Abstract
Keywords
Introduction
Absence epilepsy is considered as non-convulsive epilepsy that is characterized by a sudden loss of consciousness and is associated with generalized spontaneous and synchronous bilateral spike-wave discharges (SWDs) in the electroencephalogram (1).
It has been reported that the intra-thalamic neuronal networks originate spike-waves discharges in the absence epilepsy (2, 3). The role of the somatosensory neocortex to trigger and generation of SWDs has been reported (4-7).
The triggering role of the somatosensory cortex has been identified in the SWDs initiation in the Wistar Albino Glaxo from Rijswijk (WAG/Rij) rats (8). Analysis of cortical and thalamic electroencephalographic recordings from WAG/Rij rat, a valid and naturally occurring genetic model of absence epilepsy revealed that SWDs were initiated in the primary somatosensory cortex and then rapidly propagated to motor cortices and thalamic nuclei (9).
The somatosensory cortex is typically organized in six layers, beginning with layer I nearby the brain surface and extending progressively deeper to layer VI. The incoming sensory signals first enter the neuronal layer IV, and then propagate to the surface of the cortex and deeper layers. The processed signals are sent to the thalamus from layer VI. These signals help to adjust the excitation of thalamic nuclei (10, 11).
The ictogenic neurons in deep layers of the somatosensory cortex display fast activation, hyperexcitability and hyper synchronization in the absence seizures (4, 5).
The α-amino-3-hydroxy-5-methyl-4-isoxazole propionic acid receptors (AMPARs) modulate cortical excitability (12). These receptors are a subtype of the ionotropic glutamate receptor with intrinsic cation permeable channels and mediate fast synaptic transmission in the central nervous system (CNS). AMPARs are abundant and distributed widely in the CNS, although there are regional density differences (13). Four subtypes were designated as GluR1, GluR2, GluR3, and GluR4 for AMPARs (14). The GluR1 and GluR3 subunits show a significant Ca2+ conductance, whereas the GluR2 subunit is impermeable to Ca2+ (15).
The role of AMPARs have been determined in the pathogenesis of many neurological and neurodegenerative disorders, such as biological manifestations of dementia in Alzheimer’s disease or neuronal death in the amyotrophic lateral sclerosis and stroke (16).
Furthermore, AMPARs play a key role in the synchronization, generation and spread of epileptic discharges in the cortex, amygdala, and thalamus (17, 18).
Since the GluR1 subunit of AMPA receptors shows a significant Ca2+ conductance, and given the fact that the main evoking event in absence epilepsy is rise of intracellular calcium ions in brain regions related to epilepsy, in this study we assessed the alternation of GluR1 expression in the deep layers of somatosensory cortex during development of absence seizures as well as modulatory effect of AMPARs on the SWDs features.
Experimental
Animals
Male WAG/Rij and Wistar rats were housed under conditions with temperature 22 ± 2 °C, 12-h light/dark cycle and free access to food and water. All experiments were performed in accordance with the guidelines approved by the animal ethics of Shefa Neuroscience Research Center, Tehran, Iran. The animals were divided into four groups of both strains with 2 and 6 months of age (n = 6).
Electrocorticoencephalogram (ECoG) Recordings
To select epileptic animals, ECoG was recorded at least for 6 hours. All the animals were anesthetized by intraperitoneal chloral hydrate injection (350 mg/kg; Sigma–Aldrich, Munich, Germany) and recording electrodes were implanted stereotaxically on the dura mater of parietal cortices. Silver electrodes connected to an amplifier (EXT-02 F; NPI, Germany) and ECoG were stored by a digital oscilloscope. Sedation was retained by repeated injections of fentanyl every 20-30 min (0.033 mg/kg per hour, i.p.; (11, 19)). At the age of 6 months, WAG/Rij rats exhibited most SWDs discharges and were included in the study as epileptic rats (9). Most of the two-month-old WAG/Rij rats (before adulthood) did not display SWDs and have been considered as a pre-symptomatic group.
Immunofluorescent staining
After deep anesthesia with 350 mg/kg chloral hydrate (Sigma–Aldrich) and transcardial perfusion of 200 mL of saline and then 400 mL of 4% paraformaldehyde solution (PFA), the brains were removed and inserted in the 4% PFA for 1 week at 4 °C.
Being followed by preparing paraffin-embedded blocks, the serial coronal sections in AP = 1.8–3.3 mm posterior to the bregma (Paxinos and Watson, 1998) with 8μm thickness were cut by microtome (20). Five slices from each animal were selected for immunofluorescent staining.
The sections were rehydrated through series of xylol and alcohol and washed with phosphate-buffered saline (PBS; pH 7.4) three times and incubated in blockage solution (3% H2O2/ methanol for 5 min). The sections were boiled in citrate buffer (pH 6.0) at 95 °C for 10 min and were cooled at room temperature. The sections were incubated in 10% normal goat serum and 0.2% Triton-X100 for 1 h. The sections were incubated overnight at 4 °C with mouse monoclonal anti-rat antibody (sc-55509, Santa Cruz) against GluR1 overnight, diluted at 1:350 in a solution comprising 1–5% NGS in 0.3% Triton X-100 and 0.1 M PBS at pH of 7.4. The sections were rinsed in PBS three times and were incubated with FITC goat anti-mouse secondary antibody (sc-2010, Santa Cruz). Secondary antibody concentration was 1:100 in PBS with 0.3% Triton X-100 and 5% NGS at 22 °C for 1 h. After several times washing with PBS, nuclei were counterstained by 5 min at 37 °C submerging sections in a propidium iodide solution (75 μg/mL). The sections were mounted on a coverslip with glycerol 90% mounting buffer and were observed with a fluorescent microscope (Olympus, Japan). Control for the specificity of immunostaining was performed by the omission of the primary antibody and its substitution for normal mouse serum. Images (five visual field / section) for analysis were taken with a digital camera attached to the fluorescent microscope (40× objective). The number of cells reacted with the GluR1 antibody was counted per 1 mm2 in the IV and VI layers of the somatosensory cortex by using ImageJ software.
Drugs Application Procedures
Experiments were performed on adult 6-month-old WAG/Rij (epileptic) rats. The animals were divided into three groups: vehicle, agonist, and antagonist (n = 6). The procedures of electrode implementation and ECoG recording have been mentioned above. A hole was created on the skull bone (AP = -2.5 mm, ML = ±4.3 mm) according to the coordinates of the rat brain atlas by Paxinos and Watson (1998) for local drug infusion on the somatosensory cortex.
ECoG was recorded for 30 min as a baseline activity. Selective AMPA agonist (Rs-AMPA hydrobromide; Tocris, Cat. No. 1074) was injected in the agonist group. Selective non-NMDA (N-Methyl-D-aspartate) ionotropic glutamate receptors antagonist (DNQX, Tocris, Cat. No. 2312) was injected in the antagonist group. Distilled water was injected in the vehicle group. The injections were performed by using a 10-gaged Hamilton syringe on the primary somatosensory cortex (21). The drugs were dissolved in distilled water and microinjected in a concentration of 0.5 μg/0.5 μL for 2 min (22, 23).
ECoG monitoring was continued for 2 h followed by drug infusion. By using the AxoScope 10 software (Axon Instruments, USA) the amplitude, frequency, and duration of SWDs were analyzed.
Statistical Analysis
Data were expressed as Mean ± SEM. The immunohistochemical statistical analysis was carried out by one-way analysis of variance (ANOVA) followed by Tukey’s post hoc test. The frequency, amplitude, and duration of SWDs were statistically analyzed before and after usage of drugs by Friedman and Wilcoxon test. The significant point was considered p < 0.05. The PASW Statistics 18 was applied for statistical analysis.
Results
Cortical distribution of GluR1
The data are presented as the mean number of reacted neurons/mm2 (Figure 1A). GluR1 highly was expressed in the 6-month-old WAG/Rij rats compared to 2-month-old rats of the same race both in IV layer (0.13 ± 0.01) and in VI layers (0.08 ± 0.001) of the somatosensory cortex (p < 0.001 and p < 0.01, respectively, Figure 1B). Furthermore, the distribution of GluR1 in the 6-month-old WAG/Rij was more than 6- and 2-month-old Wistar rats in both layers of cortex (p < 0.001, Figure 1B). The mean number of reacted neurons was 0.026 ± 0.003 in layer IV and 0.046 ± 0.003 in layer VI of the 2-month-old Wistar rats. The mean number of reacted neurons was 0.023 ± 0.003 in layer IV and 0.026 ± 0.006 in the layer VI of 6-month-old Wistar rats.
There was no significant difference between 6- and 2-month- old Wistar rats in both layers of the somatosensory cortex (Figure 1B, p = 0.9).
AMPARs modulatory effects on the absence seizures
Six-month-old WAG/Rij rats were monitored under neuroleptic anesthesia during the recording of ECoG. The bilaterally synchronized SWDs at 5–9 Hz on the ECoG were started and ended abruptly on a normal background pattern. The agonist and antagonist of AMPA receptors were microinjected unilaterally on the somatosensory cortex (Figure 2).
As we have shown in Figure 2B, the amplitude of SWDs increased (0.57 ± 0.05 mV) significantly after the microinjection of AMPARs agonist (p < 0.01), while, the amplitude of SWDs decreased significantly after the microinjection of the AMPARs antagonist (p < 0.001). The mean amplitude of SWDs was 0.61 ± 0.03 mV before the injection of antagonist and 1.2 ± 0.11 mV after injection. In 2 hours after injection (recovery time) the amplitude of SWDs in agonist (p < 0.05) and antagonist (p < 0.01) groups has changed significantly compared to injection time (Figure 2B).
In addition, the duration of SWDs significantly increased (3.6 ± 0.31 s) after the application of AMPARs agonist (p < 0.001) while, following the antagonist infusion, the duration of SWDs significantly decreased (1.2 ± 0.11 S, p < 0.01) compared to before and after (recovery time) drug administration (Figure 2C). In recovery time the duration of SWDs in agonist (p < 0.001) and antagonist (p < 0.05) groups has changed significantly compared to injection time (Figure 2B).
There was no significant difference in the frequency of SWDs following the agonist and antagonist infusion.
Discussion
Cortical dis excitation developed absence seizures
Developmental alternations in the cortical excitation and/or inhibition are involved in the pathophysiology of some neurodevelopmental disorders (24, 25). Cortical disinhibition has been indicated in the pathogenesis of Parkinson’s disease (26). Cortical enhancement of excitability has a key role in the pathophysiology mechanism of Alzheimer’s disease. Further, cortical excitability might lead to cortical dysfunction in the primary lateral sclerosis as well as amyotrophic lateral sclerosis (27, 28). It has been reported that the fluctuation of cortical excitation underlies hemiplegic attacks (29). High resting, active motor threshold and small evoked potentials in patients with Sydenham’s chorea emphasized the low excitability of corticospinal output (30).
Epilepsy as a neurodevelopment disorder has been investigated for cortical anomalies.
We evaluated the changes of the GluR1 subunit in the somatosensory cortex during the development of absence epilepsy.
WAG/Rij rats display SWDs in adulthood and are considered as a valid genetic model of gene-linked absence epilepsy (9). Our findings indicated that the GluR1 subunit in the somatosensory cortex, in both IV and VI cortical layers, has been highly expressed in the adult epileptic rats. On the contrary, it has been down-regulated in the adult healthy Wistar rats.
GluR1 as a Ca2+ permeable subunit of AMPARs is important for normal synaptic function after birth. In early developmental stages, many synapses contain GluR1, start to change into GluR2 (Ca2+ impermeable subunit of AMPARs) during the age-dependent development of cortical layers (31, 32).
Abnormalities of structure and function of synapses during the development of CNS are involved in the pathogenesis of absence seizures (25). Prior studies have reported high expression of AMPA receptors in the hippocampus and cerebral cortex in the epileptic people and rats (33-35). In the hippocampus of epileptic people, the density of AMPA GluR1 subunit proteins has been increased (36). GluR2 markedly has been down-regulated in the neurons that were subject to epileptic seizures (37). Further investigations documented that enhancement of hippocampal GluR1 followed by some neuropathological conditions such as inflammation (38) or impaired autophagy, reduced GluR1 degradation and increased seizure susceptibility in the adult rats (39, 40). Functional and single-cell transcript analyses have shown that prolonged receptor responses in the hippocampal astrocytes of epileptic patients, resulted from high expression of GluR1 in this region (41-43).
These data suggest that aberrant patterns of physiological activities influence the composition of AMPA receptor subunits in a region-specific and/or cell-type-specific manner.
Synaptic activity is needed for synaptic delivery AMPA receptors containing the GluR1 subunit, whereas GluR2 is constitutively inserted into synapses (44). It is previously was shown that the expression of the stargazin, a critical regulators of the trafficking and function of AMPA receptors, increased in the somatosensory cortex of genetic absence epilepsy rat from Strasbourg (GAERS) (45); and its elevation is accompanied with an increase in AMPA receptor proteins (GluR1 and GluR2) in the somatosensory cortex of adult epileptic GAERS (46). It seems that AMPA receptor subunits in different neuronal populations are subject to distinct regulatory processes in response to intense physiological activity.
Ictogenic neurons of deep layers of somatosensory cortex display distinctive hyperactivity that leads to these data suggesting that aberrant patterns of physiological activities influence the composition of AMPA receptor subunits in a region-specific and/or cell-type-specific manner.
Synaptic activity is needed for synaptic delivery AMPA receptors containing the GluR1 subunit, whereas GluR2 is constitutively inserted into synapses (44). It is previously was shown that the expression of the stargazin, a critical regulator of the trafficking and function of AMPA receptors, increased in the somatosensory cortex of genetic absence epilepsy rat from Strasbourg (GAERS) (45); and its elevation is accompanied with an increase in AMPA receptor proteins (GluR1 and GluR2) in the somatosensory cortex of adult epileptic GAERS (46). It seems that AMPA receptor subunits in different neuronal populations are subject to distinct regulatory processes in response to intense physiological activity.
Ictogenic neurons of deep layers of somatosensory cortex display distinctive hyperactivity that leads to the firing of distant cortical and thalamic cells during the epileptic discharges (5, 47 and 48). Based on these data we conclude that aberrant cortical expression of GluR1 receptors in epileptic rats may contribute to the development of SWDs.
AMPARs modulatory effects on the absence seizures
We assessed the effect of pharmacological modulation of AMPARs on the characteristic of bioelectrical brain activities in the somatosensory cortex of epileptic rats and showed that cortical activation of AMPARs exacerbated the absence seizures in the epileptic WAG/Rij rats.
Various pathophysiological mechanisms are involved in the abnormal neuronal discharges in epilepsy. Regardless of the primary cause, abnormal glutamate (excitatory) neurotransmission, affecting on metabotropic and ionotropic receptors, is was thought to be critical for seizure generation and the epileptic state (21, 49 and 50).
Throughout the CNS, fast synaptic excitation within and between brain regions related to epilepsy is mediated mostly by AMPA and NMDA receptor localized to the postsynaptic membrane. It has been shown that activation of AMPA receptors can evoke seizures in the preclinical models (51). Based on previous studies, selective blockade of NMDA receptor alone is not sufficient to eliminate epileptiform discharges and AMPA receptors play a key role in modulating excitatory synaptic transmission in epilepsy (52, 53).
In addition, we showed that inactivation of AMPARs in the somatosensory cortex suppressed seizure activities in the absence epileptic rats.
The anti-epileptic effect of AMPA receptor antagonists has been reported. These components noticeably reduce or suppress epileptiform activity by inhibiting glutamate-mediated excitation. It was shown in in-vitro (54-57) as well as in-vivo animal models of seizure that AMPA receptor antagonists markedly reduce or abolish epileptiform activity (17, 58).
Our findings clarify the potential therapeutic role of AMPARs for absence seizures and in the modulation of cortical neural hyperactivity.
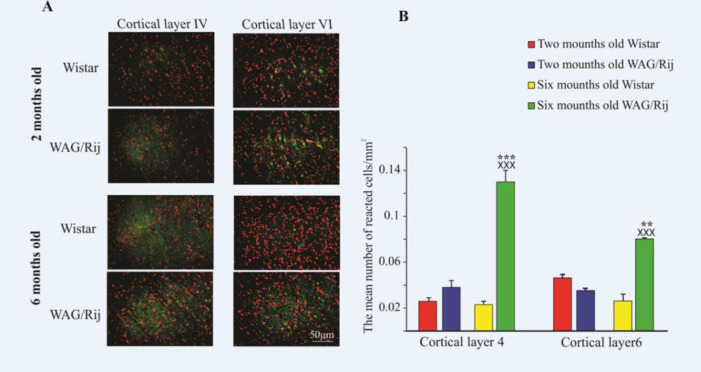
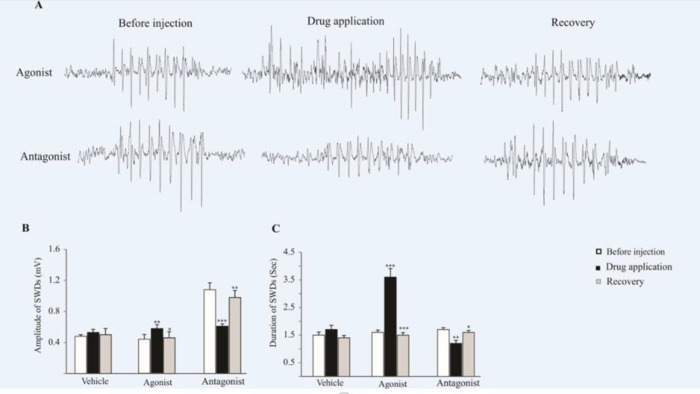
Acknowledgements
References
-
1.
Bilo L, Pappatà S, De Simone R, Meo R. The syndrome of absence status epilepsy: review of the literature. Epilepsy Res. treat. 2014;2014:320-8.
-
2.
Bal T, von Krosigk M, McCormick DA. Role of the ferret perigeniculate nucleus in the generation of synchronized oscillations in-vitro. J. Physiol. 1995;483:665-85. [PubMed ID: 7776250].
-
3.
Avanzini G, Panzica F, De Curtis M. The role of the thalamus in vigilance and epileptogenic mechanisms. Clin. Neurophysiol. 2000;111:219-26.
-
4.
Polack PO, Charpier S. Intracellular activity of cortical and thalamic neurones during high-voltage rhythmic spike discharge in Long-Evans rats in-vivo. J.Physiol. 2006;571:461-76. [PubMed ID: 16410284].
-
5.
Depaulis A, Charpier S. Pathophysiology of absence epilepsy: Insights from genetic models. Neurosci. Lett. 2018;667:53-65. [PubMed ID: 28216336].
-
6.
Seneviratne U, Cook M, D’souza W. Focal abnormalities in idiopathic generalized epilepsy: a critical review of the literature. Epilepsia. 2014;55:1157-69. [PubMed ID: 24938654].
-
7.
Polack PO, Mahon S, Chavez M, Charpier S. Inactivation of the somatosensory cortex prevents paroxysmal oscillations in cortical and related thalamic neurons in a genetic model of absence epilepsy. Cereb. Cortex. 2009;19:2078-91. [PubMed ID: 19276326].
-
8.
Russo E, Citraro R, Constanti A, Leo A, Lüttjohann A, van Luijtelaar, G De Sarro. Upholding WAG/Rij rats as a model of absence epileptogenesis: hidden mechanisms and a new theory on seizure development. Neurosci. Biobehav. Rev. 2016;71:388-408. [PubMed ID: 27687816].
-
9.
Coenen A, Van Luijtelaar E. Genetic animal models for absence epilepsy: a review of the WAG/Rij strain of rats. Behav. Genet. 2003;33:635-55. [PubMed ID: 14574120].
-
10.
Hall JE. Guyton and Hall, Textbook of Medical Physiology e-Book. 13nd ed. Elsevier Health Sciences. Philadelphia. 2015:736.
-
11.
Karimzadeh F, Mousavi SMM, Ghadiri T, Jafarian M, Soleimani M, Sadeghi Sh, Mesgari M, Joghataei M, Gorji A. The modulatory effect of metabotropic glutamate Receptor Type-1α on spike-wave discharges in WAG/Rij rats. Mol. Neurobiol. 2016;54:846-54. [PubMed ID: 26780454].
-
12.
Man HY, Sekine-Aizawa Y, Huganir RL. Regulation of α-amino-3-hydroxy-5-methyl-4-isoxazolepropionic acid receptor trafficking through PKA phosphorylation of the Glu receptor 1 subunit. Proc. Natl. Acad. Sci. 2007;104:3579-84. [PubMed ID: 17360685].
-
13.
Platt SR. The role of glutamate in central nervous system health and disease–a review. Vet. J. 2007;173:278-86. [PubMed ID: 16376594].
-
14.
Greger IH, Ziff EB, Penn AC. Molecular determinants of AMPA receptor subunit assembly. Trends Neurosci. 2007;30:407-16. [PubMed ID: 17629578].
-
15.
Meldrum BS. Glutamate as a neurotransmitter in the brain: review of physiology and pathology. Nutr. J. 2000;130:1007S-15S.
-
16.
Weiser T. AMPA receptor antagonists for the treatment of stroke. CNS Neurol. Disord. DrugTargets. 2005;4:153-9.
-
17.
Rogawski MA. AMPA receptors as a molecular target in epilepsy therapy. Acta Neurol.Scand. 2013;127:9-18.
-
18.
Beneyto M, Meador-Woodruff JH. Expression of transcripts encoding AMPA receptor subunits and associated postsynaptic proteins in the macaque brain. J. Comp. Neurol. 2004;468:530-54. [PubMed ID: 14689485].
-
19.
Karimzadeh F, Mousavi SMM, Alipour F, Ravandi HH, Kovac S, Gorji A. Developmental changes in Notch1 and NLE1 expression in a genetic model of absence epilepsy. Brain Struct. Funct. 2017;222:2773-85. [PubMed ID: 28210849].
-
20.
Paxinos G, Watson C. The Rat Brain Atlas in Stereotaxic Coordinates. 2nd ed. Academic, San Diego. 1998:63.
-
21.
Citraro R, Russo E, Gratteri S, Di Paola ED, Ibbadu GF, Curinga C, Gitto R, Chimirri A, Donato G, De Sarro. Effects of non-competitive AMPA receptor antagonists injected into some brain areas of WAG/Rij rats, an animal model of generalized absence epilepsy. Neuropharmacol. 2006;51:1058-67.
-
22.
Ghalandari-Shamami M, Hassanpour-Ezatti M, Haghparast A. Intra-accumbal NMDA but not AMPA/kainate receptor antagonist attenuates WIN55, 212-2 cannabinoid receptor agonist-induced antinociception in the basolateral amygdala in a rat model of acute pain. Pharmacol. Biochem. Behav. 2011;100:213-29. [PubMed ID: 21924283].
-
23.
Fan X, Hughes KE, Jinnah H, Hess EJ. Selective and sustained α-amino-3-hydroxy-5-methyl-4-isoxazolepropionic acid receptor activation in cerebellum induces dystonia in mice. J. Pharmacol. Exp. Ther. 2012;340:733-41. [PubMed ID: 22171094].
-
24.
Mehrabi S, Sanadgol N, Barati M, Shahbazi A, Vahabzadeh G, Barzroudi M, Seifi M. Evaluation of metformin effects in the chronic phase of spontaneous seizures in pilocarpine model of temporal lobe epilepsy. Metab. Brain Dis. 2018;33:107-14. [PubMed ID: 29080083].
-
25.
Karimzadeh F, Soleimani M, Mehdizadeh M, Jafarian M, Mohamadpour M, Kazemi H, Gorji A. Diminution of the NMDA receptor NR2B subunit in cortical and subcortical areas of WAG/Rij rats. Synapse. 2013;67:839-46. [PubMed ID: 23754322].
-
26.
Ridding M, Rothwell J, Inzelberg R. Changes in excitability of motor cortical circuitry in patients with Parkinson’s disease. Ann. Neurol. 1995;37:181-208. [PubMed ID: 7847860].
-
27.
Geevasinga N, Menon P, Sue C, Kumar K, Ng K, Yiannikas C, Kiernan MC. Cortical excitability changes distinguish the motor neuron disease phenotypes from hereditary spastic paraplegia. Eur. J. Neurol. 2015;22:826-58. [PubMed ID: 25683471].
-
28.
Menon P, Geevasinga N, Van Den Bos M, Yiannikas C, Kiernan M, Vucic S. Cortical hyperexcitability and disease spread in amyotrophic lateral sclerosis. Eur. J. Neurol. 2017;24:816-24. [PubMed ID: 28436181].
-
29.
Stern WM, Desikan M, Hoad D, Jaffer F, Strigaro G, Sander JW, Rothwell JC. Spontaneously fluctuating motor cortex excitability in alternating hemiplegia of childhood: a transcranial magnetic stimulation study. PLoS One. 2016;11:149-67.
-
30.
Khedr EM, Ahmed MA, Ali AM, Badry R, Rothwell JC. Changes in motor cortical excitability in patients with Sydenham’s chorea. Mov. Disord. 2015;30:259-62. [PubMed ID: 24909435].
-
31.
Pickard L, Noel J, Henley JM, Collingridge GL, Molnar E. Developmental changes in synaptic AMPA and NMDA receptor distribution and AMPA receptor subunit composition in living hippocampal neurons. J. Neurosci. 2000;20:7922-31. [PubMed ID: 11050112].
-
32.
Henley JM, Wilkinson KA. Synaptic AMPA receptor composition in development, plasticity and disease. Nat. Rev. Neurosci. 2016;17:337-49. [PubMed ID: 27080385].
-
33.
Babb TL, Mathern GW, Leite JP, Pretorius JK, Yeoman KM, Kuhlman PA. Glutamate AMPA receptors in the fascia dentata of human and kainate rat hippocampal epilepsy. Epilepsy Res. 1996;26:193-205. [PubMed ID: 8985700].
-
34.
Hosford DA, Crain BJ, Cao Z, Bonhaus DW, Friedman AH, Okazaki M, Nadler J. Increased AMPA-sensitive quisqualate receptor binding and reduced NMDA receptor binding in epileptic human hippocampus. J. Neurosci. 1991;11:428-34. [PubMed ID: 1846907].
-
35.
Sherwin AL. Neuroactive amino acids in focally epileptic human brain: a review. Neurochem. Res. 1999;24:1385-95.
-
36.
Ying Z, Babb TL, Comair YG, Bushey M, Touhalisky K. Increased densities of AMPA GluR1 subunit proteins and presynaptic mossy fiber sprouting in the fascia dentata of human hippocampal epilepsy. Brain Res. 1998;798:239-46. [PubMed ID: 9666139].
-
37.
Grooms SY, Opitz T, Bennett MV, Zukin RS. Status epilepticus decreases glutamate receptor 2 mRNA and protein expression in hippocampal pyramidal cells before neuronal death. Proc. Natl. Acad. Sci. 2000;97:3631-6. [PubMed ID: 10725374].
-
38.
Galic M, Riazi K, Henderson A, Tsutsui S, Pittman Q. Viral-like brain inflammation during development causes increased seizure susceptibility in adult rats. Neurobiol. Dis. 2009;36:343-51. [PubMed ID: 19660546].
-
39.
Shehata M, Matsumura H, Okubo-Suzuki R, Ohkawa N, Inokuchi K. Neuronal stimulation induces autophagy in hippocampal neurons that is involved in AMPA receptor degradation after chemical long-term depression. J. Neurosci. 2012;32:10413-22. [PubMed ID: 22836274].
-
40.
Bakker J, Basedow FJ, Dekker AD, Papantoniou C. Phosphorylation of AMPA-type glutamate receptors: the trigger of epileptogenesis? J. Neurosci. 2013;33:5879-80.
-
41.
Seifert G, Carmignoto G, Steinhäuser C. Astrocyte dysfunction in epilepsy. Brain Res.Rev. 2010;63:212-21. [PubMed ID: 19883685].
-
42.
Seifert G, Schröder W, Hinterkeuser S, Schumacher T, Schramm J, Steinhäuser C. Changes in flip/flop splicing of astroglial AMPA receptors in human temporal lobe epilepsy. Epilepsia. 2002;43:162-7. [PubMed ID: 12121314].
-
43.
Seiffert E, Dreier JP, Ivens S, Bechmann I, Tomkins O, Heinemann U, Friedman A. Lasting blood-brain barrier disruption induces epileptic focus in the rat somatosensory cortex. J. Neurosci. 2004;24:7829-36. [PubMed ID: 15356194].
-
44.
Bredt DS, Nicoll RA. AMPA receptor trafficking at excitatory synapses. Neuron. 2003;40:361-79. [PubMed ID: 14556714].
-
45.
Powell KL, Kyi M, Reid CA, Paradiso L, D’Abaco G, Kaye A, Foote S. Genetic absence epilepsy rats from Strasbourg have increased corticothalamic expression of stargazin. Neurobiol. Dis. 2008;31:261-5. [PubMed ID: 18556211].
-
46.
Kennard J, Barmanray R, Sampurno S, Ozturk E, Reid C, Paradiso L, D’Abaco GM. Stargazin and AMPA receptor membrane expression is increased in the somatosensory cortex of genetic absence epilepsy rats from strasbourg. Neurobiol. Dis. 2011;42:48-54. [PubMed ID: 21220022].
-
47.
Sadleir L, Farrell K, Smith S, Connolly M, Scheffer I. Electroclinical features of absence seizures in childhood absence epilepsy. Neurology. 2006;67:413-8. [PubMed ID: 16894100].
-
48.
Polack PO, Guillemain I, Hu E, Deransart C, Depaulis A, Charpier S. Deep layer somatosensory cortical neurons initiate spike-and-wave discharges in a genetic model of absence seizures. J. Neurosci. 2007;27:6590-9. [PubMed ID: 17567820].
-
49.
Moldrich RX, Chapman AG, De Sarro G, Meldrum BS. Glutamate metabotropic receptors as targets for drug therapy in epilepsy. Eur. J. Pharmacol. 2003;476:3-16. [PubMed ID: 12969743].
-
50.
Çavuş I, Romanyshyn JC, Kennard JT, Farooque P, Williamson A, Eid T, Spencer S, Duckrow R, Dziura J. Elevated basal glutamate and unchanged glutamine and GABA in refractory epilepsy: Microdialysis study of 79 patients at the yale epilepsy surgery program. Ann. Neurol. 2016;80:35-45. [PubMed ID: 27129611].
-
51.
Meldrum BS, Rogawski MA. Molecular targets for antiepileptic drug development. Neurotherapeutics. 2007;4:18-61. [PubMed ID: 17199015].
-
52.
Lee HK, Takamiya K, He K, Song L, Huganir RL. Specific roles of AMPA receptor subunit GluR1 (GluA1) phosphorylation sites in regulating synaptic plasticity in the CA1 region of hippocampus. J. Neurophysiol. 2009;103:479-89. [PubMed ID: 19906877].
-
53.
Rogawski MA. Revisiting AMPA receptors as an antiepileptic drug target. Epilepsy Curr. 2011;11:56-63. [PubMed ID: 21686307].
-
54.
Koh S, Tibayan FD, Simpson JN, Jensen FE. NBQX or topiramate treatment after perinatal hypoxia-induced seizures prevents later increases in seizure-induced neuronal injury. Epilepsia. 2004;45:569-75. [PubMed ID: 15144420].
-
55.
McBain C, Boden P, Hill R. The kainate/quisqualate receptor antagonist, CNQX, blocks the fast component of spontaneous epileptiform activity in organotypic cultures of rat hippocampus. Neurosci. Lett. 1988;93:341-5. [PubMed ID: 2907375].
-
56.
Neuman R, Ben-Ari Y, Cherubini E. Antagonism of spontaneous and evoked bursts by 6-cyano-7-nitroquinoxaline-2, 3-dione (CNQX) in the CA3 region of the in-vitro hippocampus. Brain Res. 1988;474:201-3. [PubMed ID: 2905616].
-
57.
Lee WL, Hablitz JJ. Involvement of non-NMDA receptors in picrotoxin-induced epileptiform activity in the hippocampus. Neurosci. Lett. 1989;107:129-34. [PubMed ID: 2575723].
-
58.
Rakhade SN, Zhou C, Aujla PK, Fishman R, Sucher NJ, Jensen FE. Early alterations of AMPA receptors mediate synaptic potentiation induced by neonatal seizures. J. Neurosci. 2008;28:7979-90. [PubMed ID: 18685023].