Abstract
Keywords
Introduction
Aggressive breast cancer is the cause of ten thousand of death in women, annually (1). The disease is characterized by an accumulation of abnormal epithelial cells as a result of multistep genetic alterations in the initiating cells. According to the World Health Organization (WHO) classification, there are several criteria for breast cancer classification (2). One of the known criteria is based on estrogen receptor (ER), progesterone receptor (PR), and human epithelial receptor 2 (HER2) statuses. Response to chemotherapy in metastatic breast cancer is controversial since unlike other solid tumors, estrogen receptor positive (ER+) breast cancer patients with skeletal metastasis showed beneficial responses to chemotherapy demonstrating favourable prognosis (3, 4). Unfortunately, this hypothesis was not well established in patients with ER- breast cancer and/or patients with skeletal metastasis (4).
Two known breast cancer cell lines, which were studied in several papers, are MCF-7 and MDA-MB-468 cell lines. MDA-MB-468 is a basal type triple-negative (ER, PR and HER2 negative) breast cancer cell line while MCF7 is the luminal type expressing estrogen and progesterone receptors (5). MDA-MB-468 cell line has genomic instability because of mutation in BRCA or TP53 gene. MCF-7 and MDA-MB-468 cell lines are characterized by uncontrolled proliferation, indefinite self-renewal, impaired differentiation, and inhibits apoptosis. Furthermore, in cancers, one of the stem cell features is indefinite self-renewal. Among the most important modulators involved in controlling the self-renewal of stem cells is NS (NS) (6). NS or GTP-binding protein is present in nucleoli, which is thought to regulate stem cell fate via the activation of the p53-dependent pathway (7), although some p53-independent mechanisms have been reported in cancerous cells (8).
In 2013, a study reported that NS depletion resulted in sphere-forming activity in MCF-7 and MDA-MB-231 cell lines. Several studies showed that senescence and apoptosis, cell cycle arrest, and eventually, embryonic death at the blastocyst stage are affected by the deletion of NS in mouse embryonic stem cells (ESCs) (9). Also, some studies showed that knockdown of NS expression in cancerous cells could induce apoptosis and cell cycle arrest (10). Therefore, NS appears to play an important role in cell cycle progression in neural stem cells (NSCs) (11), embryonic stem cells (ESCs)(9), and various cancer cell lines (12, 13). Recently, scientists reported the high level of NS expression in MCF-7 and breast cancer tissue, suggesting that this nuclear protein might be an attractive molecular target for developing tumor cells. We designed this study to better understand the association between NS expression status and apoptosis induction in both MCF-7 and MDA-MB-468 cells.
Experimental
Cell line and cell culture
MCF-7 and MDA-MB-231 cell lines were purchased from the cell repository of the research institute of biotechnology (Ferdowsi University of Mashhad, Iran). Cells were cultured in RPMI1640 medium with 10% fetal bovine serum (FBS) (Rockville, MD, USA), 100 μg/mL streptomycin, 100 U/mL penicillin (Sigma) and maintained at 37 °C in a humidified atmosphere with 5% CO2 until reaching 80% of confluency.
siRNAs
The siRNAs targeted human NS (Cat.no: SI04319504, SI04298938, SI04211872 and SI03169593, 20 µM) and scrambled negative siRNA (SI03650325, 20 µM) were purchased from Qiagen company (USA). The lyophilized siRNAs were dissolved in RNase free water to a final concentration of 20 µM. siRNA transfection was performed using HiPerFect transfection reagent (Qiagen, Cat No: 301704, USA), as described in the manufacturer’s instructions. In order to find the optimum concentrations of siRNA, a 0.2 µM siRNA stock was prepared from a 20 µM stock. Briefly, different concentrations of 0.2 µM siRNA stock were spotted in each well of 6-wells plates for qPCR and apoptosis assays (Zhejiang Sorfa Medical Plastic Co., Ltd, Zhejiang, China). Then, 12 μL of HiPerFect transfection reagent (Qiagen, USA) was mixed with 100 μL of RPMI1640 without FBS, and the mixture incubated for 15 min at room temperature. The resulting mixture was added dropwise into each well. Finally, 100,000 cells/well in 2300 μL of 10% FBS-contained RPMI1640 medium were added to each well and were allowed to adhere overnight (reverse transfection. The cells were incubated for 6 to72 h after transfection (in 5% CO2, 95% humidified air at 37 °C) before additional analyses.
Real-time quantitative PCR (q-PCR)
Total RNA was extracted using the RNX plus kit (Cinagen, Tehran). The RNA converted to cDNA using RT-PCR kit from Takara (TaKaRa, Dalian, China). The RNA quality and integrity assessed by NanoDrop (Eppendorf, USA) and Gel electrophoresis. AlleleID software and NCBI-BLAST database were employed in order to design the primers and verify their specificity. The efficiency of primers was validated and a standard curve plotted before running PCR products on 2% agarose gel. RT-qPCR performed using SYBR Green Real-time PCR (Fermentas, USA) by 96-wells ABI machine (Applied Biosystems) under following cycle conditions: 95 °C for 10 min, followed by40 cycles at 58 °C for 30 s and 72 °C for 30 s. The results normalized against GAPDH expression. Each qPCR was repeated at least three times for different experimental samples, and each reaction performed in triplicate. We used 2− (Ct control – Ct treatment) Reference- (Ct control – Ct treatment) target method. The sequence for forward and reverse primers of NS, GAPDH, and TP53 are shown in Table 1.
MTT cytotoxic assay
Briefly, various concentrations of siRNA solutions (i.e., 25, 50, 100, 200, and 400 nmol/μL) were spotted in each well of 96-well plates for transfection. In a sterile microtube, 1.5 μL of HiPerFect transfection reagent (Qiagen, USA) was mixed with 25 μL of RPMI1640 without FBS. Then, after 15 min of incubation at room temperature for liposome formation, the resulting mixture was added dropwise into each well. Finally, 5,000 cells/well in 175 μL of 10% FBS-contained RPMI1640 medium were added to each well and were allowed to adhere overnight. After 24, 48, and 72 h, 20 μL of MTT (3-(4,5-dimethylthiazol-2-yl)-2,5-diphenyltetrazolium bromide) dye (5 mg/mL) was added and incubated for 3.5 h. Afterward, 200 μL of dimethyl sulfoxide (DMSO) was added for solubilizing the formazan crystals. Following 15 min of incubation at room temperature, the absorbance was measured at 570 nm with a microplate reader. Also, cells treated with irrelevant siRNA were considered as controls.
Evaluation of the apoptosis
Apoptosis assays performed using annexin V- PI apoptosis Assay Kit (Thermo Fisher, USA). For this purpose, the number of viable and non-viable cells for each well was counted using the trypan blue test; then, 2 × 104 cells were seeded in each well of 6-well plates. After 48 h of treatment of cells with NS-siRNA (NS-targeting small interfering RNA) and irrelevant siRNA (IR-siRNA), the cells were washed in cold phosphate-buffered saline (PBS), and then gently trypsin solution (1%) was added to control transfected cells. Then, the trypsinized cells were collected and re-suspended in 200 μL of binding buffer and 5 μL of fluorochrome-conjugated annexin V (1 μg/mL). The mixture was incubated at room temperature for 15 min in the dark, and then 5 μL of PI (Propidium Iodide) was added. The cells were analyzed using a flow cytometer within 30 min of staining.
Statistical analysis
Statistical analysis was performed with SPSS version 16. An independent sample t-test and the Friedman test were used for comparison of mean ± SD values. The statistically significant level was considered P<0.05. All experiment was done at least in triplicates.
Results
Silencing of NS in breast cancer cells
First, we checked the effects of the NS-siRNAs on the expression of endogenous NS in MCF-7 human breast cancer cell lines, which had been transfected with 80 nmol/μL of NS-siRNA and the control dsRNA. At 6, 12, 24, 48, and 72 h after transfection, the expression of NS mRNA was assessed by real-time quantitative PCR. Compared with IR-siRNA, NS-siRNAs first caused a significant reduction in the expression level of the NS at 12 h after transfection (0.22 ± 0.05, p-Value<0.001) and following at 72 h after transfection, the knockdown of NS was observed. Also, the application of NS-siRNAs resulted in a significant reduction in NS expression in MDA-MB-468 cells compared to the cells treated with IR-siRNA and control (0.17 ± 0.06, p-value = 0.03) (Figure 1A). The optimal knockdown of NS in both MCF-7 and MDA-MB-468 cells was seen 72 h post-transfection (0.0001 ± 0.008, 0.0009 ± 0.009, respectively).
Decreased expression of TP53 mRNA in MDA-MB-468 compared to MCF-7 cells
To compare the expression of TP53 between the MCF-7 and MDA-MB-468 cells, mRNA levels of the TP53 was assessed in both MCF-7. Real-time PCR analysis indicated that MCF-7 cells express a comparable level of TP53 related to MDA-MB-468 cells, although the apoptosis induction of MDA-MB-468 cells is significantly less efficient than that of MCF-7 cells. (0.043 ± 0.03, p-Value<0.05). (Figure 1B)
Knockdown of NS inhibits MCF-7 and MDA-MB-468 cells proliferation
We next investigated the effects of knockdown of NS on the breast cancer cells. The four siRNAs targeting NS mRNA and IR-siRNA were respectively transfected into the MCF-7 cells at the concentrations of 25-50-100-200-400 nmol/μL. The effects of the NS-siRNAs at varying concentrations and times (24 to 72 h) on the proliferation and viability of MCF-7 and MDA-MB-468 cells were determined via MTT assay. Inhibition of cell growth and viability by NS-siRNAs was concentration and time-dependent (Figure 2). Reduction in MCF-7 cell viability via NS-siRNA treatment at increasing concentrations following 48 h ranged from 3 % to 79 %, whereas reduction of cell viability in MDA-MB-468 cells ranged from 7% to 88 % (Figure 2). Increasing concentrations resulted in a decrease in the percentage of viable cells. A similar cytotoxicity trend was also observed when the cells were treated with NS-siRNAs. The MCF-7 cells were more sensitive toward knockdown of NS than MDA-MD-468 cells (Figure 2). The IC50 values for the mixture of NS-siRNAs were 79.93 and 118.4 nmol/μL on MCF-7 and MDA-MB-468 cells, respectively.
Silencing of NS induces cell apoptosis in breast cancer cells
Then, we assessed the relationship between NS-siRNAs-mediated loss of apoptosis by annexin V/PI staining flow-cytometry analysis of MCF-7 and MDA-MB-468 cells labeled with PI and FITC. The NS-siRNAs and IR-siRNA were transfected into MCF-7 cells at 40, 80, and 160 nmol/μL concentrations and into MDA-MB-468 at 80, 160, and 240 nmol/μL concentrations. We found that the cells treated with concentrations higher than 80 nmol/μL of NS-siRNAs underwent significant apoptosis following 48 h treatment in both MCF-7 and MDA-MB-468 cells (p-value < 0.05). The number of early apoptotic cells increased to 4.37% in MCF-7 cells and 8.08% in MDA-MB-468 and the number of late apoptotic cells increased to 16.77% in MCF-7 cells and 13.02 % in MDA-MB-468. These data also showed that 52.73% of MCF-7 cells treated with NS-siRNAs and 51.62% of MDA-MB-468 cells treated with NS-siRNAs at 160 and 240 nmol/μL concentrations after 48 h were of late apoptotic cells (Figure 3, 4).
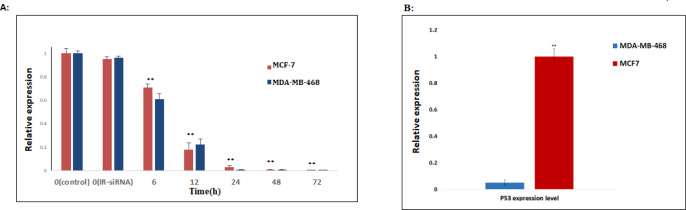
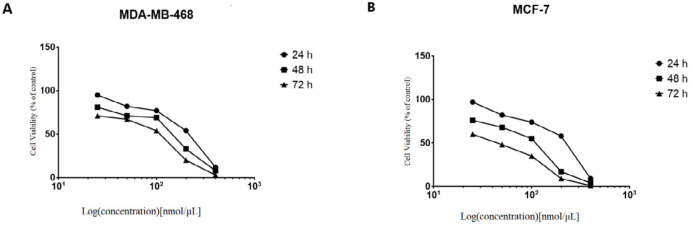
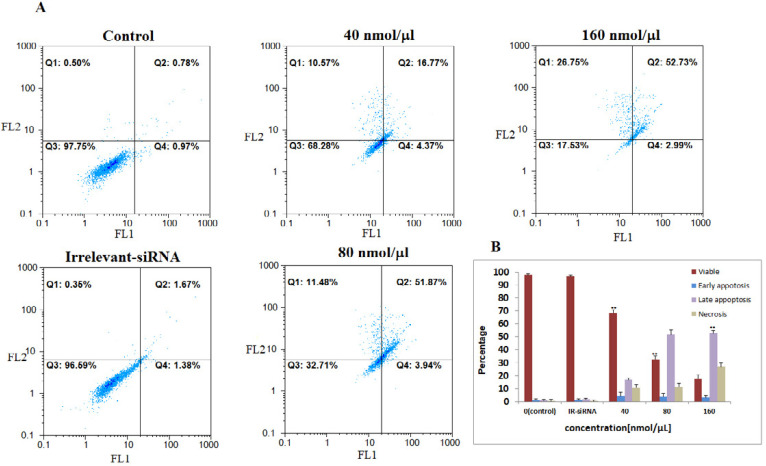
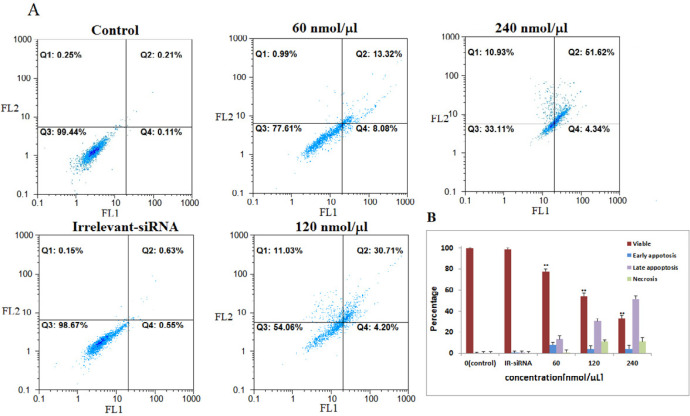
Primer sequences of TP53, NS and GAPDH
Genes | Sequence | Product length (bp) |
---|---|---|
TP53 (Forward) | CAGCACATGACGGAGGTTGT | 67 |
TP53 (Reverse) | CCAGACCATCGCTATCTGAGC | |
NS (Reverse) | AAAGCCATTCGGGTTGGAGT | 200 |
NS (Forward) | ACCACAGCAGTTTGGCAGCAC | |
GAPDH (Forward) | CATGTAGTTGAGGTCAATGAAGG | 150 |
GAPDH (Reverse) | GAGCCACATCGCTCAGACAC |
Discussion
This study revealed that NS depletion inhibits cell viability in MCF-7 and MDA-MB-468 human breast cancer cell lines at the concentrations of 25-400 nmol/μL; the decline in the viability of MCF-7 and MDA-MB-468 cell lines after knockdown of NS was entirely consistent with the literature. For instance, cell viability and cell growth decreased after NS knockdown in cervical cancer, bladder cancer, prostate cancer, and leukemia as well as many stem cells, such as human ESCs and hematopoietic stem cells (8, 10, 12, 13). Thus, these findings suggest that NS plays a critical regulatory role in the cell viability of cancers, especially MCF-7 and MDA-MB-468 cell lines.
Concerning previous studies, NS expression had increased in the MCF-7 cell line upon 17β Estradiol treatment and human breast cancer tissue (14). NS encodes a nucleolar GTP-binding protein abundantly expressed by cancers and stem cells, identified as a gene enriched in NSCs (11). Some studies revealed that NS is also needed for maintaining the proliferation of embryonic NSCs and human cancer cells and early embryogenesis (7). Therefore, the increased NS expression seems to be up-regulated in stem-like cells in tumor cells, better known as cancer stem cells or tumor-initiating cells (15). Also, our data determined that NS knockdown induces a late apoptotic response in MCF-7 and MDA-MB-468 cells. Consistent with our data, NS depletion after 48 and 72 h of NS-siRNAs transfection in K562 cells resulted in delayed apoptotic response (16). In contrast, early apoptosis response was also reported in PC-3 cells and HL-60 cells after knockdown of the NS gene (17). These discrepancies could be explained by differences in the knockdown levels of NS in different cell lines (higher than 80% in HL-60 and PC-3 cells) and differences in phenotype and proteomics of the cells.
In 2010, Tsai et al. reported that knockdown of NS reduced the sphere-forming activity of MDA-MB-231 and MCF-7 cells, and NS expression was associated with the basal subtype of mammary tumor cells. They also described that tumorigenic activities strongly increased in mammary tumor cells with higher expression levels of NS both in-vitro and in-vivo (15). Also, Antony et al, stated that knockdown of NS induced higher rates of apoptosis in Her2-transfected MCF-10A cells. Also, western blot analysis of their study indicated that MCF-7 cells express approximately 30% of the levels of NS compared to either Her2-transfected MCF-10A or SKBR3 cells. Therefore, it seems to have an essential role in the induction of apoptosis in human breast cell lines, which is consistent with our findings (18).
NS has been recently reported as a novel p53-binding protein (19) and is expressed in invasive breast cancer (20). Some investigations stated that NS is regarded as one of several nuclear proteins that can bind to MDM2 and therefore stabilize p53 and knockdown of NS induces cell cycle arrest/apoptosis in human cancer cells by up-regulation of p53 (19, 21). Some studies reported that NS depletion induced p53-independent apoptosis pathways in cancers. For example, Nikpour et al. demonstrated that NS depletion by siRNA induced a severe decline in cell proliferation and apoptosis in SW1710 cell line with mutated TP53 gene (22). Previous investigations revealed that the MDA-MB-468 cell line harbors a bi-allelic mutation in TP53, which did not affect its binding affinity to BRCA1 (23). In another paper, it is reported that the inhibition of TP53 mutation leads to apoptosis induction. Thus TP53 mutation is required for the survival of MDA-MB-468 cells (24). However, the MCF7 cells are TP53-proficient. Also, it is estimated that one-third of breast cancer cases are associated with TP53 mutations (25). The genomic instability that was observed in MDA-MB-468 cells mainly activates ATM (Ataxia Telangiectasia Mutated) and related proteins. ATM activation with the stabilization of p53 and BRCA1 results in up-regulation of the mutated p53 and BRCA1 in these cells (26, 27). In this paper, we evaluated the possible correlation between siRNA-induced NS depletion and the expression level of TP53 in MCF-7 cells (without mutated TP53) and MDA-MB-468 cells (harboring mutated TP53). The TP53 mRNA levels were assessed in MCF-7 compared to MDA-MB-468 cell lines, showing that although TP53 expression was significantly decreased in the MDA-MB-468 cells compared to MCF-7 cells prior to treatment with a mixture of NS-siRNAs, knockdown of NS induced apoptosis in both cell lines. Thus, in concordance with Nikpour et al. findings, our data demonstrated that NS may activate p53-independent apoptotic pathways in MDA-MB-468 cell lines lacking TP53 expression. Yet, the molecular mechanism of apoptosis induction via NS silencing and the link between NS and TP53 status in human breast cancer cells remained ill-defined. Therefore, the identification of these associations requires simultaneous TP53 silencing and knockdown of NS in both MCF-7 and MDA-MB-468 cells.
Conclusion
In overall, our results suggest a strong correlation between the NS signalling pathway and cancer cell survival. These findings provide evidence that knockdown of NS can induce apoptosis in MCF7 and MDA-MB-468 breast tumor cells. Thus, in line with original reports, NS may have a novel functional role in cell cycle regulation.
Acknowledgements
References
-
1.
Ghoncheh M, Pournamdar Z, Salehiniya H. Incidence and Mortality and Epidemiology of Breast Cancer in the World. Asian Pac. J. Cancer Prev. 2016;17:43-6.
-
2.
Dai X, Li T, Bai Z, Yang Y, Liu X, Zhan J, Shi B. Breast cancer intrinsic subtype classification, clinical use and future trends. Am. J. Cancer Res. 2015;5:2929-43. [PubMed ID: 26693050].
-
3.
Duffy MJ, Harbeck N, Nap M, Molina R, Nicolini A, Senkus E, Cardoso F. Clinical use of biomarkers in breast cancer: Updated guidelines from the European Group on Tumor Markers (EGTM). Eur. J. Cancer. 2017;75:284-98. [PubMed ID: 28259011].
-
4.
Chan M, Chang MC, González R, Lategan B, del Barco E, Vera-Badillo F, Quesada P, Goldstein R, Cruz I, Ocana A, Cruz JJ, Amir E. Outcomes of Estrogen Receptor Negative and Progesterone Receptor Positive Breast Cancer. PLOS ONE. 2015;10:e0132449. [PubMed ID: 26161666].
-
5.
Holliday DL, Speirs V. Choosing the right cell line for breast cancer research. Breast Cancer Res. BCR. 2011;13:215. [PubMed ID: 21884641].
-
6.
Qu J, Bishop JM. NS maintains self-renewal of embryonic stem cells and promotes reprogramming of somatic cells to pluripotency. J. Cell Biol. 2012;197:731-45. [PubMed ID: 22689653].
-
7.
Tsai RYL, McKay RDG. A nucleolar mechanism controlling cell proliferation in stem cells and cancer cells. Genes. Dev. 2002;16:2991-3003. [PubMed ID: 12464630].
-
8.
Nikpour P, Mowla SJ, Jafarnejad SM, Fischer U, Schulz WA. Differential effects of NS suppression on cell cycle arrest and apoptosis in the bladder cancer cell lines 5637 and SW1710. Cell Prolif. 2009;42:762-9. [PubMed ID: 19706044].
-
9.
Beekman C, Nichane M, De Clercq S, Maetens M, Floss T, Wurst W, Bellefroid E, Marine J-C. Evolutionarily conserved role of NS: controlling proliferation of stem/progenitor cells during early vertebrate development. Mol. Cell Biol. 2006;26:9291-301. [PubMed ID: 17000755].
-
10.
Moosavi MA, SEYED GN, ASVADI KI, Asadi M. NS gene silencing by siRNA and growth inhibition, cell cycle arrest and apoptosis induction of K562 leukemia cell line. SID.ir. 2011;14:104-16.
-
11.
Karsten SL, Kudo LC, Jackson R, Sabatti C, Kornblum HI, Geschwind DH. Global analysis of gene expression in neural progenitors reveals specific cell-cycle, signaling, and metabolic networks. Dev. Biol. 2003;261:165-82. [PubMed ID: 12941627].
-
12.
Wang J, Wang L, Ji Q, Zhu H, Han S. Knockdown of NS in an ovarian cancer SKOV-3 cell line and its effects on cell malignancy. Biochem. Bioph. Res. Co. 2017;487:262-7.
-
13.
Yoshida R, Nakayama H, Nagata M, Hirosue A, Tanaka T, Kawahara K, Nakagawa Y, Matsuoka Y, Sakata J, Arita H. Overexpression of NS contributes to an advanced malignant phenotype and a poor prognosis in oral squamous cell carcinoma. Br. J. Cancer. 2014;111:2308.
-
14.
Kobayashi T, Masutomi K, Tamura K, Moriya T, Yamasaki T, Fujiwara Y, Takahashi S, Yamamoto J, Tsuda H. NS expression in invasive breast cancer. BMC Cancer. 2014;14:215. [PubMed ID: 24650343].
-
15.
Lin T, Meng L, Li Y, Tsai RY. Tumor-initiating function of NS-enriched mammary tumor cells. Cancer Res. 2010;70:9444-52. [PubMed ID: 21045149].
-
16.
Gogani N, Rahmati M, Zarghami N, Asvadi-Kermani I, Hoseinpour-Feyzi MA, Moosavi MA. NS depletion induces post-G1 arrest apoptosis in chronic myelogenous leukemia K562 cells. Adv. Pharm .Bull. 2014;4:55-60. [PubMed ID: 24409410].
-
17.
Singh SK, Banerjee S, Acosta EP, Lillard JW, Singh R. Resveratrol induces cell cycle arrest and apoptosis with docetaxel in prostate cancer cells via a P53 / p21WAF1/CIP1 and p27KIP1 pathway. Oncotarget. 2017;8:17216-28. [PubMed ID: 28212547].
-
18.
Tin AS, Park AH, Sundar SN, Firestone GL. Essential role of the cancer stem/progenitor cell marker NS for indole-3-carbinol anti-proliferative responsiveness in human breast cancer cells. BMC. biology. 2014;12:72. [PubMed ID: 25209720].
-
19.
Dai M-S, Sun X-X, Lu H. Aberrant expression of NS activates P53 and induces cell cycle arrest via inhibition of MDM2. Mol. Cell Biol. 2008;28:4365-76. [PubMed ID: 18426907].
-
20.
Kobayashi T, Masutomi K, Tamura K, Moriya T, Yamasaki T, Fujiwara Y, Takahashi S, Yamamoto J, Tsuda H. NS expression in invasive breast cancer. BMC Cancer. 2014;14:215. [PubMed ID: 24650343].
-
21.
Meng L, Lin T, Tsai RY. Nucleoplasmic mobilization of NS stabilizes MDM2 and promotes G2-M progression and cell survival. J. Cell Sci. 2008;121:4037-46. [PubMed ID: 19033382].
-
22.
Nikpour P, Mowla SJ, Jafarnejad SM, Fischer U, Schulz WA. Differential effects of NS suppression on cell cycle arrest and apoptosis in the bladder cancer cell lines 5637 and SW1710. Cell Prolif. 2009;42:762-9. [PubMed ID: 19706044].
-
23.
Bossi G, Lapi E, Strano S, Rinaldo C, Blandino G, Sacchi A. Mutant P53 gain of function: reduction of tumor malignancy of human cancer cell lines through abrogation of mutant P53expression. Oncogene. 2006;25:304-9. [PubMed ID: 16170357].
-
24.
Lim LY, Vidnovic N, Ellisen LW, Leong CO. Mutant P53 mediates survival of breast cancer cells. Br. J. Cancer. 2009;101:1606-12. [PubMed ID: 19773755].
-
25.
Leroy B, Girard L, Hollestelle A, Minna JD, Gazdar AF, Soussi T. Analysis of TP53 mutation status in human cancer cell lines: a reassessment. Hum Mutat. 2014;35:756-65. [PubMed ID: 24700732].
-
26.
Tsai RYL. Turning a new page on NS and self-renewal. J. Cell Sci. 2014;127:3885-91. [PubMed ID: 25128565].
-
27.
Rasti M, Azimi T. TP53 Binding to BRCA1 and RAD51 in MCF7 and MDA-MB-468 Breast Cancer Cell Lines In-vivo and In-vitro. Avicenna J. Med. Biotechnol. 2015;7:76-9. [PubMed ID: 26140185].