Abstract
Keywords
Diabetes mellitus Levisticum officinale Antioxidants Glucose transporters Phytochemicals Molecular docking
Introduction
Diabetes mellitus (DM) is a serious prevalent metabolic disorder and a public health problem affecting a large proportion of population. It is well characterized by hyperglycemia, and dyslipidemia. Hypergly-cemia induces oxidative stress and leads to progression of DM-associated damages. Hence, blood glucose control is the main policy for DM management. Starch and the other carbohydrates of diet are hydrolyzed by pancreatic α- amylase (αA) and intestinal α- glucosidas enzymes and then resultant monosaccharides leave these cells toward the bloodstream through apical sodium- glucose transporter 1 (SGLT1) and basal facilitated glucose transporter 2 (GLUT2). Furthermore, lots of filtered glucose in the kidney’s glumeruli is reabsorbed via SGLT2 and GLUT2 transporters at luminal and basal epithelial membranes of proximal tubules, respectively. It seems that αA inhibition and glucose reuptake restriction are effective strategies for lowering blood glucose rise after meal. Despite availability of different classes of synthetic antidiabetic drugs, research in this field has continued because of undesired side effects of these known agents. Plants as a major natural source of antioxidants, have been a favorite source of drugs with fewer adverse side effects but a lot of health benefits. Medicinal plants have been used for DM remedy from a long times ago. Levisticum officinale Koch (Apiaceae), or lovage, is locally called karafs-e koohi in Iran, grows in a humid valley beside the Ordigan waterfall at 3200-3500 m in elevation on Hezar mountain in Kerman province. This plant is a cultivated or a semi-native plant in Europe and North America (1). L. officinale is a perennial and aromatic plant with a celery-like flavour and smell. Different parts of L. officinale have been used for many medicinal purposes. Its root has been used as carminative, spasmolytic (2), and diuretic (3). The antimicrobial and antioxidant activity of the hexane extracts from the roots and seeds of L. officinale have been determined (4) and it was revealed that a methanol extract of L. officinale root showed acetyl cholinesterase and pancreatic lipase inhibitory activity (5,6). Also, the methanolic and aqueous extract of L. officinale root showed a strong inhibitory effect on α-glucosidase (7). Furthermore, the ethanol extract from the fruit of L. officinale induced apoptosis in leukemia cell lines (8) and ethanolic extract of aerial parts exerted antiproliferative effect against breast cancer cell lines (9). In the other hand, it is reported that aerial parts essencial oil of L. officinale has antioxidant (10) antibacterial (11) and antifungal (12) properties. Moreover, ethanolic extract of its leaves, stem and root had radical scavenging activity (13). The stem and leaves of this plant are used as spice for milk products in Iran and despite local people acclaim on strong antidyslipidemic property of it, this has not been scientifically documented so far. Since, no study has been designed thus far to investigate the effect of hydroalcoholic extract of the stems and leaves of L. officinale (LOE) on various aspects of diabetic complications including dyslipidemia, the objective of this study was conducted to determine this and the related probable mechanisms and provide more valuable informations about bioactive constituents of LOE. Also, recent study evaluated and predicted αA enzyme inhibitory activity of extract ingredients by in silico analysis.
Custom
Preparation of extract
Fresh stems and leaves of L. officinale were collected during May and June from Hezar mountain in Kerman province in southeastern Iran. The plant was authenticated by a plant taxonomist, Dr. S.M. Mirtadzadini. A specimen was kept in MIR herbarium (voucher no. 2046) of Biology Department at Shahid Bahonar University of Kerman. The plant parts were dried at room temperature, ground to powder and extracted with 80% methanol by maceration. For this, powdered plant materials were allowed to soak in a closed container with solvent at room temperature for at least 3 days with occasional shaking. Then, the liquid part strained off and separated by filtration and was dried in 40 °C oven and stored at 4 °C for use (14). The final extraction yield was about 22%.
Animals and Experimental design
Male Wistar rats weighing 200–250 g were housed in the animal room of the Department of Biology in cages and maintained under environmentally controlled conditions (23 ± 2 °C; 12/12 h light/dark cycle; 55% humidity). They were provided with free food and tap water access. The study procedure was approved by the Institutional Animal Ethics Committee of Shahid Bahonar University of Kerman (approval number: 1394). Diabetes was induced in 30 rats by intraperitoneal injection of 65 mg/kg, body wt. of streptozotocin (STZ) dissolved in citrate buffer (0.1 M; pH = 4.5). Hyperglycemia developed about five day STZ post-injection. The rats were fasted for 12 h and blood obtained from the tail vein for glucose level estimation by glucometer (Accu-check; Roche Diagnostics; Germany). The animals with fasting blood glucose levels above 300 mg/dl were considered to be diabetic. The diabetic rats were randomly divided into five groups (n = 6) and received distilled water, different doses of LOE (100, 300 and 500 mg/kg body wt.) and glibenclamide (20 mg/kg body wt.) by gavage for 14 days. One group of normal rats also treated with distilled water for 14 days. At the end, the fasted rats were anesthetized by inhalation of CO2 and killed by decapitation (15, 16). The blood samples were immediately collected and centrifuged for 10 min at 3000 g to obtain serum and plasma samples, which were then stored at -20 °C for analysis of biochemical parameters. Pancreas, liver, kidney, and jejunum samples of each group were excised and frozen at -70 °C. Serum glucose, triglycerides, total cholesterol, HDL-C, creatinine, aspartate aminotransferase (AST), and alanine aminotransferase (ALT) levels were measured using standard kits (Pars Azmoon; Iran) by spectrophotometer (CS-T240; ADVANCE; China) and LDL levels were calculated according to this equation: LDL = Total cholesterol - [HDL + (Triglycerides/5)]. Serum insulin levels were determined using rat enzyme immunoassay kits (Mercodia; Uppsala; Sweden).
Oral glucose tolerance test (OGTT)
Another 30 fasted normal male rats were divided into five groups (n = 6) and received distilled water, LOE (100, 300, and 500 mg/kg body wt.) and glibenclamide (20 mg/kg body wt.) individually by gavage 30 min before glucose loading (5 g/kg body wt.). The blood samples were collected from the tail vein before treatment and glucose loading as well as at 30, 60, 90, 120, and 240 min post-treatment and glucose serum levels determined (17).
Antioxidant enzyme activity and lipid peroxidation measurement
The pancreas and liver tissues were homogenized and centrifuged and the supernatants were used for antioxidant assessment. Catalase (CAT) activity was measured as described by Aebi (18). Superoxide dismutase (SOD) activity was characterized by the method recommended by Giannopolitis and Ries (19). The tissue protein levels were determined as described by Bradford (20). The malondialdehyde (MDA) levels in these tissues were measured as recommended by Ohkawa et al. (21) and modified by Jamall and Smith (22). The plasma MDA level was determined as described by Kurtel et al. (23).
Determination of gene expression level by relative quantitative real-time RT-PCR
Total RNA extraction from the jejunum and cortical portions of kidney tissue samples from different groups was done using a Total RNA Purification Kit (GeneAll; Korea). The purity and concentration of extracted RNA was determined by spectrophotometer (Nanodrop 2000c; Thermo Fisher Scientific; USA). The integrity of the RNA samples containing GelRed was detected on 1.5% agarose gel. A specific amount of total RNA samples were used for cDNA synthesis through RevertAid First Strand cDNA Synthesis kit (Thermo Fisher Scientific; UK) according to the manufacturer instructions. The primer sequences for desired genes were according to Honari et al. design (16).and included SGLT1 (Forward: 5ʼ- CCTGTACCGTCTGTGC-3ʼ; Reverse: 5ʼ- ACAGAACAGGTCATATGCCTT-3ʼ), SGLT2 (Forward: 5ʼ- CCAATAGAGGCACAGTTGGTGG-3ʼ; Reverse: 5ʼ- GTAAATGTTCCACAACGG-3ʼ) and GLUT2 (Forward: 5ʼ- TACATTGGCGAGATTGCT-3ʼ; Reverse: 5ʼ- TGATTGCCCAGAATGAAG-3ʼ) and β-actin as an internal control (Forward: 5ʼ- GCTGAGAGGGAAATCGTGC-3ʼ; Reverse: 5ʼ-TCAACGTCACACTTCATGATGG-3ʼ). Also, the products sizes of these were 111, 186, 122 and 253 bps, respectively. To determine the sodium-glucose cotransporter 1 and 2 (SGLT1, SGLT2) and facilitated glucose transporter 2 (GLUT2) mRNA levels, relative quantitative real-time PCR was done with a multicolor real-time PCR detection system (iQ5; Bio-Rad; USA). The relative amount of mRNA was calculated by the Livak method (24) as: fold change or relative Expression = 2-ΔΔCt.
Evaluation of pancreatic α-amylase inhibition potency of LOE
To evaluate the inhibitory effect of extract on pancreatic α-amylase activity, Porcine pancreatic α-amylase enzyme solution (ppA; 0.5 U/mL) and various concentrations of LOE (0.1, 0.5, 1, and 10 mg/mL in DMSO) were mixed and incubated at 25 °C for 30 min. Next, a starch solution (0.5%) was added and incubated at 37 °C for 15 min. At the end, color reagent solution included DNSA (96 mM) was added and placed into a water bath at 85 °C for 15 min. The reaction mixture absorbance value was read at 540 nm against associated blank after dilution with distilled water (25). The control tube (without extract) was considered to be 100% enzyme activity. Acarbose (Exir; Iran) (0.5, 1, 2, 5, 10 mg/mL), the known ppA inhibitor, was used as the positive control. The inhibition percentage of α-amylase determined according to the following formula:
I α- amylase (%) = 100 × [ΔAControl – ΔASample] / ΔAControl
Phytochemistry analysis of extract
Preliminary phytochemical screening of LOE was carried out by the usual chemical tests for characterization of different plant metabolites (26). The method described by Singleton et al. (27) was used to measure the total phenolic compound content in the LOE with some modifications. Data were reported as milligrams of Gallic acid equivalent per gram of dry weight of extract (GAE/g). The method designed by Kumazawa et al. was used to optimize and as a guideline for this assay. In this method, the quantification of flavonoids is based on its complex product with aluminum chloride solution (10% w/v) and the spectrophotometric determination of the formed complex. Data were recalculated as milligrams of catechin equivalent per gram of dry weight of LOE (28).
HPLC system was applied to determine the amount of some of the flavonoids and phenolic acids in the LOE. For this, the chromatographic system conditions were set at room temperature (25 °C), quercetin (Q) ≥ 95.0%, luteolin > 98%, chlorogenic acid (CGA) ≥ 95.0%, rosmarinic acid (RA) > 98% and caffeic acid (CA) > 98% were used as standards and UV detector (K2501) adjusted at 330 nm. Also, mobile phases including A: 15% methanol (adjust to pH 2 with phosphoric acid) and B: methanol were used for CGA and CA, mobile phases including A: 0.3 g/L solution of phosphoric acid (adjust to pH 2) and B: methanol for Q and luteolin and mobile phases including A: phosphoric acid/acetonitrile/water (1:19:80 v/v/v) and B: phosphoric acid/methanol/acetonitrile (1:40:59 v/v/v) for RA were applied. The flow rate was 1.2 mL/min for RA and 1 mL/min for the others and reversed-phase C18 column (5.0 μm, 250 mm in length, 4.6 mm inner diameter) was used. Crude extract was dissolved in methanol 80%, passed through 0.45 μm filter and injected to HPLC system. The injection volume was 20 µL. Chromgate software used for data acquisition.
Also, above filtered LOE was subjected to gas chromatography and mass spectroscopy (GC-MS) analysis for phytochemical screening of bioactive volatile and semi-volatile compounds. This was carried out using Agilent 6890 series equipped with 5973 Mass Selective Detector, HP-1 capillary column of dimensions 30.0 m × 250 μm × 0.5 μm and helium (99/9%) as carrier gas with a flow rate of 1 mL/min. The oven temperature was set initially at 50 ºC for 2 min, followed by an increase of 8 ºC /min to 250 ºC and was kept isothermally for 30 min. The split sampling technique was used to inject 1 µl of sample in the ratio of 1:5. The quadrapole MS was operated at 70 eV and MS spectra range was 40-500 m/z. LOE constituents were identified and interpreted by comparison of their mass spectral data including gas chromatogram retention times with those from NIST and Wiley libraries.
Molecular docking (In silico studies)
Docking studies through Virtual screening technique were designed with some of known extract constituents (as ligands) with human pancreatic α- amylase enzyme (as receptor) in order to determine or confirm possible role of known extract ingredients in α- amylase enzyme inhibitory potency of extract. Virtual screening is a computational alternative method in the drug discovery field and has had a great success in this pharmaceutical field (29, 30). For this, human pancreatic α- amylase enzyme crystal structure (PDB ID: 5U3A) was retrieved from the RCSB protein data bank (PDB) and this 3D structure was prepared and optimized by removing water and other excess molecules or atoms before docking and the outputs were saved for docking process. Moreover, the 3D structure of the known desired extract ingredients, including chlorogenic acid, rosmarinic acid, caffeic acid, quercetin, luteolin, phthalides, thymol, phytol, hexanoic acid, carene, and menthofuran were downloaded from Zinc 15 database and designed in the SDF format. Conformational analysis and geometry optimization and preparation of both receptor (α-amylase enzyme) and ligands (above known extract compounds) were done. Schrodinger 2018-4 software package was used for all steps of this docking study. With respect to docking outputs, a more negative docking score means a more and stronger interaction possibility between associated ligand and α- amylase enzyme. Docking output results were visualized using LigPlot+.
Evaluation of LOE toxicity
Healthy Wistar rats were fasted for 12 h, then treated with an oral dose of LOE (5000 mg/kg body wt.). The animals were followed up for behavioral and neurological signs of toxicity (fatigue, paw licking, watery stool, writhing, and loss of appetite) and mortality for up to 72 h. Also, the blood metabolic parameters were monitored at the end of this period.
Statistical analysis
The results were reported as mean ± SEM. One way analysis of variance (ANOVA) and Tukey post test were applied for statistical analysis. The differences were accepted significant at p < 0.05.
Results and Discussion
In this study, an acute toxicity test with a pharmacological procedure was used for the determination of LD50 and the selection of safe dosage range of LOE used in this study. Results showed no mortality or sign of toxicity at 5000 mg/kg was caused by acute administration of LOE after 72 h. Hence, the LD50 of LOE is above this value and the recent applied doses of LOE (100, 300 and 500 mg/kg) can be considered safe.
It is well established that plant extracts possess different bioactive substances which lead to their different properties, so, here, we performed phytochemical analysis of LOE in order to determine what the extract contains. Qualitative phytochemical screening of LOE showed the presence of flavonoids, phenols, tannins, glycosides, steroids, and phytosterols. Also, according to quantitative approaches, the phenol and flavonoid content in the LOE were 69.78 ± 9.3 and 36.17 ± 4.19 mg/g of extract dry weight, respectively, based on the Gallic acid and catechin concentration/absorption curves. In addition, HPLC analysis of LOE revealed the presence of 188.73 ± 2 mg/g CGA, 0.26 ± 0.01 mg/g rosmarinic acid, 0.028 ± 0.003 mg/g caffeic acid as phenolic acids and 0.36 ± 0.05 mg/g quercetin and 0.21 ± 0.005 mg/g luteoin as flavonoids (Figure 1 A-H). Moreover, in the GC-MS analysis, the mass spectra of identified chemical compounds from LOE were matched with those found in the NIST/Wiley libraries are listed in Table 1 and the associated chromatogram is depicted in Figure 1, M. However, in this, the peaks at 15.53 min and 18.39 min are related to the solvents. Results of this analysis of LOE revealed the presence of phytochemical compounds like phthalides, thymol, phytol, hexanoic acid, carene and menthofuran which include important medicinal properties.
Regarding the literature, phytochemical analysis of essential oils of different parts of L. officinale determined the presence of monoterpenic hydrocarbons as β-phellandrene and α-Terpinyl acetate (31), Curzerene γ-Cadinene, Sabinene (10), 6-butyl-cyclohepta-1,4-diene and 7-formyl-4-methyl-cumarine (12), pentyl cyclohexa-1,3-diene, Z-ligustilide, neocnidilide, Z-β-ocimene, p-menth-1-en-8-ol acetate and pentyl cyclohexa-1,3-diene (11, 31). Besides, Tomsone et al. reported that there are the highest content of phenolic and flavonoid compounds in root, seeds and especially in lovage leaves ethanolic extract and confirm partly some of our above results (13). Thereby, even though there are informations about the composition of L. officinale essential oil, this was the first study to describe and partially quantify phytochemical components of hydroalcoholic extract of stems and leaves of lovage (LOE) with above valid methods.
In DM, disturbances in body metabolic regulatory mechanisms caused by insulin deficiency or insulin resistance led to dyslipidemia due to recruitment of free fatty acids from peripheral fat stores. This increases the production of triglyceride-rich lipoproteins in the liver were accompanied by a decrease in HDL-C (32). An increase in serum ALT and AST levels is mainly caused by leakage of these enzymes from liver cells into the blood stream and indicates the loss of hepatocyte integrity in DM (33). In the present study, anti-diabetic properties of different doses of LOE (100, 300 and 500 mg/kg) were determined. Results showed that LOE (500 mg/kg) administration, for 14 days significantly decreased serum glucose level (24.97%) and increased insulin serum level compared to the diabetic rats. Also, LOE treatment (300 and 500 mg/kg) significantly decreased the level of serum cholesterol, triglycerides, LDL, creatinine, ALT, AST and increased the HDL levels. Moreover, LOE (100 mg/kg) caused a decrease in the total cholesterol, LDL and creatinine serum levels compared with the control diabetic rats (Table 2).
Moreover, in the current experiment, administration of LOE (100, 300 and 500 mg/kg) and glibenclamide as a reference drug (20 mg/kg) on the glucose tolerance in normal rats are shown in Table 3. At 90 min after glucose loading or 120 min after LOE administration (500 mg/kg), the blood glucose level had decreased significantly by 13% when compared with the control rats.
These results can be attributed to above extract ingredients because, it has been shown that CGA exerts hypoglycemic and hypolipidemic effects (34) and it is able to modulate glucose uptake and stimulate insulin secretion from rat Langerhans islets (35). Moreover, rosmarinic acid (RA) has a significant hypoglycemic effect and insulin secreting activity in diabetic rats (36). Also, quercetin is able to regenerate the pancreatic islets and induce insulin release. Additionally a significant decrease in glucose, cholesterol, triglycerides, and glucose absorption levels have been observed in quercetin-treated diabetic rats (37). Furthermore, thymol and phytol have antihyperglycemic and antihyperlipidemic properties (38, 39) and hexanoic or caproic acid improves insulin stimulated glucose uptake (40).
It is known that glucose uptake increases in DM. SGLT1 in the apical membrane and GLUT2 in the basal membrane of enterocytes in the small intestine are mainly involved in the glucose absorption after carbohydrate digestion. Moreover, glucose transporters in the proximal tubule of kidney (SGLT2 and GLUT2) are critical to glucose reabsorption. In uncontrolled diabetes, adaptive changes caused by hyperglycemia increase the flux of transepithelial glucose through renal (41) and intestinal glucose transporters (42).
Here the effect of LOE (500 mg/kg) on glucose transporter gene expression in the jejunum and kidney tissue of diabetic rats were investigated in order to find out the antihyperglycemic mechanism of extract more, Results showed that renal SGLT2 and GLUT2 (Figure 2 A, B) as well as jejunum SGLT1 and GLUT2 gene expression levels (p < 0.05) (Figure 2 C, D) increased significantly in the diabetic rats compared to the control groups and LOE (500 mg/kg) administration significantly decreased the renal SGLT2 (p < 0.01), GLUT2 (p < 0.001), and jejunum GLUT2 mRNA levels (p < 0.001) (Figure 2).
It has been reported that CGA maintains glucose homeostasis by modulating the expression of SGLT-1 and GLUT2 in the intestinal segments of rats fed with a high-fat diet (43).
Moreover, a decrease in glucose absorption by caffeic acid (CA) through the inhibition of intestinal SGLT1 and GLUT2 has been reported (44). Moreover, quercetin acts as an antidiabetic agent through glucose absorption reduction in quercetin-treated diabetic rats (37). It has been reported that inhibition of glucose absorption by quercetin can occur through competitive inhibition of SGLTs or by noncompetitive inhibition of intestinal transporter GLUT2 (45).
One of the other therapeutic strategies to decrease the blood glucose levels in DM is inhibition of the oligo and disaccharides breakdown to absorbable monosacarides. This could be done by inhibition of the carbohydrate-hydrolysing enzymes like α-amylase (Αα). Pancreatic α-amylase enzyme catalyzes carbohydrate breakdown to oligosaccharides and disaccharides in the intestine. It is previously justified that α-amylase inhibitors can improve hyperglycemia in diabetic conditions (46). It is well documented that plant polyphenoles are inhibitors of this enzyme which can reduce glucose absorption and blunt the plasma glucose rise (47, 48).
Recent findings showed that LOE has 14% ± 2% α-amylase inhibitory activity at a concentration of 10 mg/ml compared to the inhibitory effect of acarbose (94% ± 3%) as a reference drug. This result can be attributed to the presence of phytochemical compounds especially phenolic compounds in the extract with well known α-amylase inhibitory effect as previous findings reports that RA (49), luteolin and quercetin (47) have pancreatic α-amylase inhibitory effect. Also, Komaki et al. reported that luteolin glucosides have anti- human pancreatic α-amylase property (50). Finally, our recent in silico study investigated the probable α- amylase enzyme inhibitory activity of 11 known phytochemical compounds of LOE to predict possible antihyperglycemic potency of them. Regarding the resultant docking scores (Table 4), among LOE docked compounds, luteolin, quercetin, rosmarinic, caffeic, and hexanoic acids had the greatest interaction probability with α- amylase enzyme then, thymol, carene, phthalides, and phytol showed enzyme interactions more weakly, respectively. Also, chlorogenic acid and menthofuran as the other LOE ingredients show no interaction through a molecular docking simulation (Table 4).
As shown in this table, molecular docking output revealed that quercetin, rosmarinic, and hexanoic acids have a similar interaction core with αA enzyme. Also, luteolin and caffeic acid are the same in interaction loci with this enzyme. The α- amylase enzyme is a single-chain protein which has three structural domains and amino acids residues 1-99 and 69-404 in domain A houses its active site. The active site of it includes catalytic residues: Asp197, Glu233, and Asp300 (51). Intermolecular interactions obtained from docking algorithms structural analysis were presented in Figure 3.
Regarding this, Asp197 and Glu233 at catalytic active site of αA are critical amino acid residues which get involved in at least five of the ligands (luteolin, quercetin, rosmarinic, caffeic and hexanoic acids) which have the strongest interaction with αA. Then, Arg346 in thymol and phthalides and His305 in quercetin and phytol also, can consider as important interacting residues.
In line with our molecular docking results, Rasouli et al. demonstrated possible α- amylase enzyme inhibitory activity of caffeic acid, luteolin and quercetin by virtual screening method and described that the αA inhibitory activity of luteolin was stronger than luteolin glucoside (52). Thus, according to these in silico results, we can confirm antihyperglycemic property of luteolin, quercetin, rosmarinic acid, and suggest caffeic and hexanoic acids, thymol, carene, phthalides, and phytol as potential eligible lead components to inhibit α- amylase enzyme and predict an effective antihyperglycemic potential for them. However, complementary experimental studies needed to verify this. In addition, molecular dynamic results from recent work can be helpful for future studies about drug design against DM.
There are a lot of reports include interactions between diabetes and oxidative stress. A decrease in SOD and CAT activity in the liver and pancreas of diabetic rats has been reported (53). SOD and CAT enzymes are major components of the antioxidant defense system of the body. Decreased activity in antioxidant enzymes increases accumulation of free radicals, which in turn leads to lipid peroxidation. This is determined by the elevation of MDA content. MDA can damage body organs such as the liver and pancreas (54).
In this study, the enzymatic SOD and CAT activities in the pancreas and liver tissues decreased significantly (p < 0.05) in the diabetic rats and LOE administration (500 mg/kg) increased these tissue enzymes activities in the diabetic treated rats in comparison with the untreated ones (p < 0.01; Table 5). Diabetic animals exhibited a significant increase in the plasma, pancreas and liver tissues MDA levels (p < 0.001) and LOE (500 mg/kg) significantly decreased the MDA levels over that of the controls (p < 0.001; Table 5).
As mentioned above, there are many polyphenolic compounds with antioxidant activity in the extract which mediate these properties. Kono et al. (55) had shown that chlorogenic acid and caffeic acids exert antioxidant property. Also, luteolin as another flavonoid in LOE possess antioxidant and anti-inflammatory properties (56) and menthofuran is an antioxidant with radical scavenging activity (57). Rosmarinic acid (RA) is a phenolic acid known as a potent antioxidant (58).
Thus, these substances are closely associated to antioxidant effect of LOE.

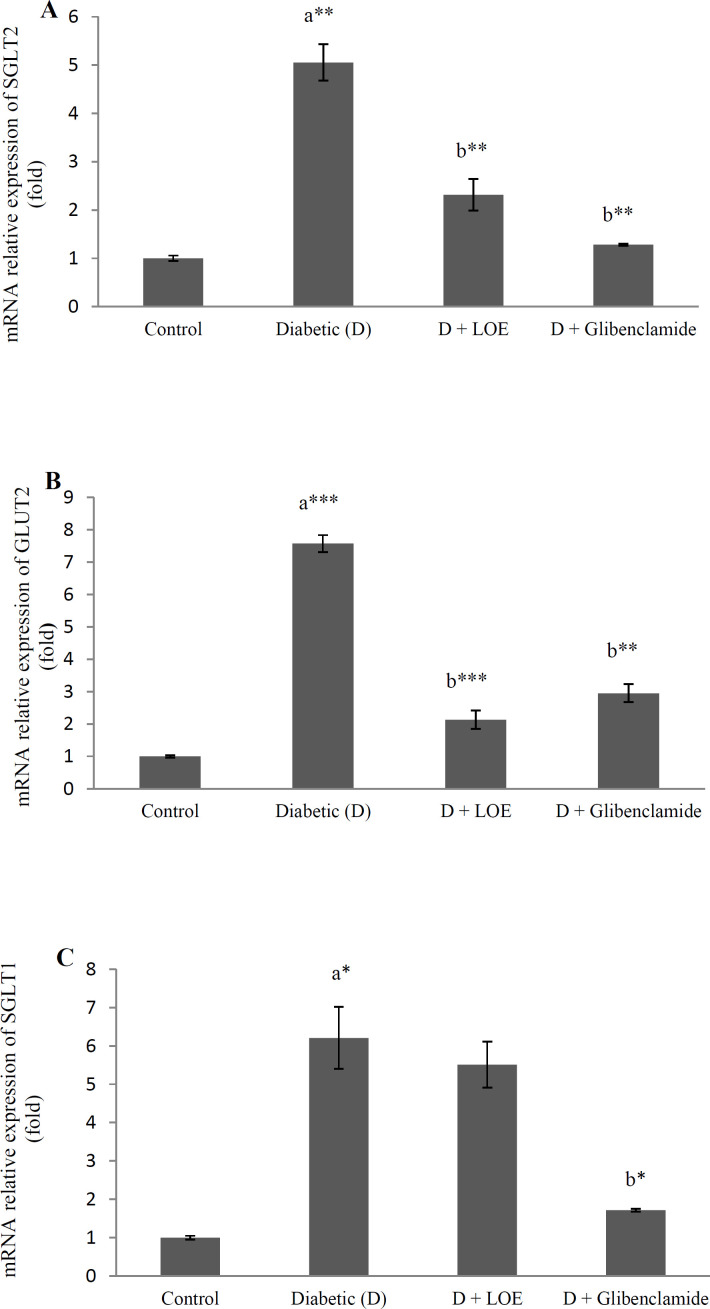
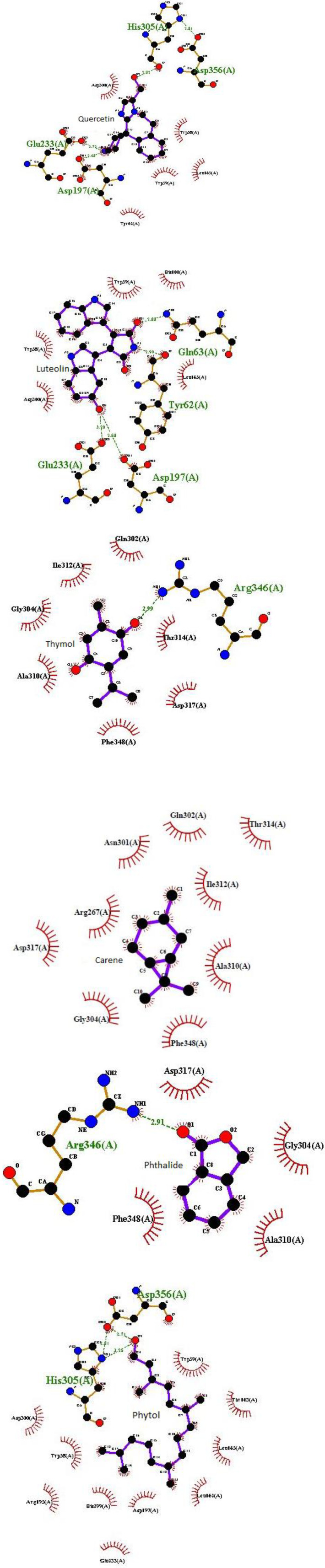
Identified LOE chemical constituents from a GC-MS analysis
NO. | Retention Time (min) | Compounds | Peak area (%) |
---|---|---|---|
1 | 11.843 | Hexanoic acid | 29.81 |
2 | 14.667 | Thymol | 1.69 |
3 | 15.434 | Carene | 2.79 |
4 | 19.395 | Isobutylidenphthalide | 1.27 |
5 | 19.882 | Cyclopropane, 1-ethenyl-2-hexeny | 2.25 |
6 | 20.289 | Butylidene Phthalide | 0.37 |
7 | 21.045 | Isobutylidenphthalide | 35.89 |
8 | 21.135 | n Butyl Phthalide | 11.92 |
9 | 21.833 | Menthofuran | 0.56 |
10 | 21.976 | n Butyl Phthalide | 11.5 |
11 | 26.080 | Phytol | 1.9 |
Effect of 14 days administration of different doses of LOE on serum levels of biochemical factors.
Groups | Glucose | Insulin | Triglycerides (mg/dl) | Total | HDL | LDL | Creatinine (mg/dl) | AST | ALT |
---|---|---|---|---|---|---|---|---|---|
Control | 85.6 ± 2.4 | 4.4 ± 0.01 | 67.5 ± 3 | 77 ± 3.2 | 41.1 ± 2.2 | 22.5 ±2 | 0.9 ± 0.0 | 137 ± 6 | 74 ± 4.7 |
Diabetic (D) | 467 | 1.08.0 | 93.0 | 108 | 24.1 | 65.4 | 1.3 | 238 | 188 |
D + Extract | 453 ± 6 | 2.0 ± 0.02 | 80.7 ± 1 | 82 ± 3.8b*** | 29.2 ± 0.8 | 36.86 | 0.9 | 235 ± 3 | 190 |
D + Extract | 430 ± 6.7 | 2.0 ± 0.0 | 61.7 ± 6b** | 44.5 ±3.5b*** | 32.5 ± 2.9b* | 19.66 ± 3.2b*** | 0.5 ± 0.01b*** | 173 ± | 147 |
D + Extract | 350 ± 16b*** | 2.1 | 72.3 ± 1b* | 48.6 ± 3.3b*** | 38 ± 3.4b** | 16.14 ± 1.7b*** | 0.5 ± 0.02b*** | 144 ± 10b*** | 57 ± 6.8b*** |
D + Gliben. | 179 ± 13b*** | 2.3 ± | 62.0 ± 2b** | 52.2 ± 1.7b*** | 38 ± 1.2b** | 11.8 ± 2.2b*** | 0.6 ± 0.03b*** | 127 ± | 66 |
Effect of different concentrations of LOE on glucose tolerance in normal rats
Groups | Glucose (mg/dl) | |||||
---|---|---|---|---|---|---|
0 min | 30 min | 60 min | 90 min | 120 min | 240 min | |
Control | 92.6 ± 4.0 | 133.0 ± 1.1 | 136 ± 2.0 | 127 ± 1.5 | 113 ± 3.2 | 92.3 ± 5.4 |
Extract | 92.0 ± 4.3 | 143.3 ± 3.8 | 141 ± 2.7 | 140 ± 2.8 | 137 ± 2.6 | 100±4.1** |
Extract | 89.3 ± 4.6 | 135.3 ± 10.8 | 136 ± 2.0 | 130 ± 1.7 | 120 ± 9.3 | 105 ± 8.0 |
Extract | 80.3 ± 3.7 | 125.0 ± 13.5 | 125 ± 7.6 | 110 ± 5.7* | 107 ± 4.9 | 95 ± 2.6 |
Glibenclamide | 74.0 ± 3.0* | 105 ± 2.5** | 101 ± 0.8** | 97 ± 2.0*** | 95.6 ± 2.9 | 84 ± 3.3 |
The docking scores, interaction loci and binding types of different known extract (LOE) compounds (ligands) with human pancreatic α- amylase (PDB ID: 5U3A) as receptor. All distance values are in angstrom (A°).
Extract ingredients | |||||||||||
---|---|---|---|---|---|---|---|---|---|---|---|
Rosmarinic acid | Quercetin | Hexanoic acid | Luteolin | Caffeic acid | Thymol | Carene | Phthalide | Phytol | Menthofuran | Chlorogenic acid | |
Docking score | -7.85 | -7.85 | -7.65 | -7.6 | -7.4 | -3.4 | -3.2 | -2.9 | -1.84 | - | - |
Interaction | H-bond | H-bond | H-bond | Mild | H-bond | H-bond | - | - |
Effects of LOE on antioxidant parameters in plasma, pancreas and liver tissues of diabetic rats
Groups | SOD | CAT | MDA | Plasma MDA (nmol/mL) | |||
---|---|---|---|---|---|---|---|
Pancreas | Liver | Pancreas | Liver | Pancreas | Liver | ||
Control | 16.7±0.4 | 11.2±0.2 | 48.4±2 | 209.9±1.7 | 0.4±0.0 | 3.2±0.2 | 0.9±0.0 |
Diabetic (D) | 11.2±0.6a*** | 8.0±0.8a* | 28.8±1.3a*** | 103.5±15.4a*** | 1.7±0.0a*** | 12.8±0.6a*** | 5.6±0.1a*** |
D + Extract | 16.6±0.5b*** | 14.8±0.4b*** | 43.0±1.9b** | 163.6±1.7b** | 0.6±0.0b*** | 3.6±0.1b*** | 1.7±0.1b*** |
D + Glibenclamide (20 mg/kg) | 16.9±0.3b*** | 16.2±0.7b*** | 44.6±1.4b** | 167.4±1b** | 0.5±0.0b*** | 3.4±0.1b*** | 1.6±0.1b*** |
Conclusion
In summary, the results of the present study show that LOE has significant antidyslipidemic and antioxidant properties and in silico studies predicted α- amylase inhibiton potency for some of its phytochemicals which may be responsible for antihyperglycemic effect of it. Also, in the light of these findings, we can justify Levisticum officinale (lovage) usage as an effective alternative medicine for diabetes complications such as dyslipidemia.
Acknowledgements
References
-
1.
Mozaffarian V. Flora of Iran (Umbelliferae Family). Tehran, Research Institute of Forests and Rangelands; 2007.
-
2.
Segebrecht S, Schilcher H. Ligustilide: guiding component for preparations of Levisticum officinale roots. Planta medica. 1989;55:572-73. [PubMed ID: 17262483].
-
3.
Yarnell E. Botanical medicines for the urinary tract. World J. Urol. 2002;20:285-93. [PubMed ID: 12522584].
-
4.
Shafaghat A. Chemical constituents, antimicrobial and antioxidant activity of the hexane extract from root and seed of Levisticum persicum Freyn and Bornm. J. Med. Res. 2011;5:5127-31.
-
5.
Gholamhoseinian A, Moradi MN, Sharififar F. Screening the methanol extracts of some Iranian plants for acetylcholinesterase inhibitory activity. Res. Pharm. Sci. 2009;4:105-12. [PubMed ID: 21589805].
-
6.
Gholamhoseinian A, Shahouzehi B, Sharififar F. Inhibitory effect of some plant extracts on pancreatic lipase. Int. J. Pharmacol. 2010;6:18-24.
-
7.
Gholamhoseinian A, Fallah H, Sharifi-far F, Mirtajaddini M. The inhibitory effect of some Iranian plants extracts on the alpha glucosidase. Iran. J. Basic Med. Sci. 2008;11:1-9.
-
8.
Bogucka-Kocka A, Smolarz H, Kocki J. Apoptotic activities of ethanol extracts from some Apiaceae on human leukaemia cell lines. Fitoterapia. 2008;79:487-97. [PubMed ID: 18672039].
-
9.
Lotfian Sargazi M, Saravani R, Shahraki A. Hydroalcoholic Extract of Levisticum officinale Increases cGMP Signaling Pathway by Down-Regulating PDE5 Expression and Induction of Apoptosis in MCF-7 and MDA-MB-468 Breast Cancer Cell Lines. Iran. Biomed. J. 2019;23:280-6. [PubMed ID: 30388886].
-
10.
Mohamadi N, Rajaei P, Moradalizadeh M, Amiri MS. Essential oil composition and antioxidant activity of Levisticum officinale Koch at various phenological stages. J. Med. Plants. 2017;16:45-55.
-
11.
Miran M, Monsef Esfahani H, Moridi Farimani M, Ali Ahmadi A, Nejad Ebrahimi S. Essential oil composition and antibacterial activity of Levisticum officinale Koch at different developmental stages. J. Essent. Oil- Bear. Plants. 2018;21:1051-5.
-
12.
Ciocarlan A, Dragalin I, Aricu A, Lupascu L, Ciocarlan N, Popescu V. Chemical composition and antimicrobial activity of the Levisticum officinale W. D. Koch essential oil. Chem. J. Moldovo. 2018;13:63-68.
-
13.
Tomsone L, Kruma Z, Talou T, Zhao TM. Natural antioxidants of horsradish and lovage extracted by accelerated solvent extraction. JHED. 2015;10:16-24.
-
14.
Chakrabarti R, Damarla RKB, Mullangi R, Sharma VM, Vikramadithyan RK, Rajagopalan R. Insulin sensitizing of Indigofera mysorensis. J. Ethnopharmacol. 2006;105:102-6. [PubMed ID: 16326056].
-
15.
Boivin GP, Bottomley MA, Schim PA, Goss L, Grobe N. Physiologic, behavioral, and histologic responses to various euthanasia methods in C57BL/6NTac male mice. J. Am. Assoc. Lab. Anim. Sci. 2017;56:69-78. [PubMed ID: 28905718].
-
16.
Honari N, Pouraboli I, Gharbi S. Antihyperglycemic property and insulin secreting activity of hydroalcoholic shoot extract of Thymus caramanicus Jalas: A wild predominant source of food additive in folk medicine. J. Func. Foods. 2018;46:128-35.
-
17.
Tatsuki R, Satoh K, Yamamoto A, Hoshi K, Ichihara K. Lipid peroxidation in the pancreas and other organs in streptozotocin diabetic rats. JPN. J. Pharmacol. 1997;75:267-73. [PubMed ID: 9434258].
-
18.
Aebi H. Catalase In-vitro. Methods Enzymol. 1984;105:121-6. [PubMed ID: 6727660].
-
19.
Giannopolitis CN, Ries SK. Superoxide dismutases I Occurrence in higher plants. Plant Physiol. 1977;59:309-14. [PubMed ID: 16659839].
-
20.
Bradford MM. A rapid and sensitive method for the quantitation of microgram quantities of protein utilizing the principle of protein-dye binding. Anal. Biochem. 1976;72:248-54. [PubMed ID: 942051].
-
21.
Ohkawa H, Ohishi N, Yagi K. Assay for lipid peroxides in animal tissues by thiobarbituric acid reaction. Anal. Biochem. 1979;95:351-8. [PubMed ID: 36810].
-
22.
Jamall IS, Smith JC. Effects of cadmium on glutathione peroxidase, superoxide dismutase, and lipid peroxidation in the rat heart: a possible mechanism of cadmium cardiotoxicity. Toxicol. Appl. Pharmacol. 1985;80:33-42. [PubMed ID: 4024106].
-
23.
Kurtel H, Granger DN, Tso P, Grisham MB. Vulnerability of intestinal interstitial fluid to oxidant stress. J. Physiol. Gastrointest. Liver Physiol. 1992;263:G573-G578.
-
24.
Livak KJ, Schmittgen TD. Analysis of relative gene expression data using real-time quantitative PCR and the 2− ΔΔCT method. Methods. 2001;25:402-8. [PubMed ID: 11846609].
-
25.
Giancarlo S, Rosa LM, Nadjafi F, Francesco M. Hypoglycaemic activity of two spices extracts: Rhus coriariaL and Bunium persicum Boiss. Nat. Prod. Res. 2006;20:882-6. [PubMed ID: 16753927].
-
26.
Harbone JB. Phytochemical methods. London: Chapman and Hall, Ltd; 1973.
-
27.
Singleton VL, Orthofer R, Lamuela-Raventós RM. Analysis of total phenols and other oxidation substrates and antioxidants by means of folin-ciocalteu reagent. Methods Enzymol. 1999;299:152-78.
-
28.
Kumazawa S, Hamasaka T, Nakayama T. Antioxidant activity of propolis of various geographic origins. Food Chem. 2004;84:329-39.
-
29.
Ziedan NI, Hamdy R, Cavaliere A, Kourti M, Prencipe F, Brancale A, Jones AT, Westwell AD. Virtual screening, SAR and discovery of 5-(indole-3-yl)-2-[(2-nitrophenyl) amino][1,3,4]-oxadiazole as a novel Bcl-2 inhibitor. Chem. Biol. Drug Des. 2017;90:1-9.
-
30.
Hussein WM, Islam NU, Guddat LW, Schenk G, McGeary RP. Penicillin inhibitors of purple acid phosphatase. Bioorg. Med. Chem. Lett. 2012;22:2555-59. [PubMed ID: 22366658].
-
31.
Heidarpour O, Souri MK, Omidbaigi R. Changes in content and constituents of essential oil in different plant parts of lovage (Levisticum officinale Koch. Cv. Budakalaszi) cultivated in Iran. J. Essent. Oil- Bear. Plants. 2013;16:318-22.
-
32.
Abbate SL, Brunzell JD. Pathophysiology of hyperlipidemia in diabetes mellitus. J. Cardiovasc. Pharmacol. 1990;16:S1-S7.
-
33.
Concepción MN, Pilar MM, Martin A, Jiménez J, Utrilla MP. Free radical scavenger and antihepatotoxic activity of Rosmarinus tomentosus. Planta Medica. 1993;59:312-14. [PubMed ID: 8372145].
-
34.
de Sotillo DVR, Hadley M. Chlorogenic acid modifies plasma and liver concentrations of: cholesterol, triacylglycerol, and minerals in (fa/fa) Zucker rats. J. Nutr. Biochem. 2002;13:717-26. [PubMed ID: 12550056].
-
35.
Johnston KL, Clifford MN, Morgan LM. Coffee acutely modifies gastrointestinal hormone secretion and glucose tolerance in humans: glycemic effects of chlorogenic acid and caffeine. Am. J. Clin. Nutr. 2003;78:728-33. [PubMed ID: 14522730].
-
36.
Runtuwene J, Cheng K-C, Asakawa A, Amitani H, Amitani M, Morinaga A, Takimoto Y, Kairupan BHR, Inui A. Rosmarinic acid ameliorates hyperglycemia and insulin sensitivity in diabetic rats, potentially by modulating the expression of PEPCK and GLUT4. Drug des. Devel. Ther. 2016;10:2193-202.
-
37.
Vessal M, Hemmati M, Vasei M. Antidiabetic effects of quercetin in streptozocin-induced diabetic rats. Comp. Biochem. Physiol. Part C: Toxicol. Pharmacol. 2003;135:357-64.
-
38.
Saravanan S, Pari L. Role of thymol on hyperglycemia and hyperlipidemia in high fat diet-induced type 2 diabetic C57BL/6J mice. Eur. J. Pharmacol. 2015;761:279-87. [PubMed ID: 26007642].
-
39.
Elmazar MM, El-Abhar HS, Schaalan MF, Farag NA. Phytol/Phytanic acid and insulin resistance: potential role of phytanic acid proven by docking simulation and modulation of biochemical alterations. PLoS ONE. 2013;8:e45638. [PubMed ID: 23300941].
-
40.
Joost H, Steinfelder H. Insulin-like stimulation of glucose transport in isolated adipocytes by fatty acids. Biochem. Biophys. Res. Commun. 1985;128:1358-63. [PubMed ID: 3890851].
-
41.
Tabatabai NM, Sharma M, Blumenthal SS, Petering DH. Enhanced expressions of sodium–glucose cotransporters in the kidneys of diabetic Zucker rats. Diabetes Res. Clin. Pract. 2009;83:e27-e30. [PubMed ID: 19095325].
-
42.
Philpott D, Butzner J, Meddings J. Regulation of intestinal glucose transport. Can. J. Physiol. Pharmacol. 1992;70:1201-7. [PubMed ID: 1493588].
-
43.
Peng BJ, Qi Z, Zhong YL, Xu S, Wang Z. Chlorogenic acid maintains glucose homeostasis through modulating the expression of SGLT-1, GLUT-2, and PLG in different intestinal segments of Sprague-Dawley rats fed a high-fat diet. Biomed. Environ. Sci. 2015;28:894-903. [PubMed ID: 26777909].
-
44.
Farrell TL, Ellam SL, Forrelli T, Williamson G. Attenuation of glucose transport across Caco‐2 cell monolayers by a polyphenol‐rich herbal extract: Interactions with SGLT1 and GLUT2 transporters. Biofactors. 2013;39:448-56. [PubMed ID: 23361943].
-
45.
Kwon O, Eck P, Chen S, Corpe CP, Lee JH, Kruhlak M, Levine M. Inhibition of the intestinal glucose transporter GLUT2 by flavonoids. FASEB J. 2007;21:366-77. [PubMed ID: 17172639].
-
46.
Kazeem MI, Oyedapo BF, Raimi OG, Adu OB. Evaluation of Ficus exasperata Vahl leaf extracts in the management of diabetes mellitus in-vitro. J. Med. Sci. 2013;13:269-75.
-
47.
Tadera K, Minami Y, Takamatsu K, Matsuoka T. Inhibition of α-glucosidase and α-amylase by flavonoids. J. Nutr. Sci. Vitaminol. 2006;52:149-53. [PubMed ID: 16802696].
-
48.
Nyambe-Silavwe H, Villa-Rodriguez JA, Ifie I, Holmes M, Aydin E, Jensen JM, Williamson G. Inhibition of human α-amylase by dietary polyphenols. J. Funct. Foods. 2015;19:723-32.
-
49.
McCue PP, Shetty K. Inhibitory effects of rosmarinic acid extracts on porcine pancreatic amylase in-vitro. Asia Pacific J. Clin. Nutr. 2004;13:101-6.
-
50.
Komaki E, Yamaguchi S, Isafumi M, Kinoshita M, Kakehi K, Tsukada Y. Identification of anti-α-amylase components from olive leaf extracts. Food Sci. Technol. Res. 2003;9:35-9.
-
51.
Brayer GD, Luo Y, Withers SG. The structure of human pancreatic α-amylase at 18 Å resolution and comparisons with related enzymes. Protein Sci. 1995;4:1730-42. [PubMed ID: 8528071].
-
52.
Rasouli H, Hosseini-Ghazvini SMB, Adibi H, Khodarahmi R. Differential α-amylase/α-glucosidase inhibitory activities of plant-derived phenolic compounds: a virtual screening perspective for the treatment of obesity and diabetes. Food Funct. 2017;8:1942-54. [PubMed ID: 28470323].
-
53.
Roy S, Sehgal R, Padhy B, Kumar V. Antioxidant and protective effect of latex of Calotropis procera against alloxan-induced diabetes in rats. J. Ethnopharmacol. 2005;102:470-73. [PubMed ID: 16054794].
-
54.
Pouraboli I, Nazari S, Sabet N, Sharififar F, Jafari M. Antidiabetic, antioxidant, and antilipid peroxidative activities of Dracocephalum polychaetum shoot extract in streptozotocin-induced diabetic rats: In-vivo and in-vitro studies. Pharma. Biol. 2016;54:272-78.
-
55.
Kono Y, Kobayashi K, Tagawa S, Adachi K, Ueda A, Sawa Y, Shibata H. Antioxidant activity of polyphenolics in diets: rate constants of reactions of chlorogenic acid and caffeic acid with reactive species of oxygen and nitrogen. Biochim. Biophys. Acta. 1997;1335:335-42. [PubMed ID: 9202196].
-
56.
Zang Y, Igarashi K, Li Y. Anti-diabetic effects of luteolin and luteolin-7-O-glucoside on KK-A y mice. Biosci. Biotechnol. Biochem. 2016;80:1580-86. [PubMed ID: 27170065].
-
57.
Racine P, Auffray B. Quenching of singlet molecular oxygen by Commiphora myrrha extracts and menthofuran. Fitoterapia. 2005;76:316-23. [PubMed ID: 15890469].
-
58.
Zhu F, Asada T, Sato A, Xu SH, Zheng W. Rosmarinic acid extract for antioxidant, antiallergic, and α-glucosidase inhibitory activities, isolated by supramolecular technique and solvent extraction from Perilla leaves. J. Agric. Food. Chem. 2014;62:885-92. [PubMed ID: 24400891].