Abstract
Keywords
Antimicrobial Diabetes Phenolics Potentilla erecta Wound healing
Introduction
Diabetes mellitus (DM) is a metabolic disease characterized by hyperglycemia resulting from abnormal insulin secretion and/or action (1). Impaired wound healing and chronic wounds are important complications of diabetes and cause severe problems, including social and economic. The underlying mechanism of the impaired healing process is not fully understood, but it is thought to be caused by neuropathy, peripheral vascular diseases, abnormal cellular activities, infection, and altered oxidative balance (2–4). Considering that DM affects hundreds of millions of people around the world, it can be said that treatment of impaired or non-healing wounds is very crucial.
Potentilla is a genus of the family Rosaceae that consists of about over 500 species of annual and perennial plants (5). Potentilla genus, the cinquefoils, are used in the traditional treatment of wounds, burns, bleeding, and stomach and digestive disorders in various countries (6–11). These plants are heavy in secondary metabolites and have anticonvulsant anticancer and antioxidant properties owing to their chemical structures (5). P. erecta L. is commonly used as herbal medicine and is called “Kurt pençesi” in Turkey (12). P. erecta has a spectacular rhizome, which is thick, woody, and red inside (13). Tannins, astringent polyphenols, are the major active pharmacological compounds (approx. 20%) of the rhizome, especially agrimoniin (13). Tomczyk and Latté reported that in the ethnobotanical review, the rhizome part of the P. erecta was used to treat wounds in various countries (5). Similarly, it has also been mentioned that in the books on herbal remedies, P. tormentilla (synonym of P. erecta) is traditionally used in wound and ulcer healing (14, 15).
The objective of this study was to compare the diabetic control group with the non-diabetic control group and then examine the time-dependent effects of the P. erecta methanol root extract (MEPE) on parameters affected by diabetes. For this purpose, wound area, wound contraction rate, and levels of collagen, TBARs, NOx, GSH on days 0, 3, and 7 were determined in circular excisional wound-induced rats, as well as detecting the phenolic, antioxidant and antimicrobial properties of MEPE.
Experimental
Plant Extract Preparation
Plant samples were collected from Bulancak/Giresun province in Turkey (40°42’18.5 “N, 38°03’15.2 “E) and were identified by Dr. Mustafa Karaköse. A voucher specimen has been deposited at the Herbarium of the Vocational School of Espiye, Giresun University, as ESPH 016. The collected samples root parts were separated and dried at room temperature. The dried, powdered root parts were extracted 100 mL of methanol for 8 h using Soxhlet apparatus. The extracts were then filtered using Whatman Millipore filter paper and the solvents were removed with a rotary evaporator. The obtained extract (MEPE) was divided into two parts. The first part was dissolved in pure water for use in antioxidant and antimicrobial assays. The remaining part was dissolved in methanol for use in reversed-phase high performance liquid chromatographic method (RP-HPLC) with UV detection.
Phenolic Compounds Determination
Phenolic compounds were determined by RP-HPLC-UV analysis. This analysis was performed via a binary solvent gradient system using a reversed-phase column (C18 HPLC (150 mm × 4.6 mm × 5 μm), Fortis, UK) with a Thermo Finnigan Surveyor HPLC (Thermo Scientific, USA) (A: 2% acetic acid-distilled water, B: 70% acetonitrile-distilled water, initial condition 5% B and it was increased to 80% B in 50 min). The analysis conditions were set at a column temperature of 30 °C, a mobile phase flow rate of 1.2 mL/min and an injection volume of 10 μL. The eluted 17 standard phenolic compounds (gallic acid, protocatechuic acid, chlorogenic acid, p-OH benzoic acid, caffeic acid, vanillic acid, syringic acid, ellagic acid, rutin, p-coumaric acid, ferulic acid, myricetin, fisetin, quercetin, apigenin, kaempferol, and isorhamnetin) were detected by comparison at 280 and 315 nm.
Antioxidant Activity Determination
The total phenolic content (TPC) of the root extracts was determined according to the Folin-Ciocalteu method using gallic acid standard (16). Firstly, 680 mL purified water, 20 mL stock solution (extract), and 400 mL 0.5 mol/L Folin-Ciocalteu reactive were mixed in a tube. After incubation for 10 min at room temperature, 400 mL Na2CO3 (10%) was added and this mixture was incubated for 120 min at room temperature. After incubation, samples were measured at 760 nm. The activities were given as mg GAE equivalents/g extract. The ferric reducing antioxidant power assay (FRAP) was assayed according to the method of Benzie and Strain (17). The FRAP assay, which is a procedure for determining the antioxidant capacity, based on the fact that the Fe3+ -TPTZ complex is reduced in the presence of antioxidants to form blue complex Fe2+ -TPTZ and that this complex gives maximum absorbance at 593 nm. Three mililiter FRAP reactive (TPTZ, FeCl3, and acetate buffer) and 100 μL extract or blank sample were added experimental tubes and mixed. After 4 min, samples were determined at 593 nm. The results were compared with the calibration curve (FeSO4.7H2O) and expressed as μM FeSO4.7H2O equivalents/g extract. The radical scavenging activity (2,2-Diphenyl-1-picrylhydrazyl (DPPH) assay) was measured spectrophotometrically using DPPH radical (18). Briefly, 50 μL stock solutions (extract) were mixed with 5 mL fresh 0.004% (w/v) DPPH solution. The mixture was incubated for 30 min at room temperature in darkness. After incubation, samples were detected at 517 nm. The radical scavenging activity was given as IC50 (mg/mL). All antioxidant activity assays were applied in triplicate.
Antimicrobial Activity Determination
The antimicrobial activities of the root extracts were determined according to a microwell dilution method and the minimal inhibitor concentration (MIC) values were calculated. All tested microorganisms were obtained from the Department of Biology, Faculty of Science at Karadeniz Technical University: Bacillus cereus (B. cereus) RSKK 709, Bacillus subtilis (B. subtilis) subsp. spizizenii ATCC 6633, Staphylococcus aureus (S. aureus) ATCC 25923, Enterococcus faecalis (E. faecalis) ATCC 29212, Escherichia coli (E. coli) ATCC 25922, Pseudomonas aeruginosa (P. aeruginosa) ATCC 27853 Klebsiella pneumoniae (K. pneumonia) ATCC 700603, Enterobacter cloacae (E. cloacae) ATCC 13047, Yersinia pseudotuberculosis (Y. pseudotuberculosis) ATCC 911, Acinetobacter baumannii (A. baumannii) RSKK 02026, and Candida albicans (C. albicans) ATCC 14053. The stock solutions (extract) were prepared with pure water at the last concentration of 40 mg/mL and sterilized by filtration via 0.45 μm Millipore filters. One-hundred microliter stock solution was added to the first well and then serial 2-fold dilutions were made in order to obtain a concentration range from 20 to 0.039 μg/mL in each well-containing nutrient broth. The last two wells were used as a sterility control (containing culture broth plus 100 µL of stock solution, without antimicrobial substance) and a growth control. Each test and growth control wells were inoculated with 5 µL of a bacterial suspension (5 × 105 CFU/well). Experiments were performed in triplicate and the microdilution trays were incubated at 28 or 30 or 37 °C. Bacterial growth was determined by addition of 40 µL of an INT (2-(4-iodophenyl)-3-(4-nitrophenyl)-5-phenyltetrazolium chloride) alcoholic solution (0.2 mg/mL). The trays were again incubated given above for 30 min, and in those wells, where bacterial growth occurred, INT changed from yellow to purple. The MIC was described as the concentration in the well containing lowest compound dose that monitored no growth. Ampicillin, amikacin, and fluconazole were used as standard antibacterial and antifungal agents (19).
Animals
This study was approved by the Local Ethics Council of Gazi University for Animal Experiments (G.Ü.ET-15.053). 36 male Wistar albino rats (200-250 g) were used. The animals were maintained under a 12 h light/dark cycle and room temperature and were housed singly and fed with standard rat food and water ad libitum.
Diabetes Induction and in-vivo Wound Healing Experiment
The animals were randomly divided into three main groups: non-diabetic control (NDM), diabetic control (STZ-DM), and P. erecta-treated (MEPE) (n = 12/group). Diabetes was induced in STZ-DM and MEPE groups by a single dose intraperitoneal administration of streptozotocin (STZ) (60 mg/kg, Sigma-Aldrich, USA) freshly prepared in sodium citrate buffer (0.1 mol/L, pH 4.5). Blood glucose levels were measured 72 h after STZ administration by glucometer (Accu-Chek Performa Nano, Roche Diagnostics, GER), and those with above 250 mg/dL were accepted as diabetic. The animals were deeply anesthetized by intramuscular administration of ketamine HCl (50 mg/kg) and xylazine HCl (5 mg/kg) (Alfamine and Alfazyne, Alfasan, Woerden, Holland). The dorsum of the rat was shaved and sterilized. Six full-thickness excisional skin wounds were opened in all rats by using an 8-mm punch (Acu-Punch; Acuderm, USA). The wounds were topically treated with P. erecta root extract (50 mg/kg, dissolved in physiological saline, single daily dose) using a sterile pipette in the MEPE groups. No treatment was applied to the NDM and STZ-DM groups.
Wound Area and Wound Contraction Rate Determination
The wound areas were photographed and measured using the ImageJ software (NIH, USA) during the healing process. The wound contraction rate refers to the wound reduction in the original wound area. This rate was calculated as follows: [(wound area day 0–wound area on day 3 or 7)/wound area day 0] × 100. Values are defined as wound area (mm2) and the rate of wound contraction.
Biochemical Estimations
On days 3 and 7, six animals from each group were killed with intracardiac blood aspiration under anesthesia to collect wound tissue, stored at − 80 °C. At the same time, skin punches indicating 0 days were also collected from non-wounded animals of NDM and STZ-DM groups. Lipid peroxidation was determined spectrophotometrically according to the method detailed before at 535 nm (20). The reactive nitrogen oxide species (NOx) levels were determined spectrophotometrically at 540 nm by Griess reaction (21). Levels of glutathione (GSH) were measured spectrophotometrically according to a modified Ellman method at 412 nm (22). The total amount of collagen (type 1-5) in the wound tissues was determined using a commercial kit (Sircol Collagen Assay Kit, Biocolor, UK) following the manufacturer’s instructions and in the manner specified by Tsuda et al. (23). The amount of collagen in the tissues was calculated as μg/mg tissue.
Statistical Analysis
All results were presented as mean ± standard deviation (SD). Statistical data comparison was performed using one-way ANOVA and a Post-hoc Tukey test (SPSS v. 16, IBM Inc, USA). P < 0.001 was considered statistically significant.
Results and Discussion
In diabetic patients, impaired wound healing or non-healing wounds are some of the important factors that decrease the quality of life and increase healthcare costs. Previous studies have reported that diabetic wounds exhibit delayed wound closure, decreased collagen levels, and affected oxidative events (2, 24–26). Medical plants are often used in the treatment of many diseases as well as in wound healing. As mentioned in ethnobotanical studies, the Potentilla genus is traditionally used for this purpose, but more scientific data is required to determine its efficacy (5, 14 and 15). The current study showed that P. erecta extract has therapeutic effects on the diabetic wound healing process on days 3 and 7 in rats.
During epithelialization, the epithelial cells at the wound edge migrate towards the wound bed and proliferate to provide wound contraction. Wound contraction is an important indicator of epithelialization and wound starting to heal (27). Delayed or impaired wound healing is a major problem in diabetic patients. In our study, it was determined that the wound area in the diabetic rats was higher than that of the non-diabetic rats (P < 0.001), and therefore wound healing was delayed (Table 1 and Figure 1). Topical administration of MEPE, when compared to the diabetic control group (STZ-DM), showed a significant decrease in the wound area on days 3 and 7 (P < 0.001). Also, it was observed that the wound area and wound contraction rate in the MEPE group were similar to the non-diabetic control group. Recently, another Potentilla species (P. fulgens) showed significant wound healing activity, especially on epithelialization (28). Otherwise, gallic acid (0.41 mg/g extract), rutin (0.29 mg/g extract) and protocatechuic acid (0.08 mg/g extract) were identified in the MEPE (Table 2 and Figure 2). The other phenolic compounds of the MEPE determined, but not identified because it is below the limit of detection (LOD). It has been shown that gallic acid and rutin decreased wound area and accelerate wound healing (29, 30). In considering these results, it can be argued that P. erecta root extract increases the wound closure of diabetic wound healing to the levels of normal wound healing and contributes to the improvement and acceleration of the diabetic wound healing process.
The collagen is one of the major proteins of the connective tissue and plays a role in cell proliferation and migration as well as providing structural support during the wound healing process (31). In our study, total collagen levels of diabetic rats decreased significantly compared to non-diabetic rats on all days (P < 0.001) (Table 3). In many studies supporting our results, it has been reported that collagen and hydroxyproline (a major component of collagen) content decreases in the skin and wound tissues in diabetic patients and diabetes-induced experimental animal models (24, 32). P. erecta extract significantly increased total collagen level on the 7th day of healing compared to diabetic control (STZ-DM) group (P < 0.001). Similarly, Kundu et al. reported that the ethanolic extract of P. fulgens increases (another species of Potentilla) the hydroxyproline levels in wound tissue (28). Our findings may suggest that the P. erecta extract is effective in the proliferation phase of diabetic wound healing process by increasing collagen levels and improves wound healing in diabetic rats.
Phenolic compounds play an active role in the treatment of various diseases by showing antimicrobial and antioxidant activity (33). Infection is one of the important local factors affecting wound healing. The increasing bacteria population in the wound area may delay wound healing (34). MEPE showed antimicrobial activity against S. aureus, P. aeruginosa, E. coli, which are pathogens and may cause delayed wound healing (34). However, all determined activities were found to be weak compared to the reference antibiotics (Table 4). Grujić-Vasić et al. reported that the various extracts of P. erecta showed antimicrobial activity in different degrees against bacteria such as S. aureus and E. coli, in support of our results (35). These findings suggest that P. erecta methanol root extract can help to wound healing by showing antimicrobial activity. Moreover, antioxidant defense systems are very important to protect against reactive oxygen species (ROS)-induced cellular damage. These systems, known as endogenous or exogenous sources, may also contribute positively to the wound healing process (36, 37). Three different methods were used to evaluate the antioxidant properties of MEPE; the amounts of the total polyphenol content (TPC), the ferric reducing antioxidant assay (FRAP) value, and the DPPH scavenging activity are listed in Table 5.
In many studies, cellular damage caused by ROS is determined by lipid, protein, or DNA-induced oxidation products (38). MDA is the end product of lipid peroxidation and the amount at the tissue can be determined by measuring TBARs levels. Compared with the NDM group, TBARs levels were significantly higher in the STZ-DM group on day 0 (P < 0.001), but no difference on days 3 and 7. Treatment with MEPE significantly decreased the TBARs levels when compared to NDM and STZ-DM groups on days 3 and 7 (P < 0.001) (Table 3). These decreases in TBARs levels may be derived from phenolic compounds of MEPE and its antioxidant activities. FRAP, TPC, DDPH values indicate that MEPE has antioxidant activity (Tables 3 and 5).
Oxygen, reactive oxygen species and reactive nitrogen species are important factors affecting the wound healing process. Sufficient oxygen must be supplied to the wound area for removal of bacteria, angiogenesis, and collagen synthesis (39). In addition, ROS and RNS may serve as secondary messengers and contribute to the healing process (40, 41). Nitric oxide is a highly reactive biological messenger produced via the NOS enzyme by the macrophage, platelet, and fibroblast cells in the wound area (42–44). Nitric oxide may contribute to the improvement of the healing process by enhancing collagen synthesis and storage, angiogenesis, and cell proliferation (45). Witte et al. reported that nitric oxide donors could partially reverse the delayed wound healing caused by diabetes (46). We found that NOx levels in diabetic wound tissues reduced compared to non-diabetic wound tissues (P < 0.001), but then this decreased level elevated to non-diabetic healing level with MEPE administration on days 3 and 7 (Table 3). Ugusman et al. found that rutin (determined in MEPE) promotes NO production via eNOS in cultured human umbilical vein endothelial cells (HUVEC) (47). A strong relationship between NOx, collagen, and fibroblasts has been reported in the literature. Witte et al. reported that NO enhances collagen synthesis in fibroblast cell culture (48). Furthermore, fibroblasts first show up in significant amounts in the wound on day 3 after injury and achieve peak amounts around day 7 (49). When the increase of the collagen and the NOx levels on day 7 were evaluated together, P. erecta methanol root extract may have triggered synthesis or deposition of collagen by increasing NOx level.
Glutathione, a low molecular weight endogenous antioxidant, is a highly effective tripeptide in the protection of oxidative stresses in cells and tissues (41). Compared with the NDM group, GSH levels were significantly lower in the STZ-DM group on all days (P < 0.001) (Table 3). Rasik and Shukla and Dinçer and Gülen reported that GSH levels in diabetic wound tissues decreased compared with healthy (non-diabetic) wound tissues (50, 51). A statistically significant increase in GSH levels was determined in the MEPE group when compared with the STZ-DM group on days 3 and 7 (P < 0.001) (Table 3). Kundu et al. showed that P. fulgens significantly increased GSH levels in the wound tissues (28). Rutin, chlorogenic acid, caffeic acid, and ferulic acid (determined in MEPE) increases cellular GSH content under in-vivo and in-vitro conditions (52–55). Based on these studies, it can be considered that the P. erecta extract increases the antioxidant capacity of the wound tissue by GSH and consequently positively contributed to the wound healing process.
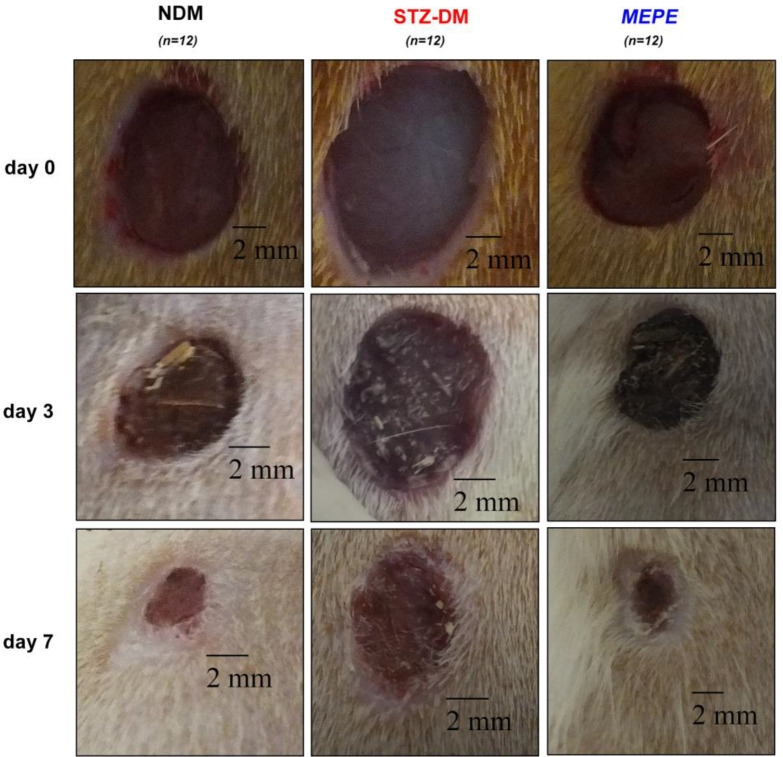
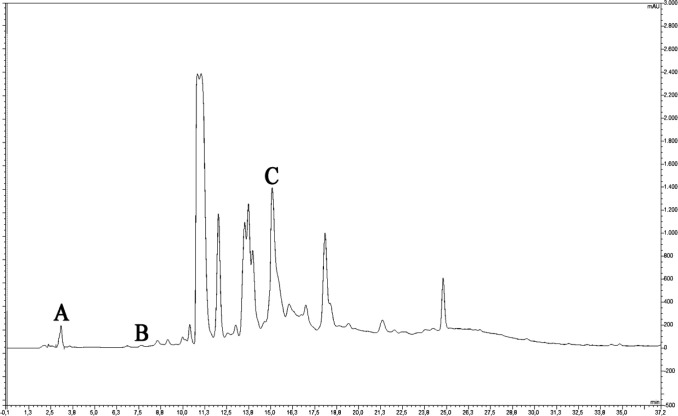
Wound areas (mm2) and the rates of wound contraction of rats
Post wounding (days) | NDM (n = 12) | STZ-DM (n = 12) | MEPE (n = 12) |
---|---|---|---|
0 | 62.09 ± 3.50 | 62.89 ± 3.73 | 60.30 ± 1.44 |
3 | 29.84 ± 3.17a | 43.80 ± 2.05a, b, e | 30.77 ± 2.11a, b, d |
7 | 7.04 ± 1.15a, c | 24.07 ± 1.68a, b, c, e | 8.02 ± 0.99a, b, c, d |
Phenolic constituents of the MEPE by RP-HPLC-UV
Phenolic compounds | mg phenolic/g extract | |
---|---|---|
1 | Gallic Acid | 0.411 |
2 | Protocatechuic Acid | 0.082 |
3 | p-OH Benzoic Acid | < LOD |
4 | Chlorogenic Acid | < LOD |
5 | Vanillic Acid | < LOD |
6 | Caffeic Acid | < LOD |
7 | Syringic Acid | < LOD |
8 | Ellagic Acid | < LOD |
9 | p-coumaric Acid | < LOD |
10 | Rutin | 0.287 |
11 | Ferulic Acid | < LOD |
12 | Myricetin | < LOD |
13 | Fisetin | < LOD |
14 | Quercetin | < LOD |
15 | Apigenin | < LOD |
16 | Kaempferol | < LOD |
17 | Isorhamnetin | < LOD |
Effects of the MEPE on some important biochemical parameters during wound healing process on days 0, 3, and 7
Collagen | NOx | TBARs | GSH | ||
---|---|---|---|---|---|
NDM | 0 day | 34.73 ± 1.37 | 776.64 ± 76.71 | 57.12 ± 5.02 | 5.53 ± 0.41 |
3 day (n = 6) | 18.04 ± 1.32a | 773.76 ± 58.59 | 43.19 ± 3.51a | 2.78 ± 0.15a | |
7 day (n = 6) | 27.21 ± 0.53a,c | 586.56 ± 47.70a,c | 47.18 ± 2.61a | 1.47 ± 0.05a,c | |
STZ-DM | 0 day | 15.93 ± 0.90a | 526.08 ± 31.27a | 68.14 ± 5.25a | 0.98 ± 0.98a |
3 day (n = 6) | 7.26 ± 0.53a,b,e | 522.24 ± 36.55a,e | 41.92 ± 3.13a,b | 0.81 ± 0.07a,e | |
7 day (n = 6) | 7.26 ± 0.60a,b,e | 555.84 ± 10.14a | 44.01 ± 3.86a,b | 0.93 ± 0.03a,e | |
MEPE | 3 day (n = 6) | 7.25 ± 0.51a,b,e | 837.12 ± 44.72b,d | 15.99 ± 1.70a,b,d,e | 1.27 ± 0.08a,d,e |
7 day (n = 6) | 26.86 ± 1.93a,b,c,d | 716.16 ± 35.44b,d | 16.44 ± 1.71a,b,d,e | 2.46 ± 0.16a,e,b,c,d |
Minimal inhibitor concentrations (MIC, μg/mL extract) of plant extract and reference antibiotics
MEPE | Ampicillin | Amikacin | Fluconazole | |||
---|---|---|---|---|---|---|
* Gram+ bacteria | ||||||
B. cereus | 625 | - | 0.49 | - | ||
B. subtilis subsp. spizizenii | 2500 | 0.98 | 0.49 | - | ||
S. aureus | 625 | 0.49 | 0.98 | - | ||
E. faecalis | 1250 | 1.95 | 62.5 | - | ||
* Gram- bacteria | ||||||
E. coli | 1250 | 7.81 | 0.49 | - | ||
P. aeruginosa | 5000 | - | 0.49 | - | ||
K. pneumonia | 1250 | - | 0.49 | - | ||
E. cloacae | 2500 | - | 0.98 | - | ||
Y. pseudotuberculosis | 156.25 | 125 | 31.25 | - | ||
A. baumannii | 5000 | 7.81 | 0.98 | - | ||
* Fungi | ||||||
C. albicans | 5000 | - | - | 1.95 |
Total phenolic contents, ferric reducing antioxidant power, and radical scavenging activities of MEPE
MEPE | |
---|---|
TPC (mg GAE/g extract) | 339.467 ± 12.563 |
FRAP (mM FeSO4.7H2O equivalents/g extract) | 5.080 ± 0.005 |
DPPH (IC50: μg/mL) | 0.012 ± 0.001 |
Conclusion
Our results demonstrated that contraction in the wound tissues of P. erecta treated rats significantly increased compared to diabetic control. P. erecta treated rats showed an increase in the levels of NOx, GSH, and collagen and a decrease in the levels of TBARs. Considering biological activities, morphological and biochemical analyzes, for the first time, may indicate that P. erecta methanol root extract improves and accelerate healing and alter oxidative events in the diabetic wound healing process in support of ethnobotanical studies.
Acknowledgements
References
-
1.
American Diabetes Association AD. Diagnosis and classification of diabetes mellitus. Diabetes Care. 2009;32(Suppl 1):S62-7. [PubMed ID: 19118289].
-
2.
Berk A, Dokumacı AH, Kaymaz MB. Wound healing and medicinal plants used in the treatment of diabetic wounds. Saglik Bilim. Derg. 2015;24:185-92.
-
3.
Peplow PV, Baxter GD. Gene expression and release of growth factors during delayed wound healing: a review of studies in diabetic animals and possible combined laser phototherapy and growth factor treatment to enhance healing. Photomed. Laser Surg. 2012;30:617-36. [PubMed ID: 23075146].
-
4.
Li H, Fu X, Zhang L, Huang Q, Wu Z, Sun T. Research of PDGF-BB gel on the wound healing of diabetic rats and its pharmacodynamics. J. Surg. Res. 2008;145:41-8. [PubMed ID: 18082770].
-
5.
Tomczyk M, Latté KP. Potentilla-A review of its phytochemical and pharmacological profile. J. Ethnopharmacol. 2009;122:184-204. [PubMed ID: 19162156].
-
6.
Tunon H, Olavsdotter C, Bohlin L, Tunon H, Olavsdotter C, Bohlin L. Evaluation of anti-inflammatory activity of some Swedish medicinal plants Inhibition of prostaglandin biosynthesis and PAF-induced exocytosis. J. Ethnopharmacol. 1995;48:61-76. [PubMed ID: 8583796].
-
7.
Ivancheva S, Stantcheva B. Ethnobotanical inventory of medicinal plants in Bulgaria. J. Ethnopharmacol. 2000;69:165-72. [PubMed ID: 10687872].
-
8.
Ozdemir E, Alpinar K. An ethnobotanical survey of medicinal plants in western part of central Taurus Mountains: Aladaglar (Nigde - Turkey). J. Ethnopharmacol. 2015;166:53-65. [PubMed ID: 25771355].
-
9.
Kayani S, Ahmad M, Sultana S, Khan Shinwari Z, Zafar M, Yaseen G, Hussain M, Bibi T. Ethnobotany of medicinal plants among the communities of Alpine and Sub-alpine regions of Pakistan. J. Ethnopharmacol. 2015;164:186-202. [PubMed ID: 25680839].
-
10.
Malla B, Gauchan DP, Chhetri RB. An ethnobotanical study of medicinal plants used by ethnic people in Parbat district of Western Nepal. J. Ethnopharmacol. 2015;165:103-17. [PubMed ID: 25571849].
-
11.
Kumar B, Vijayakumar M, Govindarajan R, Pushpangadan P. Ethnopharmacological approaches to wound healing-Exploring medicinal plants of India. J. Ethnopharmacol. 2007;114:103-13. [PubMed ID: 17884316].
-
12.
Güner A, Aslan S, Ekim T, Vural M, Babaç M. A Checklist of the Flora of Turkey (Vascular Plants). Nezahat Gökyigit Botanic Garden and Floristics Research Society Publication, Istanbul. 2012:178.
-
13.
Hoffmann J, Casetti F, Bullerkotte U, Haarhaus B, Vagedes J, Schempp C, Wölfle U. Anti-ınflammatory effects of agrimoniin-enriched fractions of Potentilla erecta. Molecules. 2016;21:792.
-
14.
Chevallier A. Encyclopedia of Herbal Medicine. 3rd ed. DK Penguin Random House, London. 2016:256.
-
15.
Zevin IV, Altman N, Zevin LV. A Russian Herbal : Traditional Remedies for Health and Healing. 1th ed. Healing Arts Press, Rochester-Vermont. 1997:142.
-
16.
Singleton VL, Rossi JA. Colorimetry of total phenolics with phosphomolybdic–phosphotungstic acid reagents. Am. J. Enol. Vitic. 1965;16:144-58.
-
17.
Benzie I, Strain J. The ferric reducing ability of plasma (FRAP) as a measure of ‘antioxidant power’: the FRAP assay. Anal. Biochem. 1996;239:70-6. [PubMed ID: 8660627].
-
18.
Kartal N, Sokmen M, Tepe B, Daferera D, Polissiou M, Sokmen A. Investigation of the antioxidant properties of Ferula orientalis L using a suitable extraction procedure. Food Chem. 2007;100:584-89.
-
19.
Güllüce M, Sökmen M, Daferera D, Aǧar G, Özkan H, Kartal N, Polissiou M, Sökmen A, Şahin F. In-vitro antibacterial, antifungal, and antioxidant activities of the essential oil and methanol extracts of herbal parts and callus cultures of Satureja hortensis L. J. Agric. Food Chem. 2003;51:3958-65. [PubMed ID: 12822930].
-
20.
Casini AF, Ferrali M, Pompella A, Maellaro E, Comporti M. Lipid peroxidation and cellular damage in extrahepatic tissues of bromobenzene-intoxicated mice. Am. J. Pathol. 1986;123:520-31. [PubMed ID: 3717304].
-
21.
Miranda KM, Espey MG, Wink DA. A rapid, simple spectrophotometric method for simultaneous detection of nitrate and nitrite. Nitric Oxide. 2001;5:62-71. [PubMed ID: 11178938].
-
22.
Aykac G, Uysal M, Yalcin AS, Kocak-Toker N, Sivas A, Oz H. The effect of chronic ethanol ingestion on hepatic lipid peroxide, glutathione, glutathione peroxidase and glutathione transferase in rats. Toxicology. 1985;36:71-6. [PubMed ID: 4040665].
-
23.
Tsuda K, Nakatani T, Sugama J, Okuwa M, Sanada H. Influence of the timing of switching a protein-free to a protein-containing diet on the wound healing process in a rat all-layer skin defect. Int. Wound J. 2010;7:135-46. [PubMed ID: 20602645].
-
24.
Blakytny R, Jude E. The molecular biology of chronic wounds and delayed healing in diabetes. Diabet. Med. 2006;23:594-608. [PubMed ID: 16759300].
-
25.
Goksen S, Balabanli B, Coskun-Cevher S. Application of platelet derived growth factor-BB and diabetic wound healing: the relationship with oxidative events. Free Radic. Res. 2017;51:498-505. [PubMed ID: 28480814].
-
26.
Dindar B, Kaltalioglu K, Coskun Cevher Ş. Effect of dual growth factor administration on oxidative markers during acute stage wound healing in rats. Turk. J. Zool. 2017;41:841-7.
-
27.
Chattopadhyay D, Arunachalam G, Mandal AB, Sur TK, Mandal SC, Bhattacharya SK. Antimicrobial and anti-inflammatory activity of folklore: Mallotus peltatus leaf extract. J. Ethnopharmacol. 2002;82:229-37. [PubMed ID: 12242000].
-
28.
Kundu A, Ghosh A, Singh NK, Singh GK, Seth A, Maurya SK, Hemalatha S, Laloo D. Wound healing activity of the ethanol root extract and polyphenolic rich fraction from Potentilla fulgens. Pharm. Biol. 2016;54:2383-93. [PubMed ID: 27043472].
-
29.
Yang DJ, Moh SH, Son DH, You S, Kinyua AW, Ko CM, Song M, Yeo J, Choi YH, Kim KW. Gallic acid promotes wound healing in normal and hyperglucidic conditions. Molecules. 2016;21:1-15.
-
30.
Almeida JS, Benvegnú DM, Boufleur N, Reckziegel P, Barcelos RCS, Coradini K, de Carvalho LM, Bürger ME, Beck RCR. Hydrogels containing rutin intended for cutaneous administration: efficacy in wound healing in rats. Drug Dev. Ind. Pharm. 2012;38:792-9. [PubMed ID: 22066462].
-
31.
Brett D. A review of collagen and collagen-based wound dressings. Wounds. 2008;20:347-56. [PubMed ID: 25941895].
-
32.
Galeano M, Torre V, Deodato B, Campo GM, Colonna M, Sturiale A, Squadrito F, Cavallari V, Cucinotta D, Buemi M, Altavilla D. Raxofelast, a hydrophilic vitamin E-like antioxidant, stimulates wound healing in genetically diabetic mice. Surgery. 2001;129:467-77. [PubMed ID: 11283539].
-
33.
Bektaş E, Kaltalıoğlu K, Şahin H, Türkmen Z, Kandemir A. Analysis of phenolic compounds, antioxidant and antimicrobial properties of some endemic medicinal plants. Int. J. Second. Metab. 2018;5:75-86.
-
34.
Bowler PG, Duerden BI, Armstrong DG. Wound microbiology and associated approaches to wound management. Clin. Microbiol. Rev. 2001;14:244-69. [PubMed ID: 11292638].
-
35.
Grujić-Vasić J, Pilipović S, Bosnić T, Redžić S. Antimicrobial activity of different extracts from rhizome and root of Potentilla erecta L. Raeuschel and Potentilla alba L. Rosaceae. Acta Med. Acad. 2006;35:19-22.
-
36.
Fitzmaurice SD, Sivamani RK, Isseroff RR. Antioxidant therapies for wound healing: a clinical guide to currently commercially available products. Skin Pharmacol. Physiol. 2011;24:113-26. [PubMed ID: 21242718].
-
37.
Süntar I, Küpeli Akkol E, Keles H, Yesilada E, Sarker SD, Baykal T. Comparative evaluation of traditional prescriptions from Cichorium intybus for wound healing: Stepwise isolation of an active component by in-vivo bioassay and its mode of activity. J. Ethnopharmacol. 2012;143:299-309. [PubMed ID: 22750434].
-
38.
Urso ML, Clarkson PM. Oxidative stress, exercise, and antioxidant supplementation. Toxicology. 2003;189:41-54. [PubMed ID: 12821281].
-
39.
Kurahashi T, Fujii J. Roles of Antioxidative enzymes in wound healing. J. Dev. Biol. 2015;3:57-70.
-
40.
Dunnill C, Patton T, Brennan J, Barrett J, Dryden M, Cooke J, Leaper D, Georgopoulos NT. Reactive oxygen species (ROS) and wound healing: the functional role of ROS and emerging ROS-modulating technologies for augmentation of the healing process. Int. Wound J. 2017;14:89-96. [PubMed ID: 26688157].
-
41.
Schäfer M, Werner S. Oxidative stress in normal and impaired wound repair. Pharmacol. Res. 2008;58:165-71. [PubMed ID: 18617006].
-
42.
Frank S, Kämpfer H, Wetzler C, Pfeilschifter J. Nitric oxide drives skin repair: Novel functions of an established mediator. Kidney Int. 2002;61:882-8. [PubMed ID: 11849442].
-
43.
Schwentker A, Vodovotz Y, Weller R, Billiar TR. Nitric oxide and wound repair: role of cytokines? Nitric Oxide. 2002;7:1-10. [PubMed ID: 12175813].
-
44.
Hou YC, Janczuk A, Wang PG. Current trends in the development of nitric oxide donors. Curr. Pharm. Des. 1999;5:417-41. [PubMed ID: 10390607].
-
45.
Luo J, Chen AF. Nitric oxide: a newly discovered function on wound healing. Acta Pharmacol. Sin. 2005;26:259-64. [PubMed ID: 15715920].
-
46.
Witte MB, Kiyama T, Barbul A. Nitric oxide enhances experimental wound healing in diabetes. Br. J. Surg. 2002;89:1594-601. [PubMed ID: 12445072].
-
47.
Ugusman A, Zakaria Z, Chua KH, Nordin NAMM, Abdullah Mahdy Z. Role of rutin on nitric oxide synthesis in human umbilical vein endothelial cells. ScientificWorldJournal. 2014;2014:1-9.
-
48.
Witte MB, Thornton FJ, Efron DT, Barbul A. Enhancement of fibroblast collagen synthesis by nitric oxide. Nitric Oxide Biol. Chem. 2000;4:572-82.
-
49.
Regan M, Barbul A. The Cellular Biology of Wound Healing. In: Schlag G, Redl H, editors. Fibrin Sealing in Surgical and Nonsurgical Fields. 1th ed. Verlag, Berlin-Heidelberg: Springer; 1994. p. 3-17.
-
50.
Rasik AM, Shukla A. Antioxidant status in delayed healing type of wounds. Int. J. Exp. Pathol. 2000;81:257-63. [PubMed ID: 10971747].
-
51.
Dinçer S, Gülen Ş. Wound tissue malondialdehyde and glutathione levels in leptin treated healthy and diabetic rats. Erciyes Med. J. 2010;32:161-6.
-
52.
Chen WC, Liou SS, Tzeng TF, Lee SL, Liu IM. Effect of topical application of chlorogenic acid on excision wound healing in rats. Planta Med. 2013;79:616-21. [PubMed ID: 23568627].
-
53.
Yang SY, Kang JH, Seomun Y, Lee KW. Caffeic acid induces glutathione synthesis through JNK/AP-1-mediated γ-glutamylcysteine ligase catalytic subunit induction in HepG2 and primary hepatocytes. Food Sci. Biotechnol. 2015;24:1845-52.
-
54.
Ghaisas MM, Kshirsagar SB, Sahane RS. Evaluation of wound healing activity of ferulic acid in diabetic rats. Int. Wound J. 2014;11:523-32. [PubMed ID: 23236955].
-
55.
Abarikwu SO, Olufemi PD, Lawrence CJ, Wekere FC, Ochulor AC, Barikuma AM. Rutin, an antioxidant flavonoid, induces glutathione and glutathione peroxidase activities to protect against ethanol effects in cadmium-induced oxidative stress in the testis of adult rats. Andrologia. 2017;49:e12696.