Abstract
Keywords
Cardiosphere-derived cells Exosomes Hypoxia preconditioning Anti-apoptotic effect
Introduction
Cardiovascular disease is one of the leading pathological causes of mortality worldwide (1). Cardiosphere-derived cells (CDCs) currently are in phase 2 clinical trials to reverse post- myocardial infarction (MI) injury. The results of studies in various animal models and also a phase 1 human study have shown that the CDCs have the ability to decrease scar mass, increase viable mass, and halt adverse remodeling (2). It was demonstrated that exosomes secreted by CDCs replicate the cardioprotective and regenerative effects of CDCs, including anti-apoptotic effect (3).
Exosomes, 30-150 nm in diameter lipid bilayer vesicles, are secreted by many cell types and contain a wide range of functional proteins, mRNAs, and miRNA. They are the key transporters of paracrine factors and are able to mediate cell–cell communication. (4). Exosomes as therapeutic agent for repairing damaged myocardium could overcome many obstacles associated with stem cell-based therapy (3, 5).
Immediately after coronary arterial occlusion in acute myocardial infarction, cardiomyocytes are stressed by hypoxia which leads to undergo apoptosis (6). Hypoxia-inducible factor-1 (HIF-1) is the master regulator of the cellular adaption to hypoxic stress (7).
Recent reports suggested that hypoxia as an in-vitro environmental stressors can modify the composition of cardiac progenitor cell-derived exosomes (CPC-exo). These studies concluded that hypoxia have beneficial effect on the cardiac response through paracrine signaling (8, 9).
In the present study, we aimed to investigate the anti-apoptotic effect of CDCs exosomes in cardiomyocytes protection against CoCl2-induced apoptosis. We assessed the anti-apoptotic effect of the isolated exosomes from the media of hypoxia (1% O2)- and normoxia- treated CDCs on human embryonic stem cell-derived cardiomyocytes (hESC-CMs) in terms of caspase-3/7 activation.
Experimental
Cell culture
Human CDCs (obtained from Iranian pediatric patients diagnosed with a congenital heart disease) were provided by Royan Cell Bank Services. The Patientsꞌ parents gave their informed consent for study participation and research use. Ethical approval was granted by the Royan institute Ethical Committee (10). CDCs were then cultured in proliferation medium [Iscoveꞌs Modified Dulbeccoꞌs Medium (IMDM), Sigma, USA] supplemented with 1% L-glutamine (Invitrogen, USA), 1% penicillin/streptomycin (Invitrogen, USA), 10% fetal bovine serum (FBS, Gibco, USA) with 10 ng/mL basic fibroblast growth factor (bFGF, Royan Biotech) at 37 ºC and 5% CO2 in 95% humidity.
Cardiac differentiation in static suspension culture
For hESC-CM production in static suspension culture, 5-day-old hESC spheroids with the size of 175 ± 25 µm were transferred to 60 mm nonadhesive bacterial plates (Sigma-Aldrich, USA) in 5 mL of differentiation medium (DM) which contains Roswell Park Memorial Institute (RPMI) 1640 medium (Gibco, USA) supplemented with 2% B27 without retinoic acid (Gibco, USA), 0.1 mM β-mercaptoethanol, 2 mM L-glutamine, 1% nonessential amino acid. First, the aggregates were treated for one day with 12 μM CHIR99021, a glycogen synthase kinase 3-β inhibitor. Next, spheroids were washed with Dulbecco’s phosphate-buffered saline (DPBS) and were transferred to DM without CHIR99021 for one day. In the next step, the spheroids were transferred to DM containing 5-µM purmorphamine (Stemgent, USA) as the sonic hedgehog agonist, 5 µM IWP2 (Tocris Bioscience, UK) as a Wnt antagonist, 5 µM SB431542 (Sigma-Aldrich, USA) as the inhibitor of transforming growth factor beta (TGF-β) super family type I activin receptor-like kinase receptors. The aggregates were maintained in the medium for two days. On day five, the medium was changed and the spheres transferred to DM after they had been washed with DPBS. The medium was changed every two days. On the seventh day, beating started and reached its highest on the tenth day.
Exosome purification
Exosomes were removed from FBS by ultracentrifugation at 120,000 ×g (Type 45 Ti rotor, 32128 rpm, k-factor 133, L-100XP ultracentrifuge, Beckman Coulter, USA) for 18 hours. After discarding the pellet, the supernatant of FBS was filtered through 0.2 µm filters (Techno Plastic Products, Switzerland) and then used in cell cultures (11). Then, CDCs at the fifth passage were cultured in complete media containing IMDM, 10% exosome-depleted FBS, 1% penicillin-streptomycin, and 1% L-glutamine under normoxic (18% O2, 5% CO2) and hypoxic (1% O2, 5% CO2 and 94% N2) conditions in two distinct incubator (Labotec C200, Germany). The conditioned media was collected 48 h later, and then the exosomes were harvested by differential ultracentrifugation (12, 13). The purified exosome pellet was resuspended in 200 μL PBS and stored at -80 °C. The protein content of the exosome suspension was analyzed by Pierce™ BCA Protein Assay kit (Thermo Scientific, USA). The size of exosomes was determined by dynamic light scattering (DLS) with a Zetasizer nanoseries instrument (Malvern Nano-Zetasizer, UK). The morphological characteristics of exosomes were observed under scanning electron microscopy (SEM, KYKY-EM3200, USA) and flow cytometry was used to analyze surface protein markers of exosomes (detailed explanation of these methods and their results will be reported elsewhere).
Immunostaining
hESC-CMs were obtained using a protocol described previously (14). To confirm the differentiation of hESC-CM, cardiac specific markers were stained. To achieve dissociated single cardiomyocytes, the beating spheroids at day 14 of differentiation were washed and maintained in DPBS for 5 min. Then, Accumax cell dissociation solution (Sigma, USA) was added and incubated for 10 min. After that, 5×104 cells/well of the single cardiomyocytes were seeded into 4-well matrigel-coated plates contained fresh DM. Two days later, after washing with DPBS, the attached cells were fixed with 4% (w/v) paraformaldehyde for 20 min at 4 °C and washed with PBS/0.1% Tween 20. Then, the cells were permeabilized with 0.5% Triton X-100 in DPBS for 30-45 min at room temperature. Blocking was performed with 5% (v/v) goat serum for 1h. Next, the cells were incubated with diluted primary antibodies (1:100) in blocking buffer overnight at 4 °C. Primary antibodies used were antibodies against cardiac specific markers: cardiac troponin T (cTnT, Abcam, UK), myosin light chain 2v (MLC2v, Santa Cruz, USA), actinin (Sigma, USA). After three rounds of washing with PBS/0.1% Tween 20 for 5 min each time, the cells were incubated with secondary antibodies [Alexa Fluor 488 goat anti-mouse IgG antibody (Abcam, UK) Alexa Fluor 546 goat anti-mouse IgG antibody (Abcam, UK)] at a dilution of 1:500 in blocking buffer for 45 min at room temperature. Finally, the cardiomyocytes were washed with PBS/0.1% Tween 20 three times. 4ʹ, 6-diamidino-2-phenylindole (DAPI) was used to stain the nuclei for 5-10 min at room temperature. The cells were examined using fluorescence microscopy (Olympus, IX71, USA). The positive hESC-CMS for cardiac specific markers were counted manually in at least five images from different areas of each sample in three independent experiments.
Apoptosis induction
Cobalt chloride (CoCl2, Sigma, USA) was used for induction of apoptosis in hESC-CMs. The appropriate concentration of CoCl2 in order to induce apoptosis in the hESC-CMs was determined. In brief, hESC-CMs were seeded on 3 cm2 matrigel-coated plates. Two days later, the cells were treated with different concentrations of CoCl2 (1, 2 and 3 mM) for 3 h (3, 15, 16). Then, the caspase-3/7 activity was measured using CellEvent® Caspase-3/7 Green Ready Probes® Reagent (Life technologies, USA) according to manufacturer’s instructions. The samples were analyzed by the flow cytometer (FACS Calibur; BD Biosciences, USA) using Flowing software, version 2.5.1 (BD Biosciences, USA).
In-vitro apoptosis assay
In order to determine the anti-apoptotic effect of exosomes, hESC-CMs were cultured on 3 cm2 matrigel-coated plates. 24 h later, the cells were treated with 10, 25 and 50 μg/mL normoxic as well as hypoxic exosomes. Apoptosis was induced in hESC-CM by addition of a selected CoCl2 concentration after 24 h. The activity of caspase-3/7 was measured 3 h later as described earlier. Apoptosis-induced hESC-CMs with no treatment were used as the positive control of apoptosis induction.
Statistical Analysis
GraphPad Prism software (version 6, USA) was used for statistical analyses. Two independent groups were compared using unpaired student’s T-test. One-way ANOVA followed by Tukey post-test was used to perform multiple group comparisons. The differences with a p <0.05 were determined to be statistically significant.
Results
CDCs-derived exosome isolation and characterization
CDCs were cultured under hypoxic or normoxic conditions and the exosomes were isolated from conditioned media by ultracentrifugation after 48 h. The DLS analysis was used to define the size of these exosomes. The mean hydrodynamic diameter of exosomes was between 150–170 nm. Under SEM, the exosomes exhibited a round morphology. The flow cytometry analysis of N-exo and H-exo showed that CD63 and CD81 which are typical exosomal markers, were expressed on the surface of exosomes (Unpublished data).
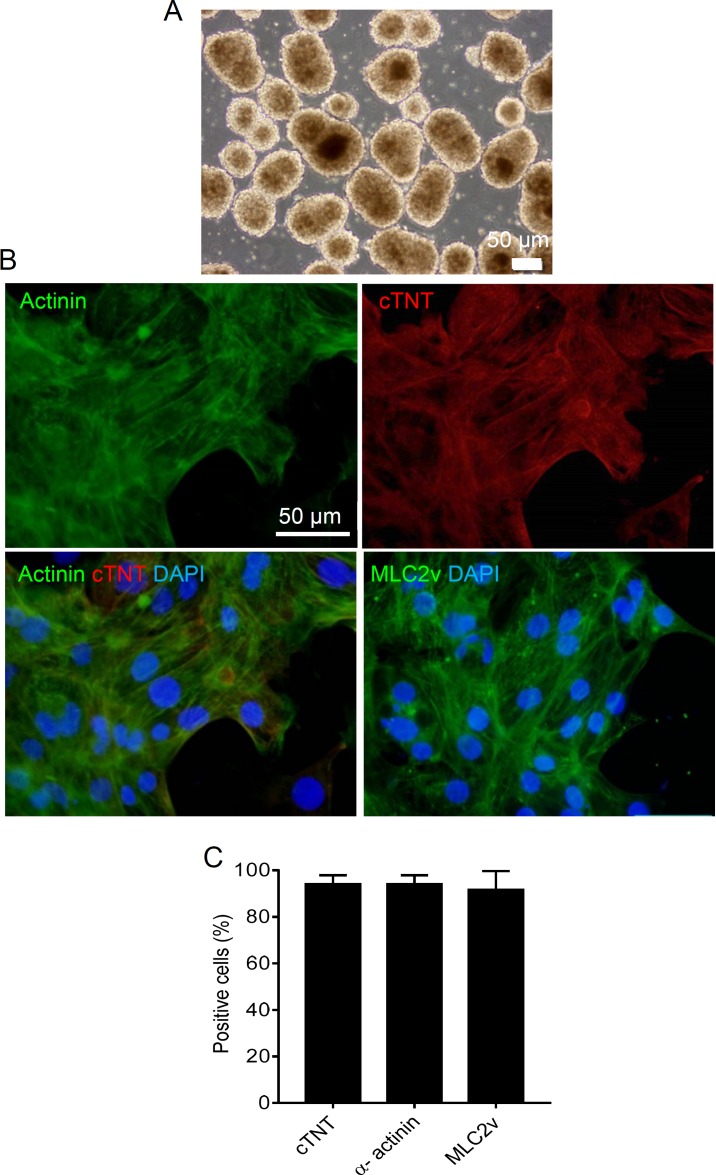
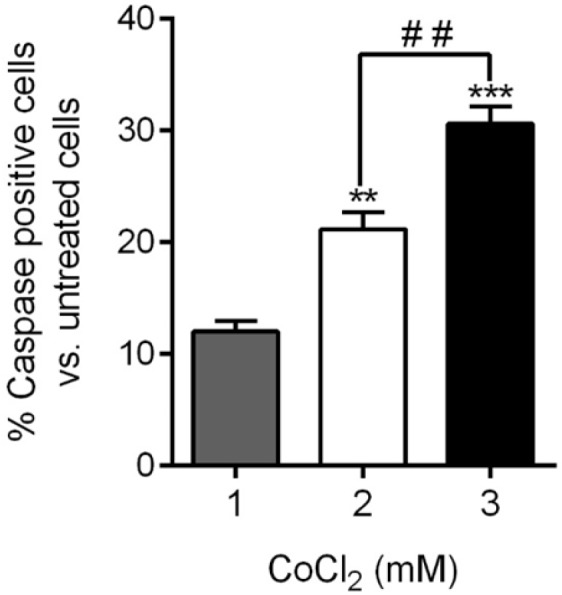
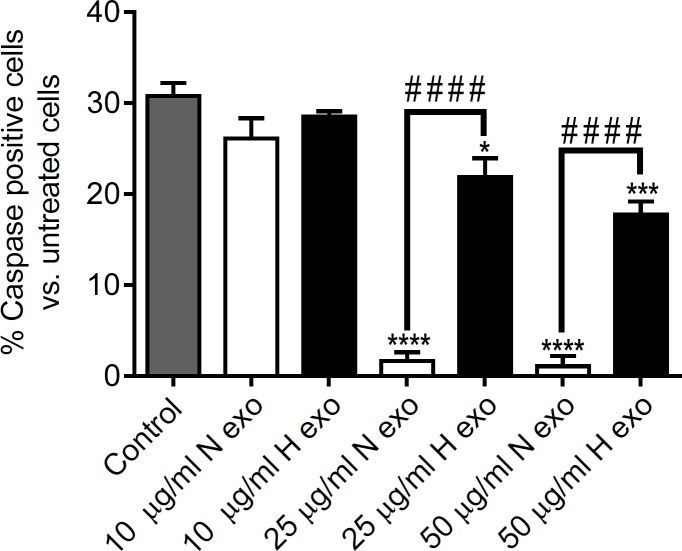
Characterization of hESC-CMs
The cardiogenic differentiation efficiency was determined by counting the number of beating spheroids at day 10 after the onset of differentiation using an inverted cell culture microscope (Figure 1 A). Approximately 100% of spheroids were beating (see supplementary video online). The 14-day-old beating spheroids were subsequently collected and dissociated for immunostaining of cardiac-specific markers; Actinin, MLC2v and cTnT (Figure 1B). hESC-CMs were approximately 90% positive for the tested cardiac specific markers (Figure 1C).
CoCl2-induced apoptosis in hESC-CMs
To determine apoptogenic concentration of CoCl2 in hESC-CMs, the caspase-3/7 activity was measured in the presence of different concentrations of CoCl2 (1, 2 and 3 mM). The analysis showed that treatment of the cells with 3 mM CoCl2 for 3 h resulted in 30.63 ± 2.66% cell apoptosis in terms of caspase-3/7 activation. As indicated in Figures. 2, 3 mM CoCl2 resulted in significant higher caspase positive cells comparing to than 1 and 2 mM (3 mM vs. 2 mM p <0.01, 3 mM vs. 1 mM p <0.001).
The effect of exosomes-derived CDCs under hypoxic and normoxic conditions on induced apoptosis in hESC-CMs
The effect of hypoxic (H-exo) and normoxic exosomes (N-exo) on CoCl2-induced apoptosis was investigated. Accordingly, hESC-CMs were treated with N-exo and H-exo at different concentrations (10, 25 and 50 μg/mL) for 24 h and then the caspase-3/7 activity was measured after apoptosis induction wih CoCl2. We found that both N-exo and H-exo inhibited hESC-CMs CoCl2-induced apoptosis (Figure. 3). N-exo at concentrations of 25 and 50 μg/mL significantly reduced caspase-positive cells as compared to untreated cells [N-exo (25 and 50 μg/mL) vs. control, p <0.0001]. H-exo resulted in significant anti-apoptotic activity at concentration of 25 and 50 μg/mL compared to control [N-exo (25 μg/mL) vs. control, p <0.05; N-exo (50 μg/mL) vs. control, p <0.001]. Both N-exo and H-exo caused no significant change in anti-apoptotic effects at the concentration of 10 μg/mL (Figure 3). The anti-apoptotic activity of N-exo at concentrations of 25 and 50 μg/mL was significantly higher from that of H-exo at these concentrations [N-exo (25 μg/mL) vs. H-exo (25 μg/mL), p <0.0001; N-exo (50 μg/mL) vs. H-exo (50 μg/mL), p <0.0001].
Discussion
CDCs have shown to promote cardiac regeneration of the infracted human heart (2, 17). Exosomes generated by CDCs are beneficial paracrine signals that reproduce CDC-induced therapeutic regeneration. They are sufficient to mediate the entire effect of CDCs (3, 5). Few studies have investigated the therapeutic potential of CDC-exosomes in animal MI models and some other cardiovascular diseases. These studies have shown that exosomes secreted by CDCs replicate the cardioprotective and regenerative effects of CDCs such as apoptosis inhibition of cardiomyocytes. Gallet and coworkers indicated that CDC-derived exosomes delivered by intramyocardial (IM) injection has the ability to decrease acute ischaemia-reperfusion injury, halt adverse remodeling and to improve LVEF in pig models of acute (AMI) and convalescent myocardial infarction (CMI) (2). In the study of Ibrahim et al., exosomes secreted by human CDCs inhibit apoptosis and promote proliferation of cardiomyocytes, while enhancing angiogenesis. Injection of exosomes into injured mouse hearts recapitulates the regenerative and functional effects produced by CDC transplantation, whereas inhibition of exosome production by CDCs blocks those benefits (3). All of these data confirmed in-vivo anti-apoptotic effect of CDC-exosomes (2, 3, 18).
In all of these studies, CDC-exosomes were obtained under normoxic conditions, which likely could not reflect the state of post-infarct tissue. While most in-vitro cultured cells maintained at oxygen levels of approximately 20%, natural cell micro-environments seem to have much lower oxygen tensions with considerable variation based on location. For instance the mean oxygen concentration of arterial blood is approximately 12%, and that of tissue is 3% (19). Adult stem cells similarly live under hypoxic conditions of 3-5% O2in-vivo and these hypoxic conditions are the physiological norms for a variety of stem cell niches (20). These studies have shown that the level of oxygen play a crucial role in the maintenance, differentiation, and function of stem cells. Nevertheless, hypoxia can also induce mitochondria-mediated apoptosis and subsequent caspases activation in bone marrow-derived mesenchymal stem cells (21). In this study we have analyzed the anti-apoptotic activity of exosomes generated by CDCs under both normoxic and hypoxic conditions. Here, we showed that H-exo and N-exo significantly decrease CoCl2-induced apoptosis in hESC-CM.
Here, to isolate the CDC-exosomes from conditioned media, differential ultracentrifugation was used as described in the literatures with some modifications (12, 13). The size of H-exo and N-exo were almost similar, with mean hydrodynamic diameter of 150 to 170 nm. CDC-derived exosomes possessed highly positive expression for exosome surface markers, such as CD63 and CD81 (unpublished data).
CoCl2 is a well-established hypoxia-mimicking substance. CoCl2-treated cells share common features with cells incubated at 1% oxygen (22). CoCl2, as a substrate of the ferrochelatase enzyme, is thought to mimic the hypoxia by binding to the heme molecules (instead of Fe2+). It was shown that the expression level of hypoxia-inducible factor-1α (HIF-1α), which is a major transcription factor and key regulator of adaptive responses to hypoxia, is markedly increased following treatment with CoCl2 in a dose-dependent manner (23). In this study, CoCl2 at concentration of 3 mM was used to induce apoptosis. In the study of Guo et al., U937 and NB4 the cell lines were treated by CoCl2 at different concentrations of 150, 200 and 300 μM. They found that at the concentration of 150, 200, and 300 μM the viability is reduced to 55, 20, and 7 % in U937 and to 60, 50, and 25% in NB4, respectively (15). Kim et al. also demonstrated that neural cells viability is reached to about 60% after 24 h CoCl2 treatment at concentration of 1 mM (16). However, it should be taken into consideration that the method of apoptosis detection is not the same in all studies. For example, trypan-blue exclusion and MTT assay were used to evaluate cell viability by Guo (15) and Kim (16) et al., respectively. Totally, our data are in agreement with the results of Guo et al. (15) which indicate CoCl2 at the concentration of greater than 50 μM induce apoptosis via mitochondria pathway-mediated caspase-3 activation. (15).
In this study, we found that exosomes secreted by human CDCs were cultured 48 h under hypoxic and normoxic conditions (1% O2) inhibited apoptosis at both 25 and 50 μg/mL concentrations. However, the anti-apoptotic effect of N-exo was significantly higher than that of H-exo at concentration of 25 and 50 μg/mL (p <0.0001). In the present study, higher oxygen percentage (1% O2) was used for hypoxic preconditioning compared to hypoxic culture condition (0.5% O2) that was used in the study of Chacko et al. (24). Their results indicated that exposure to sub-lethal hypoxia (0.5% O2) for as long as 72 h by itself does not induce cell death by apoptosis in mesenchymal stem cells (MSCs) (24). Furthermore, exposure of hypoxic preconditioned MSCs (HPC-MSCs) (0.5% O2, 24 h) to severe hypoxia (0.1% O2, 48 h) resulted in significant decrease in apoptosis compared with non-HPC MSCs (24). In another study, to imitate myocardial ischemic–reperfusion, isolated MSC were induced by hypoxia/reoxygenation (H/R) [6 h hypoxia (<0.5% O2) followed by 12 h reoxygenation (21% O2)]. It was found that hypoxia preconditioning (8% O2 hypoxia and 30 min of reoxygenation) inhibits H/R-induced apoptosis of MSC in a time-dependent manner (25). Khatibi et al. reported that preconditioning with H2O2 along with CoCl2 can cause inhibitory effect on cell apoptosis (26).
Recently, a study has reported that the CPC-derived exosomes might be crucial to protect the cardiomyocytes from apoptosis caused by oxidative stress. In their study, exosomes secreted by mice CPCs which were stressed by 100 μM H2O2 for 6h (H2O2-exosomes) decreased the percentage of the apoptotic cells to 13.58%, compared with the 33.29% in H2O2 group, (cardiomyocytes were stressed by 100 μM H2O2 for 6 h), whereas the normal exosomes (non-H2O2 induced) could only reduce the apoptotic percentage to 17.39% (27).
Totally, it can be concluded that different parameters in hypoxia preconditioning of cardiac stem cells including the percentage of oxygen and the duration of hypoxia play critical roles in their anti-apoptotic effect. Therefore, to obtain the optimum anti-apoptotic effect of cardiac stem cells (CSCs)-derived exosomes, further investigation is highly required to choose the proper manner of hypoxic preconditioning.
Additionally, the way of apoptosis induction in cardiomyocyte might be important in the anti-apoptotic effect of CSCs-derived exosomes. The severe hypoxic (0.1% O2) and reduced serum conditions that led to decreasing cells apoptosis in the study of Chacko et al., (24) may be different from the apoptosis condition that was induced by cobalt chloride (3 mM) in our study. In the study of Xiao et al., H2O2 was used to induce the oxidative stress that originates mainly in mitochondria from reactive oxygen species (ROS) (27, 28). Their results demonstrated that H2O2-exosomes reduce H2O2 induced apoptosis. Further investigations dealing with the kind of apoptosis induction will be helpful.
We can get to the conclusion that CDC-secreted exosomes have the potential to prevent apoptosis in cardiomyocytes and they will hopefully provide a promising therapeutic strategy for ischemic cardiac disease. Our results imply the need for further investigation of the effect of hypoxia-preconditioning method of cardiac stem cells on the anti-apoptotic activity of their secreting exosomes.
Acknowledgements
References
-
1.
Schmidt M, Jacobsen JB, Lash TL, Bøtker HE, Sørensen HT. 25 year trends in first time hospitalisation for acute myocardial infarction, subsequent short and long term mortality, and the prognostic impact of sex and comorbidity: a Danish nationwide cohort study. Br. Med. J. 2012;344:e356. [PubMed ID: 22279115].
-
2.
Gallet R, Dawkins J, Valle J, Simsolo E, de Couto G, Middleton R, Tseliou, E Luthringer D, Kreke M, Smith R R, Marbán L, Ghaleh B, Marbán E. Exosomes secreted by cardiosphere-derived cells reduce scarring, attenuate adverse remodelling, and improve function in acute and chronic porcine myocardial infarction. Eur. Heart. J. 2017;38:201-11. [PubMed ID: 28158410].
-
3.
Ibrahim AGE, Cheng K, Marbán E. Exosomes as critical agents of cardiac regeneration triggered by cell therapy. Stem Cell Reports. 2014;2:606-19. [PubMed ID: 24936449].
-
4.
Lener T, Gimona M, Aigner L, Börger V, Buzas E, Camussi G, Chaput N, Chatterjee D, Court FA, Portillo HAD. Applying extracellular vesicles based therapeutics in clinical trials–an ISEV position paper. J. Extracell. Vesicles. 2015;4:30087. [PubMed ID: 26725829].
-
5.
Sahoo S, Losordo DW. Exosomes and cardiac repair after myocardial infarction. Circ. Res. 2014;114:333-44. [PubMed ID: 24436429].
-
6.
Heusch G. Molecular basis of cardioprotection. Circ. Res. 2015;116:674-99. [PubMed ID: 25677517].
-
7.
Greijer A, Van der Wall E. The role of hypoxia inducible factor 1 (HIF-1) in hypoxia induced apoptosis. J. Clin. Pathol. 2004;57:1009-14. [PubMed ID: 15452150].
-
8.
Beninson LA, Fleshner M, editors. Exosomes: an emerging factor in stress-induced immunomodulation. Seminars in immunology. Elsevier; 2014.
-
9.
Gray WD, French KM, Ghosh-Choudhary S, Maxwell JT, Brown ME, Platt MO, Searles CD, Davis ME. Identification of Therapeutic Covariant MicroRNA Clusters in Hypoxia-Treated Cardiac Progenitor Cell Exosomes Using Systems Biology Novelty and Significance. Circ. Res. 2015;116:255-63. [PubMed ID: 25344555].
-
10.
Vahdat S, Mousavi SA, Omrani G, Gholampour M, Sotoodehnejadnematalahi F, Ghazizadeh Z, Gharechahi J, Baharvand H, Hosseini Salekdeh Gh, Aghdami N. Cellular and Molecular Characterization of Human Cardiac Stem Cells Reveals Key Features Essential for Their Function and Safety. Stem Cells Dev. 2015;24:1390-404. [PubMed ID: 25867933].
-
11.
Shelke GV, Lässer C, Gho YS, Lötvall J. Importance of exosome depletion protocols to eliminate functional and RNA-containing extracellular vesicles from fetal bovine serum. J Extracell. Vesicles. 2014;3:24783.
-
12.
Théry C, Amigorena S, Raposo G, Clayton A. Isolation and characterization of exosomes from cell culture supernatants and biological fluids. Curr. Protoc. Cell Biol. 2006. 3.22. 1-3. 9.
-
13.
Sáenz-Cuesta M, Arbelaiz A, Oregi A, Irizar H, I Osorio-Querejeta, M Muñoz-Culla, J M Banales, J M Falcón-Pérez, J M Olascoaga, D Otaegui. Methods for extracellular vesicles isolation in a hospital setting. Front. Immunol. 2015;6:44-55. [PubMed ID: 25798137].
-
14.
Fonoudi H, Ansari H, Abbasalizadeh S, Larijani MR, Kiani S, Hashemizadeh S, Zarchi AS, Bosman A, Gillian Blue M, S Pahlavan. A universal and robust integrated platform for the scalable production of human cardiomyocytes from pluripotent stem cells. Stem Cells Transl. Med. 2015;4:1482-94. [PubMed ID: 26511653].
-
15.
Guo M, Song L-P, Jiang Y, Liu W, Yu Y, Chen G-Q. Hypoxia-mimetic agents desferrioxamine and cobalt chloride induce leukemic cell apoptosis through different hypoxia-inducible factor-1α independent mechanisms. Apoptosis. 2006;11:67-77. [PubMed ID: 16374551].
-
16.
Kim S, Seo J-W, Oh SB, Kim SH, Kim I, Suh N, J Y Lee. Disparate roles of zinc in chemical hypoxia-induced neuronal death. Front. Cell Neurosci. 2015;9:1. [PubMed ID: 25667569].
-
17.
Kreke M, Smith RR, Marbán L, Marbán E. Cardiospheres and cardiosphere-derived cells as therapeutic agents following myocardial infarction. Expert Rev. Cardiovasc Ther. 2012;10:1185-94. [PubMed ID: 23098154].
-
18.
de Couto G, Durvasula P, Ibrahim A, Marbán E. Intracoronary Delivery of Exosomes Secreted by Cardiosphere-Derived Cells Confers Cardioprotection wWth Delayed Administration After Ischemia-Reperfusion Injury in Rats. Circulation. 2014;130(Suppl 2):A15845-A.
-
19.
Csete M. Oxygen in the cultivation of stem cells. Annals of the New York Academy of Sciences. 2005;1049:1-8.
-
20.
Abdollahi H, Harris LJ, Zhang P, McIlhenny S, Srinivas V, Tulenko T, DiMuzio PJ. The role of hypoxia in stem cell differentiation and therapeutics. J. Surg. Res. 2011;165:112-7. [PubMed ID: 20080246].
-
21.
Zhu W, Chen J, Cong X, Hu S, Chen X. Hypoxia and serum deprivation-induced apoptosis in mesenchymal stem cells. Stem Cells. 2006;24:416-25. [PubMed ID: 16253984].
-
22.
Webb JD, Coleman ML, Pugh CW. Hypoxia, hypoxia-inducible factors (HIF), HIF hydroxylases and oxygen sensing. Cell Mol. Life. Sci. 2009;66:3539. [PubMed ID: 19756382].
-
23.
Yang G, Xu S, Peng L, Li H, Zhao Y, Hu Y. The hypoxia-mimetic agent CoCl2 induces chemotherapy resistance in LOVO colorectal cancer cells. Mol. Med. Rep. 2016;13:2583-9. [PubMed ID: 26846577].
-
24.
Chacko SM, Ahmed S, Selvendiran K, Kuppusamy ML, Khan M, Kuppusamy P. Hypoxic preconditioning induces the expression of prosurvival and proangiogenic markers in mesenchymal stem cells. Am. J. Physiol. Cell Physiol. 2010;299:C1562-C70. [PubMed ID: 20861473].
-
25.
Jiang J, Shi H, Gui C, Xiang Mx, Zhang X. Hypoxic preconditioning attenuates hypoxia/reoxygenation-induced apoptosis in mesenchymal stem cells. Acta Pharmacologica Sinica. 2008;29:74-82. [PubMed ID: 18158868].
-
26.
Khatibi S, Taban ZF, Halabian R, Roushandeh AM. Combined preconditioning with hypoxia and Hydrogen Peroxide Improved efficiency of Mesenchymal Stem Cell in cell culture. Entomology and Applied Science Letters. 2017;3:89-96.
-
27.
Xiao J, Pan Y, Li X, Yang X, Feng Y, Tan HH, Jiang L, Feng J, Yu XY. Cardiac progenitor cell-derived exosomes prevent cardiomyocytes apoptosis through exosomal miR-21 by targeting PDCD4. Cell Death Dis. 2016;7:e2277. [PubMed ID: 27336721].
-
28.
Pashkow FJ. Oxidative stress and inflammation in heart disease: do antioxidants have a role in treatment and/or prevention? Int. J. Inflam. 2011;2011:514623.