z, Zadegan SA, Vaccaro AR, Rahimi-Movaghar V, Sabzevari O. Biofunctionalized peptide-based hydrogel as an injectable scaffold for BDNF delivery can improve regeneration after spinal cord injury. Injury. 2019;50:278-85. [PubMed ID: 30595411].
-
63.
Yang S, Wang C, Zhu J, Lu C, Li H, Chen F, Lu J, Zhang Z, Yan X, Zhao H, Sun X, Zhao L, Liang J, Wang Y, Peng J, Wang X. Self-assembling peptide hydrogels functionalized with LN- and BDNF- mimicking epitopes synergistically enhance peripheral nerve regeneration. Theranostics. 2020;10:8227-49. [PubMed ID: 32724468].
-
64.
Fukushima Y, Uchida S, Imai H, Nakatomi H, Kataoka K, Saito N, Itaka K. Treatment of ischemic neuronal death by introducing brain-derived neurotrophic factor mRNA using polyplex nanomicelle. Biomaterials. 2021;270:120681. [PubMed ID: 33517206].
-
65.
Braschi C, Capsoni S, Narducci R, Poli A, Sansevero G, Brandi R, Maffei L, Cattaneo A, Berardi N. Intranasal delivery of BDNF rescues memory deficits in AD11 mice and reduces brain microgliosis. Aging Clin. Exp. Res. 2021;33:1223-38. [PubMed ID: 32676979].
-
66.
Padmakumar S, Jones G, Pawar G, Khorkova O, Hsiao J, Kim J, Amiji MM, Bleier BS. Minimally Invasive Nasal Depot (MIND) technique for direct BDNF AntagoNAT delivery to the brain. J. Control. Release. 2021;331:176-86. [PubMed ID: 33484777].
-
67.
Cook DJ, Nguyen C, Chun HN, I LL, Chiu AS, Machnicki M, Zarembinski TI, Carmichael ST. Hydrogel-delivered brain-derived neurotrophic factor promotes tissue repair and recovery after stroke. J. Cereb. Blood Flow Metab. 2017;37:1030-45. [PubMed ID: 27174996].
-
68.
Lopes CDF, Gonçalves NP, Gomes CP, Saraiva MJ, Pêgo AP. BDNF gene delivery mediated by neuron-targeted nanoparticles is neuroprotective in peripheral nerve injury. Biomaterials. 2017;121:83-96. [PubMed ID: 28081461].
-
69.
Lu J, Yan X, Sun X, Shen X, Yin H, Wang C, Liu Y, Lu C, Fu H, Yang S, Wang Y, Sun X, Zhao L, Lu S, Mikos AG, Peng J, Wang X. Synergistic effects of dual-presenting VEGF- and BDNF-mimetic peptide epitopes from self-assembling peptide hydrogels on peripheral nerve regeneration. Nanoscale. 2019;11:19943-58. [PubMed ID: 31602446].
-
70.
Loy TL, Vehlow D, Kauschke V, Müller M, Heiss C, Lips KS. Effects of BDNF and PEC Nanoparticles on Osteocytes. Molecules. 2020;25:4151.
-
71.
Jimbo R, Singer J, Tovar N, Marin C, Neiva R, Bonfante EA, Janal MN, Contamin H, Coelho PG. Regeneration of the cementum and periodontal ligament using local BDNF delivery in class II furcation defects. J. Biomed. Mater. Res. Appl. Biomater. 2018;106:1611-7.