Abstract
Keywords
Juglone Resveratrol Kefir Ehrlich ascites Bax Bcl-2 p53 Caspase
Introduction
Cancer, a disease of genes directing the proliferation, differentiation, and the formation of cells, is the leading reason for death worldwide (1). Environmental and genetic factors determine the fate of cancer, which affects almost all body tissues, organs, and fluids (1, 2). Ehrlich Ascites Carcinoma (EAC) that could be developed from murine mammary spontaneously is modified to acidify form experimentally. This model can be kept going in outbred animals with serial intraperitoneal (i/p) passages. With their aggressive tumorigenic ability, EAC cells can rapidly proliferate and metastasize from peritoneal acid fluids to almost any body organ. Besides, they can also modulate some endogenous biochemical parameters in different body fluids, including serum and tissues (3, 4). Rodents’ peritoneal cavity might be used to passage and maintain the EAC cells, and the EAC model is recently used for tumor biology studies (5). The ability to monitor ascites carcinoma cells after injection to the animals, free neoplastic cell growing nature in abdominal fluids, and requirement of any mechanical stress for the disintegration of the cells make them as perfect cancer model for mechanistic cancer studies.
Recently, numerous bioactive compounds extracted from medicinal plants have been tested for their anti-cancer activity (6, 7). Several studies have reported the potential therapeutic effect of resveratrol (7, 8) and juglone (10-12) towards several cancer diseases. Pharmacological studies on resveratrol have reported its anti-oxidant, anti-inflammatory, anti-cancer, anti-aging, anti-obesity, anti-diabetic, cardio protective, neuroprotective, and anti-microbial properties (13). It also enhances the death of tumor cells by inhibiting Bcl-2 and upregulating Caspase-3 expression (14, 15). Furthermore, resveratrol could increase p53 and p21 by providing a balance between oxidative/anti-oxidative status (16, 17) and potentially causes cell death by inducing apoptosis. An irreversible inhibitor of the Pin1 (peptidyl-prolylcis/trans isomerase) enzyme; juglone is a plant-originated naphthoquinone which exhibits intense anticancer activity against numerous models in-vitro (18-21). Inhibition of Pin1 triggers mitogen-activated protein kinases that could contribute the cell survival (22, 23). Moreover, it is implicated in extensive diversity of clinical circumstances including immune response (24), allergy (25), cancer (26, 27), hyperparathyroidism (28), rheumatoid arthritis (29), and cardiac fibrosis (30-32). Recent studies revealed that juglone also induces apoptosis through the pathways dependent on mitochondrial pathways by the induction of the Bax/Bcl-2 ratio (33). Likewise, juglone increased Ca2+ concentration and Caspase-3 activity on human breast cancer cells (34). Its anti-tumor activity is usually linked to the damages on DNA due to alkylating species or oxygen radicals (35). Potentiation of tumor necrosis factor-α (TNF-α) related apoptosis in human melanoma cells via upregulating Caspase-3, p38, and p53 were also reported (36). Although juglone and/or resveratrol were shown to have anti-cancer effects in many in-vitro studies, promising results either alone or in combination in clinical studies have not been achieved yet.
Probiotics are currently defined as nonpathogenic microorganisms that improve human health due to their anti-inflammatory and immunoregulatory properties (37). Even though their action mechanism is not well-established, it is thought to be due to their metabolic products (38). A natural complex fermented milk product; kefir contains probiotic bacteria, primarily composed of Lactobacillus, Leuconostoc, Kluyveromyces, Pichia, and Saccharomycesspecies. It has anti-inflammatory, anti-allergenic, anti-oxidative, anti-tumor, cholesterol-lowering, constipation-lessening, and anti-microbial properties (39). Kefir also increases the anti-oxidant potential and regulates several biological processes such as apoptosis and proliferation (40, 41), and its apoptotic effects are attributed to the stimulation of Caspase-3 and Bax, repression of Bcl-2, and membrane potential reduction of mitochondria (42). Therefore, juglone, resveratrol, and kefir could have promising therapeutic potential against cancer treatments individually. However, in the current literature, there is no study showing the bioactive efficacy of combined juglone, resveratrol, and kefir solutions on cancer cell growth. Additionally, any study demonstrated the anti-cancer effects of secondary metabolites of kefir. In this study, we hypothesized that secondary metabolites of kefir rather than the bacterial part could have therapeutically properties. We also proposed that supplementation of kefir secondary metabolites with juglone and resveratrol and their potent secondary by-products obtained during the fermentation process could have strong pharmaceutical potential for cancer cell destruction. Therefore, this study was conducted to demonstrate the potent in-vivo apoptotic and anti-apoptotic effects of kefir secondary metabolites enriched with resveratrol and juglone and their potent by-products on of Ehrlich Ascites Carcinoma cells and of EAC-bearing BALB/c mice.
Experimental
Drugs and Preparation of the Solution JRK
Chemicals for the bioassays were obtained from Sigma Aldrich (St. Louis, Missouri, USA) at the highest purity available. Juglone (H4700316) and resveratrol (EPRS-Y0001194) were dissolved in 0.1% DMSO, and the prepared mix has a ratio of 1:2, respectively. Kefir yeast contains Lactobacillus helveticus, Lactobacillus parakefiri, Lactobacillus casei, Lactobacillus reuteri, Lactobacillus acidophilus, Lactobacillus bulgaricus, Lactobacillus fermentum, Leuconostocmesentereoides, Lactococcuslactis, Lactobacillus kefiranofacien, and Acetobacterpasteurianus, Streptococcus thermophilus, Bifidobacteriumbifidu, Kluyveromycesmarxianus, Saccharomyces cerevisiae, and Kluyveromyceslactis were obtained from Danem-Kefir (Isparta, TURKEY). The bacteria in the kefir yeast were incubated in 10 mL of broth (DifcoTMLactobacilli MRS Broth 288130) for one night at 35 oC and grown at the 1.5x10+9 cell/mL. During the fermentation process, juglone and resveratrol (200 µM-400 µM, respectively) were prepared (ethanol, <1% final concentration) and added to the broth and exposed for 48 h. The doses of juglone and resveratrol were determined according to parallel trials; resveratrol (43-45) and juglone (43, 46, 47) doses were chosen from previous in-vitro studies. Then, the solution was filtered through a 0.45 µm membrane, and the bacterial part was removed, and the remaining JRK solution was stored at -85 oC until use. The numbers of bacteria were determined with trypan blue exclusion technique with Breed method.
Animals and Tumor Model
Animal protocols were permitted in advance by the Local Ethics Commission for the Animal Research Studies at the Afyon Kocatepe University (AKUHADYEK-49533702.08). Male BALB/c inbred mice (18–22 g) were obtained from KOBAY A.Ş. (Ankara, TURKEY). Animals were kept growing in temperature-controlled rooms (20-22 °C) with a 12-h light-dark cycle. They were nourished with a typical rodent diet composed of 23% protein, 62% starch, 4% fat, 7% cellulose, standard vitamins, and a mixture of salt (chow pellet) and drinking water ad libitum. The EAC cells were gained from Afyonkarahisar Health Sciences University, Faculty of Medicine, Department of Anatomy (Afyonkarahisar, TURKEY). They were perpetuated with intraperitoneal (i/p) transplantation of 1x10+6 viable tumor cells in mice with a 25G needle to be used in future experimental studies. After five days of observation, JRK solution (0.1 mL/day i/p) was applied to the EAC treated mice for five days. Following the treatments, body weights and waist circumference of the mice were measured, and then ascites were isolated by a pre-scarification injector. The EAC cells’ viability was assessed with trypan blue exclusion technique (48).
Experimental Study Design
After acclimation for one-week, male BALB/c inbred mice were randomly separated into three groups with ten animals each. Study protocol was designed as follows; Group (1): Non-EAC-bearing mice and untreated control group (Control), Group (2): EAC-bearing mice; injected 1×10+6 viable tumor cells in 1 mL of saline (Sham), Group (3): Applied JRK solution (0.1 mL/day i/p) to EAC-bearing mice for five days (JRK). Cancer modelling lasted five days. After this period, 0.1 mL saline was injected into the control and sham groups while applying the JRK solution.
Measurement of Apoptotic/Anti-apoptotic Biomarkers in the Plasma and EAC
Cardiac blood and EAC samples of mice were centrifuged immediately at 10.000 g for 30 min, and the cell pellet and supernatants were collected. Total protein levels in both plasma and EAC were determined by the Brilliant Blue G stain method using Sigma commercial kits (Saint Louis, Missouri, USA) as indicated in the package insert. The apoptotic Bax, p53, Caspase-3, Caspase-8, Caspase-9, and anti-apoptotic APAF-1 Bcl-2, Bcl-xl protein levels were determined by commercial rat specific ELISA kits (Cloud-Clone Corp., Export Processing Zone, Wuhan, Hubei, PRC) according to the manufacturer’s instructions. ELISA microplate reader (ChemWell 2910, Awareness Technology, Inc. Martin Hwy, Palm City) was utilized for the spectrophotometric measurements.
Determination of the Expressions Levels of Apoptotic and Anti-Apoptotic Genes
After the animal treatments, RNA samples were isolated from the tissue and EAC cells with a commercial RNA isolation kit (Qiagen, Netherlands) along with the manufacturer procedure. Quality and quantity were assessed with spectrophotometry and electrophoresis. Thereafter, cDNA synthesis was conducted using a commercial first-strand cDNA synthesis kit (Thermo Scientific, USA). BAX, CASPASE-6, CASPASE-8, and CASPASE-9 genes expression levels were determined with quantitative real-time polymerase chain reaction (Roche, Switzerland) as we described in detail previously (49). Briefly, SYBR Green Master Mix (Roche FastStart Universal SYBR Green Master Mix), cDNA samples, and the primer pairs (Table 1) were mixed, and the qRT-PCR was conducted. Triplicate measurements were performed for each sample, and the melt analysis confirmed the specificity of PCR reactions. For the normalization of data, internal control gapdh was utilized, and the relative expression of genes was calculated by LightCycler 480 SW 1.5.1 software (Roche, USA).
Immunocytochemical Analysis
The suspended EAC cells were first pelleted by centrifugation at low speed for 5 min, and then, they were re-suspended in 4% formaldehyde in PBS. After warming up for 10 min at room temperature, EAC cells were pelleted again by centrifugation for 5 min at room temperature and resuspended in 80% ethanol. Fixed cells were stored at +4 oC, and then the smears were stained with immunocytochemical (ICC) staining. For ICC, immobilized cells were incubated with 0.2% Tween-20 solution for 20 min for permeability. Afterward, the slides were washed with PBS and incubated for 10 min in 3% hydrogen peroxide/methanol solution. After washing, they were incubated with Bax (sc-526, Santa Cruz Biotechnology, 1/50 dilution), Bcl-2 (sc-7382, Santa Cruz Biotechnology, 1/50 dilution), p53 (sc-6243, Santa Cruz Biotechnology, 1/50 dilution), Caspase-3 (ab-4051, Abcam, 1/50 dilution) primary antibodies for 90 min. Then, HRP-conjugated secondary antibody kit (Anti-polyvalent HRP, Labvision Corp, Fremont, CA) was used to probe primary antibodies. After coloring with the chromogen, counter-staining with Mayer Hematoxylin staining was performed, and the slides were inspected using a light microscope. Cytoplasmic immunoreactivity for Bax, Bcl-2, and Caspase-3 and nuclear and cytoplasmic immunoreactivity for p53 were considered as specific. Bax, Bcl-2, p53, and Caspase-3 expressions were calculated as the percentage of positive cells out of the total cell number at five different randomly chosen areas from each slide under 400x magnification and the data are presented as percent positive cells.
Statistical Analysis
Data are represented as mean ± standard error of the mean throughout the manuscript. Student’s t-test or one-way ANOVA followed by the Bonferroni post hoc test were utilized where appropriate to determine statistically significant differences. The level of significance was set as P values which are smaller than 0.05.
Results
The Effects of JRK Solution Body Weight, Waist Circumference and EAC Cell Viability
As shown in Table 2, Sham mice gained significantly higher weight and waist circumference than the control group (P < 0.05), whereas the terminal body weight and waist circumference of JRK-treated mice showed a tendency towards a decrease compared to Sham group.
Besides, the number of viable cells increased significantly (252.4 ± 18.4x10+6 cell/mL) in EAC cells-injected (10+6) mice at the end of the 5th day (Figure 1). Notably, JRK treatment reduced the number of viable EAC cells as compared to the Sham group (P < 0.05).
The Effects of JRK Solution on Apoptotic/Anti-apoptotic Parameters in the Plasma and EAC Cells
Apoptotic/anti-apoptotic biomarkers in the plasma of Sham and JRK supplemented group were evaluated with ELISA and qRT-PCR. Accordingly, the Bax, Bcl-xl, p53, Caspase-8, Caspase-9 and APAF-1 protein levels were all similar, while Bcl-2 was upregulated and Caspase-3 was downregulated in the plasma of Sham group. The changes in plasma Bcl-2 and Caspase-3 levels were normalized with JRK solution (data are not shown). Furthermore, there was a significant increase in Bax, p53, Caspase-3, 8, 9 and APAF-1 protein contents in the EAC-cell line of JRK-treated mice as compared to the sham group (Figure 2), while Bcl-xl levels were suppressed (Figure 2C) with JRK treatment. The mRNA expression levels of BAX, CASPASE-6, CASPASE-8, andCASPASE-9 were assessed in the EAC cell line, and the values are demonstrated in Figure 3. Accordingly, significantly higher levels of BAX (Figure 3A), CASPASE-6 (Figure 3B), CASPASE-8 (Figure 3C), and CASPASE-9 (Figure 3D) expressions were detected in JRK groups as compared to Sham. For technical reasons, it was not possible to detect CASPASE-3 gene expression in the EAC cells.
The Influences of JRK Solution in the EAC cells: Immunocytochemical Evaluation
Immunocytochemical examinations confirmed the diffuse and higher positive staining for Bax, p53, and Caspase-3 proteins in EAC cells of the JRK-treated group, but positive staining for Bcl-2 in the Sham mice (Figure 4A). As shown in Figure 4B, Bax (14.6 ± 1.4–36.5 ± 5.8), p53 (10.3 ± 2.3–26 ± 4.4), and Caspase-3 (9.75 ± 1–32.8 ± 3.1) positive rate of (%) EAC cells of JRK-treated mice were enhanced significantly compared to Sham group, whereas Bcl-2 (42.6 ± 3.3–21.5 ± 3.2) was suppressed.
Primer sequences of bax, caspase-6, caspase-8, caspase-9, and internal standard gapdhused for the mRNA expression determination of qRT-PCR
Gene | Reverse Primer | Forward Primer | Gene Bank Accession Number | Product Size (bp) |
---|---|---|---|---|
GAPDH | TCCTTGGAGGCCATGTGGGCCA | TGATGACATCAAGAAGGTGGT | NM-017008.4 | 240 |
BAX | GCTCAGCTTCTTGGTGGATG | CCTTTTTGCTACAGGGTTTCAT | NM-017059.2 | 110 |
CASPASE- 6 | GACCGACTAAAACAGGCCC | AATTACTGTGCGCAAATGCC | XM-008761476.2 | 246 |
CASPASE-8 | GCTGTAACCTGTCGCCG | ACCTCCGGTGTTTTATAGTTCC | NM-022277.1 | 179 |
CASPASE-9 | ATGGTCTTTCTGCTCACCAC | GTCACGGCTTTGATGGAGAT | NM-031632.1 | 246 |
Initial and terminal body weight (BW), the number of viable cells, and the waist circumference (WC) of the experimental groups
Groups | Initial BW (g) | Terminal BW (g) | WC (cm) | Cell Alive x10+6 |
---|---|---|---|---|
JRK | 21.3 ± 0.4 | 25 ± 1.1 | 10.2 ± 0.4 | 119.1±7.4 * |
Control | 20.3 ± 0.9 | 21.8 ± 1.5 | 4 ± 0.1 | - |
Sham | 20.9 ± 0.7 | 25.9 ± 1 # | 11 ± 0.5 # | 252.4±18.4 |
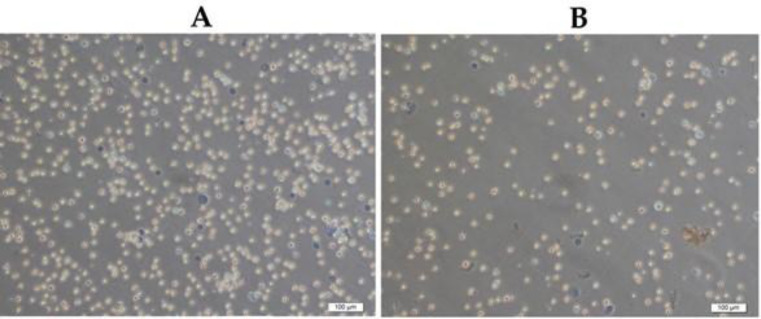
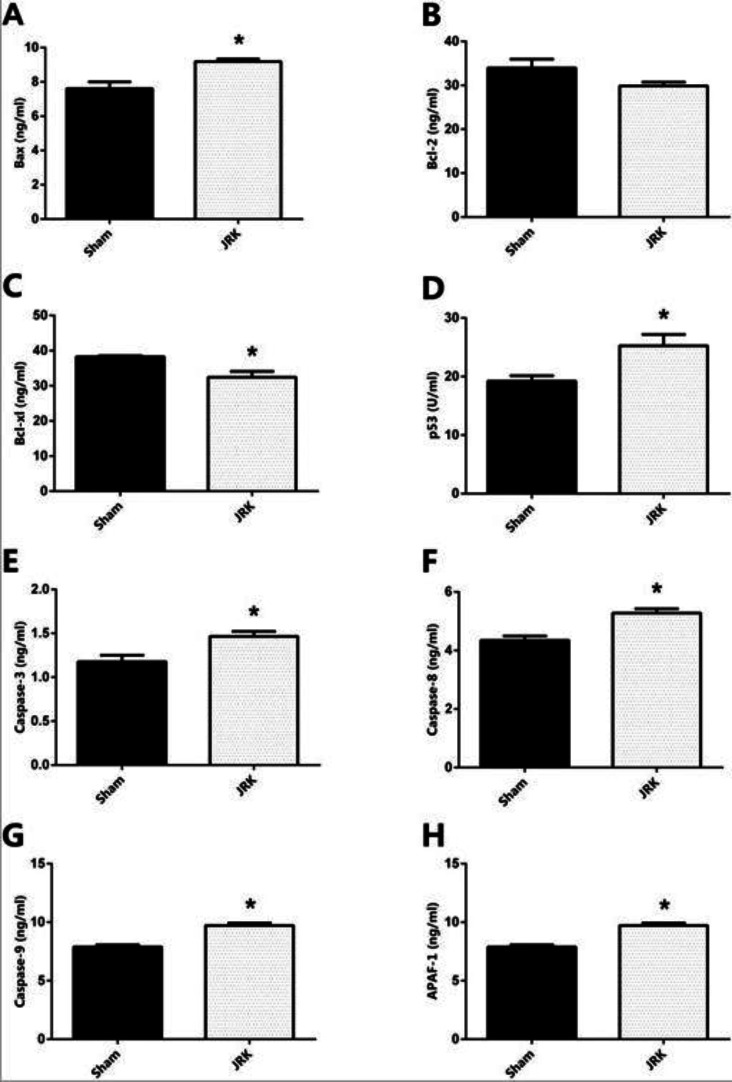
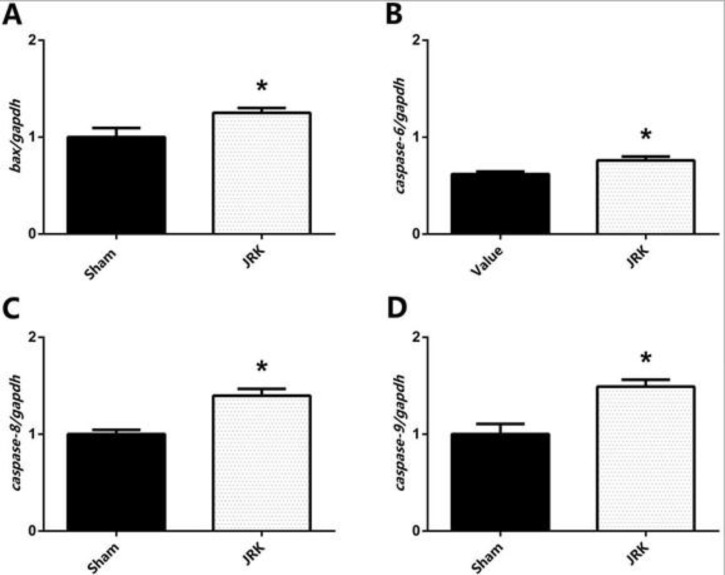
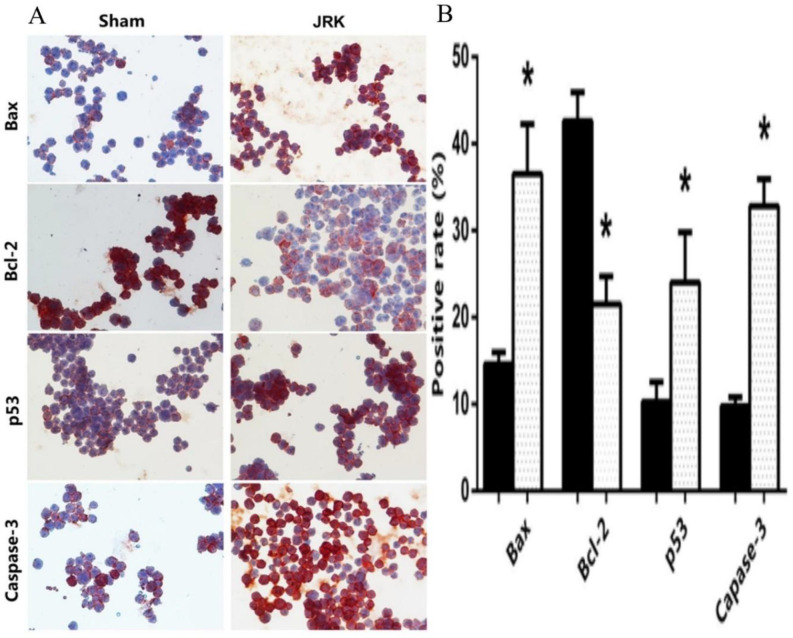
Discussion
Cancer is a global problem of healthcare due to worldwide rising incidence and limited therapeutic options. Not only the ineffectiveness of treatments and side effects but also the complete remission in many cases prevents radical treatment of the disease (50). Therefore, it is essential to discover new therapeutic targets as well as new molecules with satisfying potential. Plant-derived compounds; juglone and resveratrol might be such potential therapeutic agents. Besides, dietary probiotics have also great potential for cancer prevention and treatment. Several studies have shown that juglone (10, 11, 18, 19, 33, 37, 51) resveratrol (8, 9, 12, 14-16) and kefir (41, 42) have positive effects on cancer treatment of colon, pancreas, lung, prostate, breast, and thyroid with human melanoma and myeloid leukaemia. Although the tremendous anti-cancer efficacy of the juglone through the Pin1 inhibition is well-known, its potent cytotoxic activity suppresses its selectivity (19). In this regard, taking juglone together with potent anti-oxidants would have a synergistic effect to reduce the cytotoxicity over healthy cells and to ensure appropriate pharmacotherapy (51). In this regard, we aimed to increase the selectivity of the juglone with a different strategy by investigating the effects of the end products of the kefir fermentation under juglone and resveratrol supplements.
Examination of the morphological alterations of the animals before decapitation indicated the body weight gain and waist circumference increment in the mice treated with EAC and JRK groups. This situation could be attributed to the increased intraabdominal fluid five days after the injection of EAC cells. Importantly, the number of viable EAC cells in the intraabdominal fluid from the mice in which the JRK solution is administered was reduced by more than 50% compared to the Sham group indicating the cytotoxic efficacy of JRK over EAC cells. These results are in parallel with the literature since juglone and/or resveratrol can induce apoptosis and repair DNA damage by affecting some endogenous factors. Exemplarily, juglone showed anti-cancer effect by inducing ROS/p38/p53 pathway in melanoma cells (36).
Similarly, resveratrol and kefir induced expression of Bax, while inhibiting Bcl-2, and reinforcing the expression of p53 (52, 53). However, it was determined that consumption of kefir increased caspase-3 in myeloid leukemia cells (54). These parameters and mechanisms involved in cancer pathology can induce apoptosis. Our results also demonstrated the induction of apoptotic pathways and the suppression of anti-apoptotic markers in EAC cells of the mice treated with JRK-fractions. In EAC-cells, there was a general induction of apoptotic markers; Bax, p53, Caspase-3, 8, 9 and APAF-1 with JRK application. These inductions of apoptotic markers are also evidenced with the upregulated gene expression levels of BAX, CASPASE-6, CASPASE-8, andCASPASE-9. Besides, JRK downregulated the anti-apoptotic Bcl2-xl in EAC-cells of BALB/c in plasma of male mice. The present study also revealed proapoptotic Bax, p53, Caspase-8, Caspase-9, and APAF-1 and anti-apoptotic Bcl-xl levels do not alter in the serum of Sham group. However, upregulated Bcl-2 and suppressed Caspase-3 levels in the Sham group were normalized by JRK treatment.
In general respect, mitochondrial outer membrane permeabilization is an irreversible master regulator for the entry of cells towards the death which is strongly affected by synchronizing activities of Bax and Bcl-2 family proteins. Following the changes in membrane permeability, cytochrome C is released to the cytoplasm to activate particular proteases triggering apoptosis together with the APAF-1 association. In this process, while Bax potentially activates the apoptosis, Bcl-xl suppresses it by interacting with Bax (55, 56). Besides, cellular stress can also stimulate apoptosis via acetylation of p53 protein, which arrests the cell cycle under normal circumstances. Upon acetylation, p53 activates downstream proapoptotic target genes such as BAX. As a family of cysteine proteases and the master mediators of apoptotic cell death, Caspases are activated through cytochrome C release together with APAF-1 oligomerization (57, 58). Caspase-8 and Caspase-9 are initially activated with the cytochrome C and then lead to the proteolytic activation of other downstream proteases such as Caspase-3 and Caspase-6 which eventually shut down cellular functions by cleaving many proteins during the programmed cell death (59). The EAC modelling that we create shows first i/p distribution and systemic changes become apparent after a certain period; therewithal, we have set 10 days between the first injection and decapitation. There might have been any change in the subcomponents in the mechanism of apoptosis in the plasma. The modelling and the treatment that we applied cause changes in the essential apoptotic proteins; Bcl-2 and Caspase-3. This result means that systemic changes are in the initiation phase. In this context, JRK treatment decreased the anti-apoptotic Bcl-2 level and increased the apoptotic Caspase-3 level, which is a necessary consequence. In EAC cells, the proapoptotic factors; Bax, p53, Caspase-3, 8, 9 increased and anti-apoptotic Bcl-xl and Bcl-2 protein levels decreased in a synchronized way. These changes might induce the process leading to cell death. Although we used the JRK solution for the first time, other supporting literature information is available with that we can compare results. Firstly, resveratrol augmented the expression of apoptotic genes such as P53, BAX, CASPASE-3, and CASPASE-8 and promoted apoptosis with a pathway depending on mitochondria in breast cancer modelling (60). Similarly, resveratrol inhibited tumor angiogenesis as demonstrated by the reduction of microvessel density by vascular endothelial growth factor (VEGF) and VEGF receptor type-2 in the EAC-bearing mice (61). Moreover, juglone hindered EAC cells with reduced p-Akt levels (62). Another study presented the enrichment of BAX, CASPASE-3, CASPASE-8, and CASPASE-9 with juglone to inhibit the growth of breast cancer cells (63). Similarly, in our study, JRK solution amplified BAX, CASPASE-6, CASPASE-8, and CASPASE-9gene expressions as compared to Sham, which is in line with the change of their protein levels. Furthermore, the positive rate of the EAC cell line was examined by immunostaining of Bax, Bcl-2, p53, and Caspase-3. Staining of proapoptotic proteins Bax, p53, and Caspase-3 appear an increase following JRK-treatment, which is a probable compensatory mechanism against reduced Bcl-2. These results appear to be consistent with the protein and gene expression results of the measured parameters of the apoptotic mechanism.
Conclusion
In conclusion, in this study, we highlighted the role, and the associated molecular mechanisms of JRK solution in cancer treatment, by mainly focusing on apoptotic mechanisms in EAC-bearing BALB/c mice. Even though this first stage study demonstrated that JRK is beneficial in cancer treatment and might be useful in clinical therapy, it necessitates additional clinical evidence. Further studies should be performed to determine the chemical composition of JRK solution, the secondary metabolites of kefir fermentation, juglone and/or resveratrol derivatives that might be formed through the fermentation process. To understand concrete molecular alterations of EAC and favourable effects of JRK, further studies should focuson the identification of JRK solution composition, long term, and dose-dependent response to JRK and determine survival rate and time.
Acknowledgements
References
-
1.
Torres G, Zakhary SM, Leheste JR. An evolutionary perspective of neoplastic diseases in the universe. Cureus. 2019;11:e4030. [PubMed ID: 31011493].
-
2.
El-Far M, Salah N, Essam A, Abd El-Azim A, Karam M, El-Sherbiny IM. Potential anticancer activity and mechanism of action of nano formulated curcumin in experimental Ehrlich Ascites Carcinoma-bearing animals. Nanomedicine London). 2019;14:553-73.
-
3.
Eisa NH, Said HS, ElSherbiny NM, Eissa LA, El-Shishtawy MM. Phenethylisothiocyanate triggers apoptosis, combats oxidative stress and inhibits growth of Ehrlich Ascites Carcinoma mouse model. Iran. J. Pharm. Res. 2018;17:1328-38. [PubMed ID: 30568691].
-
4.
Verma AS, Singh A, Mallick P, Dwivedi PD. Cysteine supplementation mitigates the toxicity associated with antitumor therapy of Ehrlich’s ascites fluid adsorbed over protein containing Staphylococcus aureus Cowan I. J. Pharm. Bioallied. Sci. 2019;11:23-32. [PubMed ID: 30906136].
-
5.
Mishra S, Tamta AK, Sarikhani M, Desingu PA, Kizkekra SM, Pandit AS, Kumar S, Khan D, Raghavan SC, Sundaresan NR. Subcutaneous Ehrlich Ascites Carcinoma mice model for studying cancer-induced cardiomyopathy. Sci. Rep. 2018;8:5599. [PubMed ID: 29618792].
-
6.
Gupta M, Mazumder UK, Kumar RS, Sivakumar T, Vamsi ML. Antitumor activity and antioxidant status of Caesalpiniabonducella against Ehrlich Ascites Carcinoma in Swiss albino mice. J. Pharmacol. Sci. 2004;94:177-84. [PubMed ID: 14978356].
-
7.
Taneja S, Qazi G. Bioactive molecules in medicinal plants: a perspective in their therapeutic action. In: Chorghade MS, editor. Drug Discovery and Development. JohnWiley and Sons, Inc: New Jersey, USA; 2007. p. 1-50.
-
8.
Bortolotti C, Kunit T, Moder A, Hufnagl C, Schmidt S, Hartl A, Langelueddecke C, Fürst J, Geibel JP, Ritter M, Jakab M. The phytostilbene resveratrol induces apoptosis in INS-1E rat insulinoma cells. Cell. Physiol. Biochem. 2009;23:245-54. [PubMed ID: 19471092].
-
9.
Santandreu FM, Valle A, Oliver J, Roca P. Resveratrol potentiates the cytotoxic oxidative stress induced by chemotherapy in human colon cancer cells. Cell. Physiol. Biochem. 2011;28:219-28. [PubMed ID: 21865729].
-
10.
Bayram D, Ozgocmen M, Armagan I, Sevimli M, Türel GY, Şenol N. Investigation of apoptotic effect of juglone on CCL-228-SW 480 colon cancer cell line. J. Cancer Res. Ther. 2019;15:68-74. [PubMed ID: 30880757].
-
11.
Fang F, Chen S, Ma J, Cui J, Li Q, Meng G, Wang L. Juglone suppresses epithelial-mesenchymal transition in prostate cancer cells via the protein kinase B/glycogensynthase kinase-3β/Snail signalling pathway. Oncol. Lett. 2018;16:2579-84. [PubMed ID: 30013652].
-
12.
Yousef M, Vlachogiannis IA, Tsiani E. Effects of resveratrol against lung cancer: in-vitro and in-vivo studies. Nutrients. 2017;9:E1231. [PubMed ID: 29125563].
-
13.
Rauf A, Imran M, Suleria HAR, Ahmad B, Peters DG, Mubarak MS. A comprehensive review of the health perspectives of resveratrol. Food Funct. 2017;8:4284-305. [PubMed ID: 29044265].
-
14.
Kumar S, Eroglu E, Stokes JA 3rd, Scissum-Gunn K, Saldanha SN, Singh UP, Manne U, Ponnazhagan S, Mishra MK. Resveratrol induces mitochondria-mediated, caspase-independent apoptosis in murine prostate cancer cells. Oncotarget. 2017;8:20895-908. [PubMed ID: 28157696].
-
15.
Zhao H, Han L, Jian Y, Ma Y, Yan W, Chen X, Xu H, Li L. Resveratrol induces apoptosis in human melanoma cells through negatively regulating Erk/PKM2/Bcl-2 axis. Onco. Targets Ther. 2018;11:8995-9006. [PubMed ID: 30588012].
-
16.
Luo H, Yang A, Schulte BA, Wargovich MJ, Wang GY. Resveratrol induces premature senescence in lung cancer cells via ROS-mediated DNA damage. PLoS One. 2013;8:e60065. [PubMed ID: 23533664].
-
17.
Velioğlu-Oğünç A, Sehirli O, Toklu HZ, Ozyurt H, Mayadağli A, Ekşioğlu-Demiralp E, Erzik C, Cetinel S, Yeğen BC, Sener G. Resveratrol protects against irradiation-induced hepatic and ileal damage via its anti-oxidative activity. Free Radic. Res. 2009;43:1060-71. [PubMed ID: 19707923].
-
18.
Avci E, Arikoglu H, Erkoc-Kaya D. Investigation of juglone effects on metastasis and angiogenesis in pancreatic cancer cells. Gene. 2016;588:74-8. [PubMed ID: 27155528].
-
19.
Hu YG, Shen YF, Li Y. Effect of Pin1 inhibitor juglone on proliferation, migration and angiogenic ability of breast cancer cell line MCF7Adr. J. Huazhong Univ. Sci. Technolog. Med. Sci. 2015;35:531-4. [PubMed ID: 26223922].
-
20.
Jha BK, Jung HJ, Seo I, Suh SI, Suh MH, Baek WK. Juglone induces cell death of Acanthamoeba through increased production of reactive oxygen species. Exp. Parasitol. 2015;159:100-6. [PubMed ID: 26358271].
-
21.
Meskelevicius D, Sidlauskas K, Bagdonaviciute R, Liobikas J, Majiene D. Jugloneexerts cytotoxic, anti-proliferative and anti-invasive effects on glioblastoma multiforme in a cell culture model. Anticancer Agents Med. Chem. 2016;16:1190-7. [PubMed ID: 26845134].
-
22.
Ahmad T, Suzuki YJ. Juglone in oxidative stress and cell signalling. Antioxidants Basel). 2019;8:E91. [PubMed ID: 30959841].
-
23.
Jordens J, Janssens V, Longin S, Stevens I, Martens E, Bultynck G, Engelborghs Y, Lescrinier E, Waelkens E, Goris J, Van Hoof C. The protein phosphatase 2A phosphatase activator is a novel peptidyl-prolylcis/trans-isomerase. J. Biol. Chem. 2006;281:6349-57. [PubMed ID: 16380387].
-
24.
Esnault S, Braun RK, Shen ZJ, Xiang Z, Heninger E, Love RB, Sandor M, Malter JS. Pin1 modulates the type 1 immune response. PLoS One. 2007;2:e226. [PubMed ID: 17311089].
-
25.
Esnault S, Rosenthal LA, Shen ZJ, Sedgwick JB, Szakaly RJ, Sorkness RL, Malter JS. A critical role for Pin1 in allergic pulmonary eosinophilia in rats. J. Allergy Clin. Immunol. 2007;120:1082-8. [PubMed ID: 17720236].
-
26.
Fan G, Fan Y, Gupta N, Matsuura I, Liu F, Zhou XZ, Lu KP, Gélinas C. Peptidyl-prolylisomerase Pin1 markedly enhances the oncogenic activity of the rel proteins in the nuclear factor-kappa B family. Cancer Res. 2009;69:4589-97. [PubMed ID: 19458071].
-
27.
Kim G, Khanal P, Kim JY, Yun HJ, Lim SC, Shim JH, Choi HS. COT phosphorylates prolyl-isomerase Pin1 to promote tumorigenesis in breast cancer. Mol. Carcinog. 2015;54:440-8. [PubMed ID: 24265246].
-
28.
Nechama M, Uchida T, MorYosef-Levi I, Silver J, Naveh-Many T. The peptidyl-prolylisomerase Pin1 determines parathyroid hormone mRNA levels and stability in rat models of secondary hyperparathyroidism. J. Clin. Invest. 2009;119:3102-14. [PubMed ID: 19770516].
-
29.
Jeong HG, Pokharel YR, Lim SC, Hwang YP, Han EH, Yoon JH, Ahn SG, Lee KY, Kang KW. Novel role of Pin1 induction in type II collagen-mediated rheumatoid arthritis. J. Immunol. 2009;183:6689-97. [PubMed ID: 19846884].
-
30.
Liu X, Liang E, Song X, Du Z, Zhang Y, Zhao Y. Inhibition of Pin1 alleviates myocardial fibrosis and dysfunction in STZ-induced diabetic mice. Biochem. Biophys Res. Commun. 2016;479:109-15. [PubMed ID: 27634219].
-
31.
Wu D, Huang D, Li LL, Ni P, Li XX, Wang B, Han YN, Shao XQ, Zhao D, Chu WF, Li BY. TGF-β1-PML SUMOylation-peptidyl-prolylcis-trans isomerase NIMA-interacting 1 (Pin1) form a positive feedback loop to regulate cardiac fibrosis. J. Cell. Physiol. 2019;234:6263-73. [PubMed ID: 30246389].
-
32.
Wu X, Li M, Chen SQ, Li S, Guo F. Pin1 facilitates isoproterenol-induced cardiac fibrosis and collagen deposition by promoting oxidative stress and activating the MEK1/2-ERK1/2 signal transduction pathway in rats. Int. J. Mol. Med. 2018;41:1573-83. [PubMed ID: 29286102].
-
33.
Xu HL, Yu XF, Qu SC, Zhang R, Qu XR, Chen YP, Ma XY, Sui DY. Anti-proliferative effect of Juglone from Juglansmandshurica Maxim on human leukemia cell HL-60 by inducing apoptosis through the mitochondria-dependent pathway. Eur. J. Pharmacol. 2010;645:14-22. [PubMed ID: 20655907].
-
34.
Ji YB, Xin GS, Qu ZY, Zou X, Yu M. Mechanism of juglone-induced apoptosis of MCF-7 cells by the mitochondrial pathway. Genet. Mol. Res. 2016:15.
-
35.
Powis G. Free radical formation by antitumor quinones. Free Radic. Biol. Med. 1989;6:63-101. [PubMed ID: 2492250].
-
36.
Liu X, Chen Y, Zhang Y, Du J, Lv Y, Mo S, Liu Y, Ding F, Wu J, Li J. Juglone potentiates TRAIL-induced apoptosis in human melanoma cells via activating the ROS/p38/p53 pathway. Mol. Med. Rep. 2017;16:9645-51. [PubMed ID: 29039537].
-
37.
Seminario-Amez M, López-López J, Estrugo-Devesa A, Ayuso-Montero R, Jané-Salas E. Probiotics and oral health: A systematic review. Med. Oral Patol. Oral Cir. Bucal. 2017;22:e282-88. [PubMed ID: 28390121].
-
38.
Dimidi E, Christodoulides S, Scott SM, Whelan K. Mechanisms of action of probiotics and the gastrointestinal microbiota on gut motility and constipation. Adv. Nutr. 2017;8:484-94. [PubMed ID: 28507013].
-
39.
Fiorda FA, de Melo Pereira GV, Thomaz-Soccol V, Rakshit SK, Pagnoncelli MGB, Vandenberghe LPS, Soccol CR. Microbiological, biochemical, and functional aspects of sugary kefir fermentation- A review. Food Microbiol. 2017;66:86-95. [PubMed ID: 28576377].
-
40.
Daniluk U. Probiotics, the new approach for cancer prevention and/or potentialization of anti-cancer treatment. J. Clin. Exp. Oncol. 2012;1:1.0E+108.
-
41.
Kumar M, Nagpal R, Verma V, Kumar A, Kaur N, Hemalatha R, Gautam SK, Singh B. Probiotic metabolites as epigenetic targets in the prevention of colon cancer. Nutr. Rev. 2013;71:23-34. [PubMed ID: 23282249].
-
42.
Ghoneum M, Gimzewski J. Apoptotic effect of a novel kefir product, PFT, on multidrug-resistant myeloid leukaemia cells via a hole-piercing mechanism. Int. J. Oncol. 2014;44:830-37. [PubMed ID: 24430613].
-
43.
Pektas MB, Turan Ö, Öztürk G, Şumlu E, Sadi G, Yıldırım OG, Akar F. High-glucose causes vascular dysfunction through Akt/eNOS pathway: Reciprocal modulation by juglone and resveratrol. Can. J. Physiol. Pharmacol. 2018;96:757-64. [PubMed ID: 29527953].
-
44.
Rakici O, Kiziltepe U, Coskun B, Aslamaci S, Akar F. Effects of resveratrol on vascular tone and endothelial function of human saphenous vein and internal mammary artery. Int. J. Cardiol. 2005;105:209-15. [PubMed ID: 16243115].
-
45.
Coskun B, Soylemez S, Parlar AI, TulgaUlus A, FehmiKatircioglu S, Akar F. Effect of resveratrol on nitrate tolerance in isolated human internal mammary artery. J. Cardiovasc. Pharmacol. 2006;47:437-45. [PubMed ID: 16633087].
-
46.
Chiasson VL, Munshi N, Chatterjee P, Young KJ, Mitchell BM. Pin1 deficiency causes endothelial dysfunction and hypertension. Hypertension. 2011;58:431-38. [PubMed ID: 21810655].
-
47.
Ruan L, Torres CM, Qian J, Chen F, Mintz JD, Stepp DW, Fulton D, Venema RC. Pin1 prolylisomerase regulates endothelial nitric oxide synthase. Arterioscler. Thromb. Vasc. Biol. 2011;31:392-8. [PubMed ID: 21051667].
-
48.
MacLimans WF, Davis EV, Glover FL, Rake GW. The submerged culture of mammalian cells: the spinner culture. J. Immunol. 1957;79:428-33. [PubMed ID: 13491853].
-
49.
Pektas MB, Koca HB, Sadi G, Akar F. Dietary fructose activates insulin signaling and inflammation in adipose tissue: Modulatory role of resveratrol. Biomed. Res. Int. 2016;2016:8014252. [PubMed ID: 27066503].
-
50.
Pelicano H, Carney D, Huang P. ROS stress in cancer cells and therapeutic implications. Drug Resist. Updat. 2004;7:97-110. [PubMed ID: 15158766].
-
51.
Gaikwad S, Chakraborty A, Salwe S, Patel V, Kulkarni S, Banerjee S. Juglone-ascorbic acid synergy inhibits metastasis and induces apoptotic cell death in poorly differentiated thyroid carcinoma by perturbing SOD and catalase activities. J. Biochem. Mol. Toxicol. 2018;32:e22176. [PubMed ID: 29992683].
-
52.
Wang D, Gao Z, Zhang X. Resveratrol induces apoptosis in murine prostate cancer cells via hypoxia-inducible factor1-alpha (HIF-1α)/reactive oxygen species (ROS)/P53 signalling. Med. Sci. Monit. 2018;24:8970-76. [PubMed ID: 30531685].
-
53.
Badr El-Din NK, Shabana SM, Abdulmajeed BA, Ghoneum M. A novel kefir product (PFT) inhibits Ehrlich ascites carcinoma in mice via induction of apoptosis and immunomodulation. BMC Complement Med. Ther. 2020;20:127. [PubMed ID: 32345289].
-
54.
Mamdooh G, James G. Apoptotic effect of a novel kefir product, PFT, on multidrug-resistant myeloid leukemia cells via a hole-piercing mechanism. Int. J. Oncol. 2014;44:830-37. [PubMed ID: 24430613].
-
55.
Kale J, Osterlund EJ, Andrews DW. BCL-2 family proteins: changing partners in the dance towards death. Cell Death Differ. 2018;25:65-80. [PubMed ID: 29149100].
-
56.
Saha T, Kar RK, Sa G. Structural and sequential context of p53: A review of experimental and theoretical evidence. Prog. Biophys Mol. Biol. 2015;117:250-63. [PubMed ID: 25550083].
-
57.
He W, Wang Q, Xu J, Xu X, Padilla MT, Ren G, Gou X, Lin Y. Attenuation of TNFSF10/TRAIL induced apoptosis by an autophagic survival pathway involving TRAF2 and RIPK1/RIP1 mediated MAPK8/JNK activation. Autophagy. 2012;8:1811-21. [PubMed ID: 23051914].
-
58.
You M, Savaraj N, Kuo MT, Wangpaichitr M, Varona-Santos J, Wu C, Nguyen DM, Feun L. TRAIL induces autophagic protein cleavage through caspase activation in melanoma cell lines under arginine deprivation. Mol. Cell. Biochem. 2013;374:181-90. [PubMed ID: 23180246].
-
59.
Shakeri R, Kheirollahi A, Davoodi J. Apaf-1: Regulation and function in cell death. Biochimie. 2017;135:111-25. [PubMed ID: 28192157].
-
60.
Mirzapur P, Khazaei MR, Moradi MT, Khazaei M. Apoptosis induction in human breast cancer cell lines by the synergic effect of raloxifene and resveratrol through increasing proapoptotic genes. Life Sci. 2018;205:45-53. [PubMed ID: 29705353].
-
61.
El-Azab M, Hishe H, Moustafa Y, El-Awady el-S. Anti-angiogenic effect of resveratrol or curcumin in Ehrlich Ascites Carcinoma-bearing mice. Eur. J. Pharmacol. 2011;652(1-3):7-14. [PubMed ID: 21114990].
-
62.
Ourique F, Kviecinski MR, Felipe KB, Correia JF, Farias MS, Castro LS, Grinevicius VM, Valderrama J, Rios D, Benites J, Calderon PB, Pedrosa RC. DNA damage and inhibition of akt pathway in mcf-7 cells and Ehrlich tumor in mice treated with 1,4-naphthoquinones in combination with ascorbate. Oxid. Med. Cell. Longev. 2015;2015:495305. [PubMed ID: 25793019].
-
63.
Jin X, Zhang Y, Zhang Z, Che D, Lv H. Juglone loaded poloxamer188/phospholipid mixed micelles evaluated in-vitro and in-vivo in breast cancer. Int. J. Pharm. 2016;515:359-66. [PubMed ID: 27744033].