Abstract
Keywords
Synthesis Design 4-Oxo-1 4-dihydroquinoline-3-carbohydrazide HCV NS5B polymerase Molecular modeling studies
Introduction
Hepatitis C caused by the hepatitis C virus (HCV) has been considered as a leading cause for liver fibrosis, cirrhosis, and hepatocellular carcinoma (1, 2). According to the world health organization (WHO) report, chronic HCV infection has been estimated to affect about 71 million individuals worldwide (3). Prior to 2011, a combination of pegylated interferon alpha and ribavirin was used for HCV therapy, which sometimes caused severe adverse reactions, and was poorly tolerated (4). Currently, the treatment for HCV chronic infection consists of the addition of direct-acting antiviral drugs (DAAs) to the ribavirin regimen with or without pegylated interferon (5, 6). DAAs specifically target essential proteins in HCV life cycle including NS3 protease, NS5A serine protease, and NS5B RNA dependent RNA polymerase (7). In recent years, extensive efforts have been focused on discovery of direct-acting antiviral agents (8, 9). However, the emergence of drug resistance due to mutation of virus necessitate developing of new anti-HCV agents with different mode of actions and structures.
Non-structural protein 5B (NS5B) is a RNA-dependent RNA polymerase that plays a major role in the replicating the viral RNA of HCV. NS5B has no counterpart in human cells, and as a consequence, it is emerged as an attractive therapeutic target. Right-hand-like structure of NS5B polymerase consists of palm, thumb and fingers subdomains. Molecules that bind to and inhibit NS5B polymerase are classified into nucleoside inhibitors (NIs) that target active site and non-nucleoside inhibitors (NNIs) that target one of five allosteric sites referred as the thumb I, thumb II, palm I, palm II and palm-β (10, 11). The NS5B polymerase active site in the top region of the palm subdomain contains two Mg2+ or Mn2+ ions that are kept in their place by conserved residues Asp318, Asp319, and Asp220 (12). The metal ions play a critical role in the formation of the phosphodiester bond between sequential nucleotides (13). Therefore, compounds featuring chelating motif that bind to metal ions can be attributed to NS5B inhibition. This class of inhibitors represents a rare type of active site binding NNIs. Similar to NIs, active site binding NNIs have a high genetic barrier to development of drug resistance, because they bind to the highly conserved active site whereas typical NNIs bind to highly mutable allosteric sites (14-16). Active site binding NNIs inhibitors with a chelating motif generally also bear an aromatic ring directly connected to the chelating core. Since HCV NS5B polymerase shares a similar active site fold to HIV integrase (17), numerous HIV integrase inhibitors including diketoacids (compound 1), 5-hydroxy-pyrimidinones (compound 2), 2-hydroxyisoquinoline-1,3-diones (compound 3), quinolones (compounds 4, 5) have shown potent inhibition against HCV NS5B (Figure 1) (18-23).
Quinolones are among the most privileged scaffolds in medicinal chemistry for designing inhibitors of interesting targets, a notable example being HIV IN inhibitor elvitegravir (24). We have previously introduced some quinolone derivatives based on HIV IN pharmacophores that effectively inhibited HIV-1 in cell culture (compound 6 as an example, Figure 1) (25-33). We expected that these derivatives could be HCV inhibitors through targeting NS5B. On this basis, we decided to retain quinolone scaffold as a main core while make changes on substituents on it. Previous studies focused on aromatic substitution on C-6 and C-7 of quinolone scaffold. This prompted us to design analogues with an aromatic substitution on other position of quinolone core. So we introduced arylidenehydrazide fragment at position 3. Since most of quinolone NS5B inhibitor possesses two hydrophobic moieties, we put a O-benzyl group at position C-6 of quinolone core. Combining the two structural modifications into a quinolone scaffold resulted in a new template. In this template, we explored the effect of various substituted benzylidenehydrazides on HCV inhibition.
Experimental
Chemistry
All chemicals and solvents were purchased from “Merck/Sigma-Aldrich®” company and used directly without purification. Reaction progress was monitored by thin-layer chromatography (TLC) analysis on silicagel 60 F254 plates (Merck), visualized by UV light. Melting points (MP) were determined by the use of a Thomas–Hoover capillary apparatus. Infrared spectra were acquired using a Perkin-Elmer model 1420 spectrophotometer. A Bruker FT-400 MHz instrument (Bruker Biosciences, USA) was used to acquire 1H-NMR spectra. TMS was used as the internal standard and Chloroform-D and DMSO-d6 were used as the solvents. The amounts of coupling constant (J) are estimated in hertz (Hz) and spin multiples are reported as s (singlet), d (doublet), t (triplet), q (quartet) and m (multiplet). The mass spectroscopy experiments were performed by the use of a 6410 Agilent LCMS triple quadrupole mass spectrometer (LC-MS) with an electrospray ionization (ESI) interface. Microanalyses, determined for C and H, were within ± 0.4% of theoretical values.
Synthesis of N-(4-(benzyloxy) phenyl)acetamide (8)
To a mixture of acetaminophen (1.0 g, 6.6 mmol) and sodium hydroxide (0.28 g, 6.6 mmol) in methanol (10 mL), benzyl bromide (0.8 mL, 6.6 mmol) was added and stirred for 12 h at room temperature. Then, lots of water was added to the reaction mixture and the precipitate was filtered off and washed up by water and n-hexane give the compound 8. Yield: 98%; mp: 120 °C; IR (KBr): υ (cm-1) 3276 (NH), 1656 (C=O), 1400-1600 (aromatic); GC-MS m/z: 241.0 (M, 100).
Synthesis of 4-(benzyloxy) aniline (9)
Compound 8 (1 g, 4 mmol) was added to a 50% hydro-alcoholic solution of potassium hydroxide (KOH) and the mixture was refluxed for 48 hours. The mixture was poured into ice water and filtered to give the product 9. Yield: 80%; mp: 45 °C; IR (KBr): υ (cm-1) 2837 - 3474 (NH2), 1400-1600 (aromatic); GC-MS m/z: 199.0 (M, 100).
Synthesis of diethyl 2-(((4-(benzyloxy)phenyl)amino)methylene)malonate (10)
Compound 9 (1 g, 5 mmol) was added to EMME (1 mL, 5 mmol) and the mixture was stirred at room temperature for 10 minutes. After reaction completion, n-hexane was added to the mixture and the precipitate was filtered off to give the compound 10. Yield: 99%; mp: 180 °C; IR (KBr): υ (cm-1) 1669 (C=O), 1400-1600 (aromatic); LC-MS (ESI) m/z 392 (M + 23).
Synthesis of ethyl 6-(benzyloxy)-4-oxo-1,4-dihydroquinoline-3-carboxylate (11)
A diphenyl ether solution of compound 10 (2 g, 5.4 mmol) containing catalytic 2-chlorobenzoic acid was heated by microwave irradiation (250 °C) for 5 minutes. After cooling, the reaction mixture was filtered and the precipitate was washed with n-hexane and water. Yield: 70%; mp: 210 °C; IR (KBr): υ (cm-1) 2725-3241 (NH), 1689 (C=O), 1400-1600 (aromatic); LC-MS (ESI) m/z 324.0 (M+1), 346.1 (M+23).
Synthesis of 6-(benzyloxy)-4-oxo-1,4-dihydroquinoline-3-carbohydrazide (12)
To a solution of compound 11 (2 g, 3.1 mmol) in absolute ethanol (10 mL), hydrazine hydrate (4 mL, 124 mmol) was added and the mixture was refluxed for 36 hours. After evaporation, the precipitated was filtered off and washed by water and n-hexane. Yield: 55%; mp: 65 °C; IR (KBr): υ (cm-1) 2601-3339 (NH), 1671 (C=O), 1400-1600 (aromatic); LC-MS (ESI) m/z: 310.0 (M+1).
General procedure for the synthesis of compounds13-25
To a solution of compound 12 (0.5 g, 1.6 mmol) in absolute ethanol (10 mL), benzaldehyde derivatives (1.6 mmol) were added and the mixture was refluxed for 36 h. After cooling down to room temperature, the mixture was poured into ice water, and the precipitate was isolated by filtration. Target compounds were obtained by crystallization in ethanol.
N′-Benzylidene-6-(benzyloxy)-4-oxo-1, 4-dihydroquinoline-3-carbohydrazide (13)
Yield: 97%: white powder; mp: 130 ºC (decomposed), IR (KBr): υ (cm-1) 2725-3223 (NH), 1658 (C=O), 1450-1600 (aromatic C=C); LC-MS (ESI) m/z 398.0 (M+1, 100%); 1H-NMR (DMSO-d6, 400 MHz) δ ppm, 5.26 (s, 2H, CH2), 7.29-7.37 (3H, m, H2, H4, H6-benzyl), 7.40-7.44 (2H, t, H3, H5-benzyl, J = 8 Hz), 7.50-7.53 (3H, m, H3, H4, H5-benzylidene), 7.71-7.76 (3H, m, H5-quinolone/ H2, H6-benzylidene), 7.80-7.84 (2H, m, H7, H8-quinolone), 8.43 (1H, s, H-C=N-), 8.80 (1H, s, H2-quinolone), 12.97 (1H, br s, NH-amide), 13.50 (1H, s, NH-quinolone); 13C-NMR (DMSO-d6, 100 MHz) δ ppm, 21.53, 70.05, 106.62, 109.49, 122.11, 123.88, 127.59, 128.15, 128.41, 128.79, 128.98, 129.85, 130.00, 132.27, 137.16, 140.18, 143.95, 147.69, 156.22, 162.18, 175.47; Anal. Calcd for C24H19N3O3: C, 72.53; H, 4.82; N, 10.57. Found: C, 72.66; H, 4.59; N, 10.50.
6-(Benzyloxy)-N′-(4-(methylthio)benzylidene)-4-oxo-1,4-dihydroquinoline-3-carbohydrazide (14)
Yield: 90%; yellow powder; mp: 160 ºC (decomposed), IR (KBr): υ (cm-1) 2533-3513 (NH), 1653 (C=O), 1450-1600 (aromatic C=C); LC-MS (ESI) m/z: 444.0 (M+1, 100%), 909.3 (2M+23, 20%); 1H-NMR (DMSO-d6, 400 MHz) δ ppm, 2.56 (3H, s, CH3), 5.22 (2H, s, CH2), 7.23-7.26 (1H, q, H7-quinolone, J= 5 Hz/ 2 Hz), 7.35-7.41 (4H, m, H3, H5- benzylidene/ H4-benzyl/ NH-amide), 7.43-7.46 (2H, t, H3, H5-benzyl, J=7.6 Hz), 7.53-7.55 (2H, d, H2, H6-benzyl, J=7.4 Hz), 7.57-7.59 (1H, d, H8-quinolone, J=8.7 Hz), 7.70-7.72 (2H, d, H2, H6-benzylidene, J=8.3 Hz), 7.75-7.76 (1H, d, H5-quinolone, J=3 Hz), 8.30 (1H, d, H-C=N-, J=6 Hz), 8.78 (1H, s, H2-quinolone), 12.97 (1H, br s, NH-amide), 14.86 (1H, s, NH-quinolone); 13C-NMR (DMSO-d6, 100 MHz) δ ppm, 14.73, 70.07, 106.68, 109.58, 121.72, 124.04, 126.07, 127.52, 128.02, 128.16, 128.43, 128.98, 131.37, 134.53, 137.12, 141.27, 143.55, 147.44, 156.32, 161.99, 175.58; Anal. Calcd for C25H21N3O3S: C, 72.53; H, 4.82; N, 10.57. Found: C, 72.42; H, 4.56; N, 10.23.
6-(Benzyloxy)-N′-(4-fluorobenzylidene)-4-oxo-1,4-dihydroquinoline-3-carbohydrazide (15)
Yield: 85%; white powder; mp: 305 ºC (decomposed), IR (KBr): υ (cm-1) 2680-3444 (NH), 1662 (C=O), 1450-1600 (aromatic C=C); LC-MS (ESI) m/z 416.2 (M+1, 100%), 853.3 (2M+23, 71%); 1H-NMR (DMSO-d6, 400 MHz) δ ppm, 5.26 (2H, s, CH2), 7.29-7.37 (3H, m, H2, H4, H6-benzyl), 7.40-7.44 (2H, t, H3, H5-benzyl, J=8 Hz), 7.49-7.51 (3H, d, H7-quinolone/ H2, H6-fluorobenzylidene, J=8 Hz), 7.70-7.76 (1H, m, H5, H8-quinolone), 7.80-7.83 (2H, q, H3, H5-fluorobenzylidene, J=4 Hz), 8.42 (1H, s, H-C=N), 8.80 (1H, s, H2-quinolone), 12.98 (1H, br s, NH-amide), 13.55 (1H, s, NH-quinolone); 13C-NMR (DMSO-d6, 100 MHz) δ ppm 70.04, 106.61, 109.33, 116.20, 116.42, 122.42, 123.75, 127.62, 128.15, 128.40, 128.97, 129.66, 129.74, 131.62, 135.57, 137.18, 144.27, 146.50, 156.16, 162.25, 162.44, 164.71, 175.40; Anal. Calcd for C24H18FN3O3: C, 69.39; H, 4.37; N, 10.12. Found: C, 69.21; H, 4.12; N, 10.23.
6-(Benzyloxy)-N′-(4-chlorobenzylidene)-4-oxo-1,4-dihydroquinoline-3-carbohydrazide (16)
Yield: 95%; white powder; mp: 329 ºC (decomposed), IR (KBr): υ (cm-1) 2676-3312 (NH), 1644 (C=O), 1450-1600 (aromatic C=C); LC-MS (ESI) m/z 432.0 (M+1, 100%), 454.0 (M+23, 36%), 885.0 (2M+23, 18%); 1H-NMR (DMSO-d6, 400 MHz) δ ppm, 5.26 (2H, s, CH2), 7.33-7.37 (1H, t, H4-benzyl, J= 8 Hz), 7.40-7.44 (2H, t, H3, H5-benzyl, J= 8 Hz), 7.49-7.54 (5H, m, H7-quinolone/H2, H6-chlorobenzylidene/ H2, H6-benzyl), 7.71-7.79 (4H, m, H3, H5-chlorobenzylidene/ H5, H8-quinolone), 8.43 (1H, s, H-C=N), 8.80 (1H, s, H2-quinolone), 12.98 (1H, br s, NH-amide), 13.49 (1H, s, NH-quinolone). 13C-NMR (DMSO-d6, 100 MHz) δ ppm 70.06, 106.67, 109.48, 121.66, 124.09, 127.51, 128.16, 128.43, 128.98, 129.21, 129.36, 129.56, 130.50, 133.06, 133.90, 134.42, 134.83, 136.50, 137.10, 143.58, 146.61, 156.35, 161.14, 162.12, 175.62; Anal. Calcd for C24H18ClN3O3: C, 66.75; H, 4.20; N, 9.73. Found: C, 66.56; H, 4.03; N, 9.99.
6-(Benzyloxy)-N′-(4-methylbenzylidene)-4-oxo-1,4-dihydroquinoline-3-carbohydrazide (17)
Yield: 98%; white powder; mp: 330 ºC (decomposed), IR (KBr): υ 2765-3204 (NH), 1647 (C=O), 1450-1600 (aromatic C=C); LC-MS (ESI) m/z: 412.2 (M+1, 100%), 845.4 (2M+23, 29%); 1H-NMR (DMSO-d6, 400 MHz) δ ppm, 2.36 (3H, s, CH3), 5.26 (2H, s, CH2), 7.27-7.29 (2H, d, H3, H5-benzylidene, J= 8 Hz), 7.33-7.37 (1H, t, H4-benzyl, J= 8 Hz), 7.40-7.44 (2H, t, H3, H5-benzyl, J= 8 Hz), 7.48-7.51 (3H, m, H7-quinolone/ H2, H6-benzyl), 7.65-7.67 (2H, d, H2, H6-benzylidene, J=8 Hz), 7.70-7.76 (2H, m, H5, H8-quinolone), 8.36 (1H, s, H-C=N), 8.80 (1H, s, H2-quinolone), 12.98 (1H, br s, NH-amide), 13.52 (1H, s, NH-quinolone). 13C-NMR (DMSO-d6, 100 MHz) δ ppm 19.0, 70.07, 106.68, 109.48, 116.22, 116.44, 121.86, 124.00, 127.54, 128.16, 128.43, 128.98, 129.70, 129.78, 131.59, 134.73, 137.13, 143.74, 146.72, 156.30, 162.17, 162.28, 164.74, 175.56; Anal. Calcd for C25H21N3O3: C, 72.98; H, 5.14; N, 10.21. Found: C, 72.65; H, 5.29; N, 10.53.
6-(Benzyloxy)-N′-(4-methoxybenzylidene)-4-oxo-1,4-dihydroquinoline-3-carbohydrazide (18)
Yield: 97%; white powder; mp: 154 ºC (decomposed), IR (KBr): υ (cm-1) 2643-3151 (NH), 1646 (C=O), 1450-1600 (aromatic C=C); LC-MS (ESI) m/z 428.1 (M+1, 100%), 450.1 (M+23, 33%); 1H-NMR (DMSO-d6, 400 MHz) δ ppm, 3.82 (3H, s, CH3), 5.22 (2H, s, CH2), 7.01-7.04 (2H, d, H3, H5-benzylidene, J= 10 Hz), 7.32-7.36 (3H, m, H3, H4, H5-benzyl), 7.40-7.44 (2H, t, H2, H6-benzyl, J=8 Hz), 7.49-7.51 (d, 2H, H2, H6-benzylidene, J=8 Hz), 7.60-7.62 (1H, d, H7-quinolone, J=8 Hz), 7.68-7.74 (2H, m, H5, H8-quinolone, J=8 Hz), 8.28 (1H, s, H-C=N), 8.76 (1H, s, H2-quinolone), 12.98 (1H, br s, NH-amide), 14.18 (1H, s, NH-quinolone). 13C-NMR (DMSO-d6, 100 MHz) δ ppm 55.84, 69.87, 114.69, 115.78, 122.66, 128.17, 128.29, 128.91, 129.66, 137.59, 145.17, 157.12, 158.35, 162.08, 175.03; Anal. Calcd for C25H21N3O4: C, 70.25; H, 4.95; N, 9.83. Found: C, 70.60; H, 4.88; N, 9.60.
6-(Benzyloxy)-N′-(3-fluorobenzylidene)-4-oxo-1,4-dihydroquinoline-3-carbohydrazide (19)
Yield: 75%; white powder; mp: 305 ºC (decomposed), IR (KBr): υ (cm-1) 2670-3254 (NH), 1658 (C=O), 1450-1600 (aromatic C=C); LC-MS (ESI) m/z 416.3 (M+1, 100%), 853.3 (2M+23, 30%); 1H-NMR (DMSO-d6, 400 MHz) δ ppm, 5.27 (2H, s, CH2), 7.26-7.30 (1H, m, H4-benzyl, J=8 Hz), 7.34-7.37 (1H, t, H5-fluorobenzylidene, J=8 Hz), 7.41-7.44 (2H, t, H3, H5-benzyl, J= 8 Hz), 7.50-7.56 (5H, m, H2, H6-benzyl/ H2, H4, H6-fluorobenzylidene), 7.60-7.62 (1H, d, H7-quinolone, J=8 Hz), 7.72 - 7.76 (2H, m, H5, H8-fluorobenzylidene), 8.45 (1H, s, H-C=N); 8.81 (1H, s, H2-quinolone), 12.98 (1H, br s, NH-amide), 13.56 (1H, s, NH-quinolone); 13C-NMR (DMSO-d6, 100 MHz) δ ppm 70.08, 106.70, 109.42, 113.44, 113.67, 117.05, 117.25, 121.73, 123.91, 124.08, 127.52, 128.16, 128.43, 128.98, 131.31, 131.39, 134.51, 137.10, 137.49, 137.57, 143.68, 146.57, 156.36, 161.62, 162.23, 164.05, 175.62; Anal. Calcd for C24H18FN3O3: C, 69.39; H, 4.37; N, 10.12. Found: C, 69.52; H, 4.56; N, 10.30.
6-(Benzyloxy)-N′-(2-fluorobenzylidene)-4-oxo-1,4-dihydroquinoline-3-carbohydrazide (20)
Yield: 70%; white powder; mp: 324 ºC (decomposed), IR (KBr): υ (cm-1) 2581-3527 (NH), 1659 (C=O), 1450-1600 (aromatic C=C); LC-MS (ESI) m/z 416.3 (M+1, 100%), 853.4 (2M+23, 30%); 1H-NMR (DMSO-d6, 400 MHz) δ ppm, 5.27 (2H, s, CH2), 7.30-7.37 (3H, m, H2, H4, H6-benzyl), 7.41-7.44 (2H, t, H3, H5-benzyl, J= 8 Hz/ J=4 Hz), 7.48-7.55 (4H, m, H7-quinolone/ H4, H5, H6-fluorobenzylidene), 7.73-7.77 (2H, m, H5, H8-quinolone), 7.93-7.97 (1H, t, H3-fluorobenzylidene, J= 8 Hz), 8.56 (1H, s, H-C=N), 8.81 (1H, s, H2-quinolone), 12.98 (1H, br s, NH-amide), 13.53 (1H, s, NH-quinolone); 13C-NMR (DMSO-d6, 100 MHz) δ ppm 70.08, 106.68, 109.42, 113.44, 113.67, 117.05, 117.25, 121.73, 123.91, 124.08, 127.52, 128.15, 128.43, 128.99, 131.31, 131.39, 134.51, 137.10, 137.49, 137.57, 143.68, 146.57, 156.36, 161.62, 162.23, 164.05, 175.60; Anal. Calcd for C24H18FN3O3: C, 69.39; H, 4.37; N, 10.12. Found: C, 69.12; H, 4.20; N, 10.33.
6-(Benzyloxy)-N′-(3-chlorobenzylidene)-4-oxo-1,4-dihydroquinoline-3-carbohydrazide (21)
Yield: 70%; white powder; mp: 291 ºC (decomposed), IR (KBr): υ (cm-1) 2620-3396 (NH), 1690 (C=O), 1450-1600 (aromatic C=C); LC-MS (ESI) m/z 432.3 (M+1, 100%), 454.2 (M+23, 70%); 1H-NMR (DMSO-d6, 400 MHz) δ ppm, 5.27 (2H, s, CH2), 7.34-7.37 (1H, t, H4-benzyl, J= 8 Hz/ J=4 Hz), 7.41-7.44 (2H, t, H3, H5-benzyl, J=8 Hz), 7.50-7.55 (5H, m, H2, H6-benzyl/ H5, H7, H8-quinolone), 7.71-7.76 (3H, m, H4, H5, H6-chlorobenzylidene), 7.80 (1H, s, H2-chlorobenzylidene), 8.43 (1H, s, H-C=N), 8.81 (1H, s, H2-quinolone), 12.98 (1H, br s, NH-amide), 13.52 (1H, s, NH-quinolone); 13C-NMR (DMSO-d6, 100 MHz) δ ppm 70.01, 106.51, 108.93, 123.34, 126.10, 126.77, 127.75, 128.13, 128.96, 129.85, 131.16, 134.02, 137.29, 137.41, 145.34, 145.54, 155.94, 163.08, 175.14; Anal. Calcd for C24H18ClN3O3: C, 66.75; H, 4.20; N, 9.73. Found: C, 66.56; H, 4.33; N, 10.01.
6-(Benzyloxy)-N′-(3-methylbenzylidene)-4-oxo-1,4-dihydroquinoline-3-carbohydrazide (22)
Yield: 80%; white powder; mp: 310 ºC (decomposed), IR (KBr): υ (cm-1) 2661- 3354 (NH), 1661 (C=O), 1450-1600 (aromatic C=C); LC-MS (ESI) m/z 412.2 (M+1, 100%), 823.3 (2M+1, 20%); 1H-NMR (DMSO-d6, 400 MHz) δ ppm, 2.37 (3H, s, CH3), 5.27 (2H, s, CH2), 7.25-7.27 (1H, d, H4-benzylidene, J=8 Hz), 7.34-7.38 (2H, t, H5-benzylidene/ H4-benzyl, J= 8 Hz), 7.41-7.44 (2H, t, H3, H5-benzyl, J= 8 Hz), 7.50-7.56 (4H, m, H7-quinolone/ H6-benzylidene/ H2, H6-benzyl), 7.60 (1H, s, H2-benzylidene); 7.73 - 7.76 (2H, d, H5, H8-quinolone, J= 8 Hz/ J=4 Hz), 8.37 (1H, s, H-C=N); 8.81 (1H, s, H2-quinolone), 12.97 (1H, br s, NH-amide), 13.43 (1H, s, NH-quinolone); 13C-NMR (DMSO-d6, 100 MHz) δ ppm 21.40, 70.08, 106.68, 109.58, 121.66, 124.09, 125.15, 127.53, 127.78, 128.16, 128.43, 128.98, 129.15, 131.16, 134.45, 134.88, 137.11, 138.43, 143.55, 147.90, 156.35, 162.05, 175.61; Anal. Calcd for C25H21N3O3: C, 72.98; H, 5.14; N, 10.21. Found: C, 72.70; H, 5.32; N, 10.37.
6-(Benzyloxy)-N′-(2-methylbenzylidene)-4-oxo-1,4-dihydroquinoline-3-carbohydrazide (23)
Yield: 85%; white powder; mp: 290 ºC (decomposed), IR (KBr): υ (cm-1) 2563-3529 (NH), 1650 (C=O), 1450-1600 (aromatic C=C); LC-MS (ESI) m/z 412.2.0 (M+1, 100%), 823 (2M+1, 30%); 1H-NMR (DMSO-d6, 400 MHz) δ ppm, 2.50 (3H, s, CH3), 5.27 (2H, s, CH2), 7.25-7.37 (4H, m, H3-benzylidene/H2, H4, H6-benzyl), 7.41-7.44 (2H, t, H3, H5-benzyl, J= 8 Hz/ J= 4 Hz), 7.50-7.55 (3H, m, H4, H5, H6-benzylidene); 7.73-7.77 (2H, m, H5, H7-quinolone), 7.85-7.87 (1H, d, H8-quinolone, J= 8 Hz), 8.60 (1H, s, H-C=N), 8.81 (1H, s, H2-quinolone), 12.95 (1H, br s, NH-amide), 13.40 (1H, s, NH-quinolone); 13C-NMR (DMSO-d6, 100 MHz) δ ppm 19.64, 70.06, 106.65, 109.62, 121.71, 124.06, 126.55, 126.64, 127.56, 128.12, 128.42, 128.99, 130.11, 131.32, 132.91, 134.52, 137.10, 137.47, 143.60, 146.27, 156.33, 162.07, 175.55; Anal. Calcd for C25H21N3O3: C, 72.98; H, 5.14; N, 10.21. Found: C, 72.74; H, 5.41; N, 10.33.
6-(Benzyloxy)-N′-(3-methoxybenzylidene)-4-oxo-1,4-dihydroquinoline-3-carbohydrazide (24)
Yield: 70%; white powder; mp: 280 ºC (decomposed); IR (KBr), υ (cm-1) 2665-3424 (NH), 1663 (C=O), 1450-1600 (aromatic C=C); LC-MS (ESI) m/z 428.3 (M+1, 100%), 856.4 (2M+1, 14%); 1H-NMR (DMSO-d6, 400 MHz) δ ppm, 3.82 (3H, s, CH3), 5.27 (2H, s, CH2), 7.00-7.03 (1H, m, H2-benzylidene), 7.32-7.44 (6H, m, H4-benzylidene, H2, H3, H4, H5, H6-benzyl); 7.50 - 7.54 (3H, q, H7-quinolone/H5, H6-benzyl, J=8 Hz/ J=4 Hz), 7.72-7.76 (2H, m, H5, H8-quinolone, J=8 Hz/ J= 4 Hz), 8.39 (1H, s, H-C=N); 8.80 (1H, s, H2-quinolone), 12.95 (1H, br s, NH-amide), 13.51 (1H, s, NH-quinolone); 13C-NMR (DMSO-d6, 100 MHz) δ ppm 55.63, 70.07, 106.68, 109.54, 111.94, 116.52, 120.48, 121.67, 124.08, 127.52, 128.17, 128.43, 128.98, 130.35, 134.44, 136.34, 137.11, 143.55, 147.81, 156.35, 159.96, 162.08, 175.62; Anal. Calcd for C25H21N3O4: C, 70.25; H, 4.95; N, 9.83. Found: C, 70.01; H, 5.97; N, 9.68.
6-(Benzyloxy)-N′-(2-methoxybenzylidene)-4-oxo-1,4-dihydroquinoline-3-carbohydrazide (25)
Yield: 85%; white powder; mp: 299 ºC (decomposed), IR (KBr): υ (cm-1) 2481-3164 (NH), 1642 (C=O), 1450-1600 (aromatic C=C); LC-MS (ESI) m/z 428.3 (M+1, 100%), 877.4 (2M+23, 25%); 1H-NMR (DMSO-d6, 400 MHz) δ ppm, 3.90 (3H, s, CH3), 5.26 (2H, s, CH2), 7.02-7.06 (1H, t, H5-benzylidene, J = 8 Hz), 7.12-7.14 (1H, d, H3-benzylidene, J = 8 Hz), 7.34-7.38 (1H, t, H4-benzyl, J = 8 Hz), 7.41-7.46 (3H, m, H3, H5-benzyl/ H4-benzylidene), 7.50-7.53 (3H, m, H2, H6-benzyl/ H6-benzylidene, J=8 Hz/ J=4 Hz), 7.72-7.77 (2H, m, H5, H7-quinolone, J=8 Hz/ J=1 Hz), 7.86-7.88 (1H, m, H8-quinolone, J = 8 Hz/1 Hz), 8.57 (1H, s, H-C=N); 8.80 (1H, s, H2-quinolone), 12.95 (1H, s, NH-amide), 13.53 (1H, s, NH-quinolone); 13C-NMR (DMSO-d6, 100 MHz) δ ppm 56.22, 70.00, 106.45, 126.04, 128.14, 128.38, 128.97, 137.27, 155.94, 158.12, 175.30; Anal. Calcd for C25H21N3O4: C, 70.25; H, 4.95; N, 9.83. Found: C, 70.41; H, 5.11; N, 10.02.
Molecular modeling study
The active compound 21 was selected for docking studies against HCV NS5B. 1GX6 was used for binding mode analysis of NS5B inhibitory activity. All the compounds were built and subsequently optimized using the HyperChem 8.0 software (34). The protein structure was prepared for docking using AutoDock tools 1.5.6 from MGL Tools package (35). Docking was performed by Autodock VINA program (36).
Co-crystallized ligand and all water molecules were removed from crystal protein while manganese ions (Mn2+) at the active site of HCV NS5B were remained. Polar hydrogens were added and non-polar hydrogens were merged, finally, Kollman united atom charge and atom type parameter was added to 1GX6. Grid map dimensions (20×20×20) were set surrounding active site.
Cell Culture and virus production
Huh7.5 cells originated from hepatocellular carcinoma which has been shown to support efficient HCV replication and production (kindly provided by Rice C) was cultured in Dulbecco′s modified Eagle′s medium (DMEM) supplemented with 10% fetal bovine serum (FBS), penicillin (100 U/mL) and streptomycin (100 μg/mL).
The plasmid encoding the full genome of Japonicum Fulminant Hepatitis virus serotype 1 (JFH-1) strain (kindly provided by Wakita T) was used for in-vitro transcription to produce approximately high titer stocks of cell culture-produced virus (HCVcc) as previously described (37).
RT-qPCR assay
Huh7.5 cells were seeded into 48-well plates at a density of 3×105 cells per well with DMEM containing 10% FBS. After 24 h, huh7.5 cells were infected with HCV viral stock (105 IU/mL) for 3 h, at which point the infection was stopped and each well was washed three times by sterile PBS to removed non-penetrated virions and then the fresh medium was added to each well. Subsequently, the infected cells were treated with newly synthesized compounds at different concentrations and the sofosbuvir (Sigma-Aldrich, USA) as a positive control. After three days inoculation, the supernatant medium was collected and the viral RNA was extracted using viral QIAamp viral RNA mini kit (Qiagen, Düsseldorf, Germany), according to the manufacturer protocol. Then, the amount of HCV replicon RNA was determined using Quantitative RT-PCR assay by hepatitis C virus viral load kit (Gene Proof, Czech Republic, with CE, IVD) according to the manufacturer’s instruction.
The 50% effective concentration (EC50) was determined as the ratio of the normalized HCV RNA amount in treated samples relative to the controls (the samples without compound treatment) using GraphPad Prism5.0 software (GraphPad Software, San Diego, CA).
Cytotoxicity Assay
The cytotoxicity of compounds to Huh-7.5 cells was measured in parallel with the HCV replication assay based on the cleavage of the yellow tetrazolium salt XTT® (sodium 3-[1 (phenylaminocarbonyl)-3, 4-tetrazolium]-bis(4-methoxy-6-nitro)benzene sulfonic acid) to generate an orange formazan dye by metabolic function of alive and active cells. Cells were treated with various concentrations (500, 250, 100, 25, and 12 μm/mL) of compounds and incubated for 72 hours.
The cell cytotoxicity was determined using XTT proliferation assay kit (Sigma-Aldrich) following the manufacturer’s instructions. The 50% cytotoxic concentration (CC50) was defined as the concentration of compound that reduced cell proliferation by 50%.
Results and Discussion
Chemistry
Target compounds 13-25 were prepared in good yields (70-98%) from the acetaminophen as a starting material as shown in scheme 1. Starting from benzylation of acetaminophen (7) with benzyl bromide followed by basic hydrolysis of acetamide group gave the O-benzyl aniline (9). The key quinolone intermediate (11) was synthesized via the well-known Gould–Jacobs approach (38). This procedure began with condensation of O-benzyl aniline (9) with ethoxymethylenemalonate diethyl ester (EMME) to produce malonate intermediate (10), which upon intramolecular cyclization afforded the ethyl quinolone-3-carboxylate (11). The resulting ester was converted to carbohydrazide analogue (12) via reaction with hydrazine hydrate. N′-Arylidene fragment in target compounds (13-25) were introduced through reaction of carbohydrazide intermediate 12 with substituted benzaldehydes. The structure of the synthesized compounds was confirmed by IR, 1H-NMR, and ESI-MS.
HCV replicon assay
Target compounds 13-25 were evaluated in a cell-based viral replication surrogate assay known as the replicon system (39). We hypothesized that our quinolone analogues will inhibit NS5B, likely via binding to the active site. To test this hypothesis, FDA-approved active site binding NI, sofosbuvir, was used as a control compound. In parallel, compounds were also evaluated for their cytotoxic effects in the same cell line to ensure that the activity is completely devoid of cytotoxicity effects. The biological results are expressed as EC50, CC50 and SI (selectivity index, given by the CC50/EC50 ratio).
As shown in Table 1, some of the target compounds were found active against HCV with the EC50 values in the range from 35 to 120 µM. Furthermore, except for compound 24, all of these active compounds showed SI > 2, rendering them suitable as an inhibitor scaffold for HCV. The anti-HCV assay results revealed a few discernible structure-activity relationship (SAR) trends. First of all, introduction a suitable substituent on the phenyl ring of compounds was found to greatly impact potency as compound 13 with an unsubstituted phenyl was inactive (EC50>200 µM) whereas a number of analogues with substituted phenyl showed significant activity (compounds 20-24). Secondly, the identity of substituent also appeared crucial for inhibition as among active compounds, anti-HCV activity decreased in the following order: Cl > Me > F > OMe > SMe. Thirdly, position of substituent on the phenyl ring influenced the anti-HCV activity as for nearly all substituents, ortho- or meta-position of the phenyl ring was tolerated better than para-position. Notably, compound 21 with Cl group in meta-position (EC50 = 35 µM) was proven to be considerably more potent than its para-counterpart (16, EC50 > 200 µM). In compounds with methyl substitution, changing from ortho- to meta-position increased the both activity (EC50 = 70 and 45 µM, respectively) and cytotoxicity (CC50 = 160 and 45 µM, respectively). Regarding the similarity of EC50 and CC50 of compound 22, it seems that its anti-HCV activity was result of cytotoxicity. Compounds 20 and 24 having, respectively, 2-fluoro and 3-methoxy substitutions displayed similar activities with an EC50 = 120 µM. These differences in activity and toxicity between substituents and their positions may reflect a fundamental change in their binding mode to the protein target or physicochemical properties, although further investigation is required to determine the structure–activity relationships in detail.
Overall, the best activities were observed with 3-chloro (compound 21, EC50 = 35 µM) and 2-methyl (compound 23, EC50 = 70 µM) substituents, suggesting that designed scaffold has the potential for antiviral discovery against HCV. Further optimization may be required to address the potency of compounds.
EC50, CC50 and TI for newly - synthesized compounds
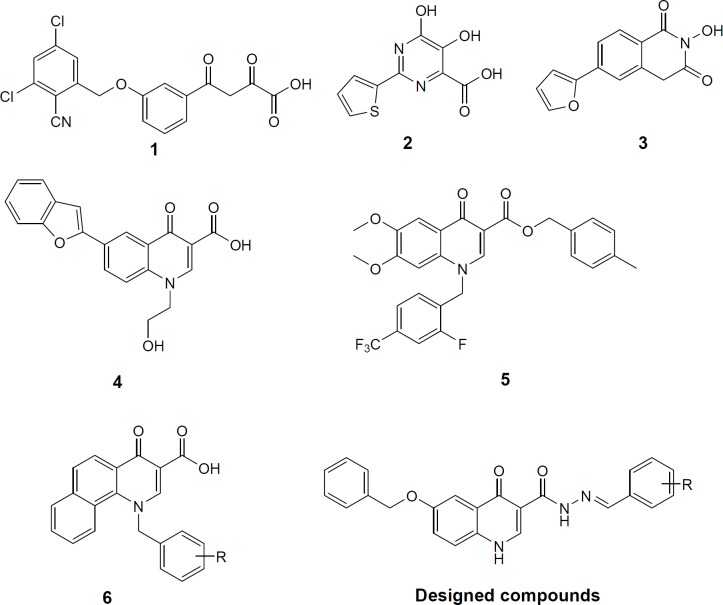
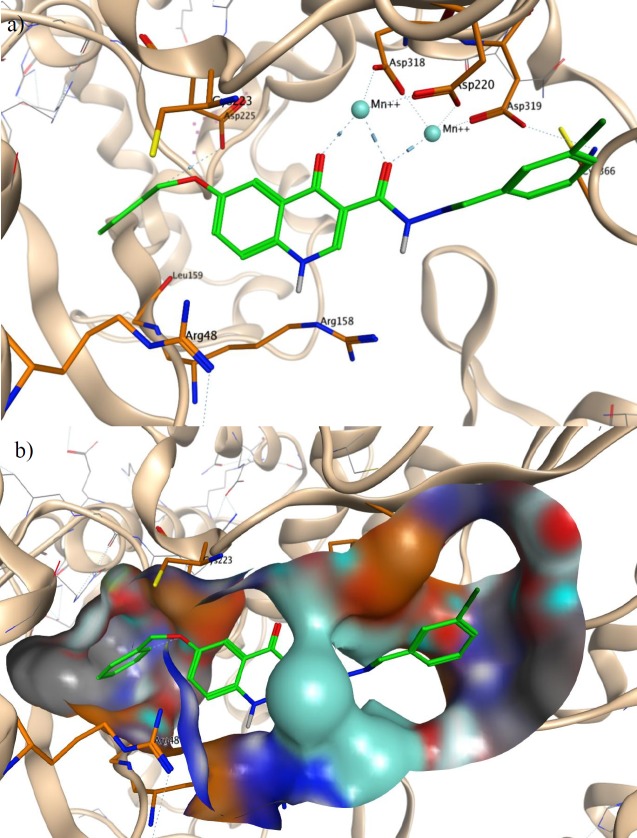
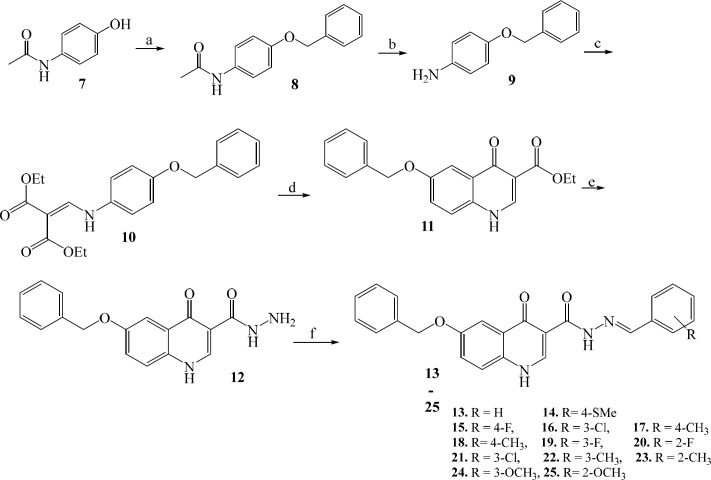
Molecular Modeling Study
Our compounds were designed based on pharmacophore model of NS5B active site inhibitors. Therefore, we generated a docking model to investigate binding modes of designed compounds with NS5B active site. Docking model was generated on the basis of the existing X-ray crystallographic structure of NS5B polymerase complexed with uridine triphosphate (UTP) and divalent metal ions (PDB code: 1GX6) (40). The most active compound observed in anti-HCV assay, compound 21 was docked into the binding pocket of NS5B active site after the removal of the original UTP. As shown in Figure 4, compound 21 interacted with two Mn2+ that are coordinated by active site residues Asp220, Asp318 and Asp319. The Mn2+ ions were chelated through two carbonyl groups of quinolone scaffold with the distances of 2.76, 2.37 and 2.42 Å. The O-benzyl moiety of compound 21 was inserted into a hydrophobic pocket in the enzyme consisting of Leu159 and Cys223. Leu159 is an important residue in interaction with the pyrimidine base of co-crystalized UTP. Oxygen atom of O-benzyl formed a hydrogen bond to the side chain of Asp225 with the distance of 3.76 Å, the same residue that is bonded to 2′-hydroxy group of UTP. In addition, NH group of quinolone scaffold formed interactions with side chain of Arg158 and Arg48. An extra hydrophobic bond was found between 3-chlorophenyl group of compound 21 and Cys366. These observed interactions are in agreement with reported studies on docking of NS5B active site inhibitors (41-43). So anti-HCV activity of synthesized compounds may be due to NS5B inhibition.
Conclusion
In this study, in order to identify new scaffold as anti-HCV agents, we designed and synthesized new N′-arylidene-6-(benzyloxy)-4-oxo-1,4-dihydroquinoline-3-carbohydrazide derivatives. Results of cell-based HCV replicon assay revealed that several compounds (20, 21, 22, 23, and 24) exhibited moderate to good activity with EC50 values ranging from 35 to 120 µM. Molecular modeling studies suggested that the designed compounds might interact with two magnesium ions and other key residues in the NS5B polymerase active site. These results make the designed scaffold a promising starting point for development of more active compounds in future.
References
-
1.
Bosetti C, Levi F, Lucchini F, Zatonski WA, Negri E, La Vecchia C. Worldwide mortality from cirrhosis: An update to 2002. J. Hepatol. 2007;46:827-39. [PubMed ID: 17336419].
-
2.
Modi AA, Liang TJ. Hepatitis C: a clinical review. Oral Dis. 2008;14:10-4. [PubMed ID: 18173443].
-
3.
-
4.
Neukam K, Macías J, Mira JA, Pineda JA. A review of current anti-HCV treatment regimens and possible future strategies. Expert Opin. Pharmacother. 2009;10:417-33. [PubMed ID: 19191679].
-
5.
Alexopoulou A, Karayiannis P. Interferon-based combination treatment for chronic hepatitis C in the era of direct acting antivirals. Ann. Gastroenterol. 2015;28:55-65. [PubMed ID: 25608803].
-
6.
Lim TR, Tan BH, Mutimer DJ. Evolution and emergence of a new era of antiviral treatment for chronic hepatitis C infection. Int. J. Antimicrob. Agents. 2014;43:17-25. [PubMed ID: 24183752].
-
7.
Zajac M, Muszalska I, Sobczak A, Dadej A, Tomczak S, Jelinska A. Hepatitis C - New drugs and treatment prospects. Eur. J. Med. Chem. 2019;165:225-49. [PubMed ID: 30685524].
-
8.
Asselah T, Marcellin P. Direct acting antivirals for the treatment of chronic hepatitis C: one pill a day for tomorrow. Liver Int. 2012;32:88-102. [PubMed ID: 22212578].
-
9.
Schinazi R, Halfon P, Marcellin P, Asselah T. HCV direct-acting antiviral agents: the best interferon-free combinations. Liver Int. 2014;34:69-78.
-
10.
Gerber L, Welzel TM, Zeuzem S. New therapeutic strategies in HCV: polymerase inhibitors. Liver Int. 2013;33:85-92. [PubMed ID: 23286851].
-
11.
Shih IH, Vliegen I, Peng B, Yang H, Hebner C, Paeshuyse J, Purstinger G, Fenaux M, Tian Y, Mabery E, Qi X, Bahador G, Paulson M, Lehman LS, Bondy S, Tse W, Reiser H, Lee WA, Schmitz U, Neyts J, Zhong W. Mechanistic characterization of GS-9190 (Tegobuvir), a novel nonnucleoside inhibitor of hepatitis C virus NS5B polymerase. Antimicrob. Agents Chemother. 2011;55:4196-203. [PubMed ID: 21746939].
-
12.
Bressanelli S, Tomei L, Rey FA, De Francesco R. Structural analysis of the hepatitis C virus RNA polymerase in complex with ribonucleotides. J. Virol. 2002;76:3482-92. [PubMed ID: 11884572].
-
13.
Appleby TC, Perry JK, Murakami E, Barauskas O, Feng J, Cho A, Fox D, Wetmore DR, McGrath ME, Ray AS. Structural basis for RNA replication by the hepatitis C virus polymerase. Science. 2015;347:771-5. [PubMed ID: 25678663].
-
14.
Beaulieu P, Tsantrizos Y. Inhibitors of the HCV NS5B polymerase: new hope for the treatment of hepatitis C infections. Curr. Opin. Investig. Drugs. 2004;5:838-50.
-
15.
Boyce SE, Tirunagari N, Niedziela-Majka A, Perry J, Wong M, Kan E, Lagpacan L, Barauskas O, Hung M, Fenaux M. Structural and regulatory elements of HCV NS5B polymerase–β-loop and C-terminal tail–are required for activity of allosteric thumb site II inhibitors. PLoS One. 2014;9:e84808. [PubMed ID: 24416288].
-
16.
Powdrill MH, Bernatchez JA, Götte M. Inhibitors of the hepatitis C virus RNA-dependent RNA polymerase NS5B. Viruses. 2010;2:2169-95. [PubMed ID: 21994615].
-
17.
Hajimahdi Z, Zarghi A. Progress in HIV-1 integrase inhibitors: A review of their chemical structure diversity. Iran. J. Pharm. Res. 2016;15:595. [PubMed ID: 28243261].
-
18.
Summa V, Petrocchi A, Matassa VG, Taliani M, Laufer R, De Francesco R, Altamura S, Pace P. HCV NS5b RNA-dependent RNA polymerase inhibitors: from α,γ-diketoacids to 4,5-dihydroxypyrimidine-or 3-methyl-5-hydroxypyrimidinonecarboxylic acids. Design and synthesis.J. Med. Chem. 2004;47:5336-9. [PubMed ID: 15481971].
-
19.
Koch U, Attenni B, Malancona S, Colarusso S, Conte I, Di Filippo M, Harper S, Pacini B, Giomini C, Thomas S, Incitti I, Tomei L, De Francesco R, Altamura S, Matassa VG, Narjes F. 2-(2-Thienyl)-5,6-dihydroxy-4-carboxypyrimidines as Inhibitors of the Hepatitis C Virus NS5B Polymerase: Discovery, SAR, Modeling, and Mutagenesis. J. Med. Chem. 2006;49:1693-705. [PubMed ID: 16509585].
-
20.
Chen YL, Tang J, Kesler MJ, Sham YY, Vince R, Geraghty RJ, Wang Z. The design, synthesis and biological evaluations of C-6 or C-7 substituted 2-hydroxyisoquinoline-1,3-diones as inhibitors of hepatitis C virus. Bioorg. Med. Chem. 2012;20:467-79. [PubMed ID: 22100256].
-
21.
Kumar DV, Rai R, Brameld KA, Somoza JR, Rajagopalan R, Janc JW, Xia YM, Ton TL, Shaghafi MB, Hu H. Quinolones as HCV NS5B polymerase inhibitors. Bioorg. Med. Chem. Lett. 2011;21:82-7. [PubMed ID: 21145235].
-
22.
Chen Y-L, Zacharias J, Vince R, Geraghty RJ, Wang Z. C-6 aryl substituted 4-quinolone-3-carboxylic acids as inhibitors of hepatitis C virus. Bioorg. Med. Chem. 2012;20:4790-800. [PubMed ID: 22748708].
-
23.
Cheng Y, Shen J, Peng RZ, Wang GF, Zuo JP, Long YQ. Structure-based optimization and derivatization of 2-substituted quinolone-based non-nucleoside HCV NS5B inhibitors with submicromolar cellular replicon potency. Bioorg. Med. Chem. Lett. 2016;26:2900-6. [PubMed ID: 27133482].
-
24.
Daneshtalab M, Ahmed A. Nonclassical biological activities of quinolone derivatives. J. Pharm. Pharm. Sci. 2011;15:52-72.
-
25.
Hajimahdi Z, Ranjbar A, Suratgar AA, Zarghi A. QSAR Study on anti-HIV-1 activity of 4-oxo-1,4-dihydroquinoline and 4-oxo-4H-pyrido[1,2-a]pyrimidine derivatives using SW-MLR, artificial neural network and filtering methods. Iran. J. Pharm. Res. 2015;14 (Supplement):69-75. [PubMed ID: 26185507].
-
26.
Hajimahdi Z, Zabihollahi R, Aghasadeghi MR, Ashtiani SH, Zarghi A. Novel quinolone-3-carboxylic acid derivatives as anti-HIV-1 agents: design, synthesis, and biological activities. Med. Chem. Res. 2016;25:1861-76.
-
27.
Hajimahdi Z, Zabihollahi R, Aghasadeghi MR, Zarghi A. Design, synthesis and docking studies of new 4-hydroxyquinoline-3-carbohydrazide derivatives as anti-HIV-1 agents. Drug Res. 2013;63:192-7.
-
28.
Hajimahdi Z, Zarghi A, Zabihollahi R, Aghasadeghi MR. Synthesis, biological evaluation, and molecular modeling studies of new 1,3,4-oxadiazole- and 1,3,4-thiadiazole-substituted 4-oxo-4H-pyrido[1,2-a]pyrimidines as anti-HIV-1 agents. Med. Chem. Res. 2013;22:2467-75.
-
29.
Parizadeh N, Alipour E, Soleymani S, Zabihollahi R, Aghasadeghi MR, Hajimahdi Z, Zarghi A. Synthesis of novel 3-(5-(alkyl/arylthio)-1,3,4-oxadiazol-2-yl)-8-phenylquinolin-4(1H)-one derivatives as anti-HIV agents. Phosphorus Sulfur Silicon Relat. Elem. 2018;193:225-31.
-
30.
Faghihi K, Safakish M, Zebardast T, Hajimahdi Z, Zarghi A. Molecular Docking and QSAR Study of 2-Benzoxazolinone, Quinazoline and Diazocoumarin Derivatives as Anti-HIV-1 Agents. Iran. J. Pharm. Res. 2019;18:1253-63.
-
31.
Safakish M, Hajimahdi Z, Zabihollahi R, Aghasadeghi MR, Vahabpour R, Zarghi A. Design, synthesis, and docking studies of new 2-benzoxazolinone derivatives as anti-HIV-1 agent. Med. Chem. Res. 2017;26:2718-26.
-
32.
Livani ZA, Safakish M, Hajimahdi Z, Soleymani S, Zabihollahi R, Aghasadeghi MR, Alipour E, Zarghi A. Design, synthesis, molecular modeling, in silico ADME studies and anti-HIV-1 assay of new diazocoumarin derivatives. Iran. J. Pharm. Res. 2018;17:65-77. [PubMed ID: 31011343].
-
33.
Hajimahdi Z, Zabihollahi R, Aghasadeghi MR, Zarghi A. Design, Synthesis, Docking Studies and Biological Activities Novel 2,3- Diaryl-4-Quinazolinone Derivatives as Anti-HIV-1 Agents. Curr. HIV Res. 2019;17:214-22. [PubMed ID: 31518225].
-
34.
HyperChem(TM) Professional 8.0, Hypercube, Inc. 1115 NW 4th Street, Gainesville. Florida 32601, USA;
-
35.
Morris GM, Goodsell DS, Halliday RS, Huey R, Hart WE, Belew RK, Olson AJ. Automated Docking Using a Lamarckian Genetic Algorithm and and Empirical Binding Free Energy Function. J. Comput. Chem. 1998;19:1639-62.
-
36.
Trott O, Olson AJ. AutoDock Vina: improving the speed and accuracy of docking with a new scoring function, efficient optimization, and multithreading. J. Comput. Chem. 2010;31:455-61. [PubMed ID: 19499576].
-
37.
Wakita T, Pietschmann T, Kato T, Date T, Miyamoto M, Zhao Z, Murthy K, Habermann A, Kräusslich H-G, Mizokami M. Production of infectious hepatitis C virus in tissue culture from a cloned viral genome. Nat. Med. 2005;11:791-6. [PubMed ID: 15951748].
-
38.
Gould Jr R, Jacobs W. The synthesis of certain substituted quinolines and 5,6-benzoquinolines. J. Am. Chem. Soc. 1939;61:2890-5.
-
39.
Blight KJ, Kolykhalov AA, Rice CM. Efficient initiation of HCV RNA replication in cell culture. Science. 2000;290:1972-4. [PubMed ID: 11110665].
-
40.
Bressanelli S, Tomei L, Rey FA, De Francesco R. Structural analysis of the hepatitis C virus RNA polymerase in complex with ribonucleotides. J. Virol. 2002;76:3482-92. [PubMed ID: 11884572].
-
41.
Deore RR, Chen GS, Chang PT, Chern TR, Lai SY, Chuang MH, Lin JH, Kung FL, Chen CS, Chiou CT, Chern JW. Discovery of N-arylalkyl-3-hydroxy-4-oxo-3,4-dihydroquinazolin-2-carboxamide derivatives as HCV NS5B polymerase inhibitors. ChemMedChem. 2012;7:850-60. [PubMed ID: 22383290].
-
42.
Bhatt A, Gurukumar KR, Basu A, Patel MR, Kaushik-Basu N, Talele TT. Synthesis and SAR optimization of diketo acid pharmacophore for HCV NS5B polymerase inhibition. Eur. J. Med. Chem. 2011;46:5138-45. [PubMed ID: 21893371].
-
43.
Zhong D, Liu M, Cao Y, Zhu Y, Bian S, Zhou J, Wu F, Ryu KC, Zhou L, Ye D. Discovery of Metal Ions Chelator Quercetin Derivatives with Potent Anti-HCV Activities. Molecules. 2015;20:6978-99. [PubMed ID: 25913935].