Abstract
Keywords
Nurses Human Lymphocytes Inhalation Exposure Mitochondrial Damage Antineoplastic Drugs
Introduction
Antineoplastic drugs are widely used agents for the treatment of cancer. most of these agents act by interfering directly with the deoxyribonucleic acid of tumour cells and thereby intercept their growth (1). Unfortunately, antineoplastic drugs are generally non-selective and therefore healthy cells may also be damaged which, in turn, results in toxic effects. Given this, there is a risk to healthcare individuals who handle, prepare, and administer antineoplastic drugs (2). Numerous investigations have examined antineoplastic drug contamination in healthcare instruments. Studies have demonstrated surface contamination of countertops, cabinets and floors within the drug preparation area (3). Traceable levels of environmental drug contamination have also been found in patient care areas. In the absence of any current occupational exposure limits for antineoplastic drugs, it is therefore important to minimize contamination (3). More investigations revealed that these surface contaminations were within a healthcare facility the pharmacy, where the drugs are provided, and the administration units where the prepared drugs are given to patients (4). The emphasis on these places is warranted since direct handling of the drugs is anticipated during both preparation and administration (5, 6).
Employees in oncological units may experience occupational exposure to low doses of anticancer drugs (7). The International Agency for Research on Cancer (IARC) has classified many anticancer drugs as class 1 human carcinogens such as cyclophosphamide, etoposide, busulfan, melfalan, class 2A probably carcinogenic to humans such as azacitidine, cisplatin, doxorubicine and class 2B possibly carcinogenic to humans such as bleomycin, dacarbazine, mitoxantrone, mitomycin (8). Occupational exposure to anticancer drugs has been shown to result in increased total chromosomal aberrations (9-11). Interestingly, nurses occupationally exposed to anticancer drugs showed an elevated level of not only chromatid-type aberrations (CTA), but also chromosome-type aberrations (CSA), such as chromosome breaks, which is typical of radiation exposure (12).
Cancer is one of the leading causes of death in the world. A large percentage of patients are diagnosed at an advanced stage, making the removal of tumors in this population problematic. Therefore, early stage detection would be helpful in reducing cancer mortality (13). For this reason, a well-established assessment model would greatly benefit patients, clinicians, and researchers because it would allow individuals at high risk to be identified at the earliest stages. Accurately assessing cancer risk in average- and high-risk individuals is crucial to controlling the suffering and death due to cancer. Cancer prediction proceedings provide an important approach to assessing risk by identifying individuals at high risk (13).
Human peripheral blood lymphocytes (PBL) are extensively used in biomonitoring of populations exposed to various mutagenic or carcinogenic compounds (14). This is because of the ease of sampling, the possibility of obtaining large numbers of scorable cells (15). The conceptual basis for using of toxicity in PBL as a biomarker has been the hypothesis that the extent of genetic damage in PBL reflects similar events in the precursor cells for carcinogenic processes in the target tissues (16). The aim of this work was to evaluate cellular and mitochondrial toxicity parameters such as cytotoxicity, reactive oxygen species (ROS), mitochondrial membrane potential (MMP), lysosomal integrity, glutathione levels, lipid peroxidation in the blood lymphocytes of oncology nurses occupationally exposed through inhalation exposure to anticancer drugs and compare the findings with those of unexposed hospital nurses.
Experimental
Cellular Assessments
Blood samples
All blood samples (exposed nurses = 50 and unexposed nurses = 50) were acquired and approved by Blood Administration Center. Exposed nurses were exposed with anti-cancer drugs such as 5- Fluoro Uracil, Methotrexate, Cisplatin, Vinblastine and Tamoxifen. The studies were performed at the school of Pharmacy, Shahid Beheshti University of Medical Sciences, under the guidance of an expert physician. This study was approved by Shahid Beheshti University of Medical Sciences, and all healthy individuals signed an informed consent form.
Lymphocytes isolation
Lymphocytes were collected from nurses occupationally exposed to anticancer drugs and unexposed nurses at age range 30 to 35 years old whose anthropometric and biochemical characteristics were similar to those of the expose group. Blood was obtained from, non-smoking volunteers, who showed no signs of infection disease symptoms at the time that the blood samples were collected. Lymphocytes were isolated using Ficoll Paque Plus according to the manufacturerꞌs instructions. The obtained lymphocytes were suspended in RPMI1640 medium with L-glutamine and 10% FBS. The final lymphocytes account used in the experiments was 1 × 106 cells/mL. The viability of the lymphocytes was over 95% (17, 18).
Determination of ROS
To determine the rate of lymphocytes ROS generation, dichlorofluorescein diacetate (DCFH-DA, 1.6 µM) was added to the lymphocytes. It penetrates lymphocytes and becomes hydrolyzed to non-fluorescent dichlorofluorescein (DCFH). The latter then reacts with ROS to form the highly fluorescent dichlorofluorescein (DCF), which effluxes the cell. The fluorescence intensity of DCF was measured using a Shimadzu RF5000U fluorescence spectrophotometer. Excitation and emission wavelengths were 500 nm and 520 nm, respectively. The results were expressed as fluorescent intensity per 106 cells (17, 19).
MMP Assay
Mitochondrial uptake of the cationic fluorescent dye, rhodamine 123 (1.5 µM), has been used for the estimation of mitochondrial membrane potential. The amount of rhodamine 123 remaining in the incubation medium was measured fluorimetrically using a Shimadzu RF5000U fluorescence spectrophotometer set at 490 nm excitation and 520 nm emission wavelengths. The capacity of mitochondria to take up the rhodamine 123 was calculated as the difference (between control and treated cells) in rhodamine 123 fluorescence (17, 20).
Lysosomal Membrane Integrity Assay
Lymphocytes lysosomal membrane stability was determined from the redistribution of the fluorescent dye, acridine orange. Aliquots of the cell suspension (0.5 mL) that were previously stained with acridine orange (5 µM) were separated from the incubation medium by 1 min centrifugation at 1000 rpm. Cell washing process was carried out twice to remove the fluorescent dye from the media. Acridine orange redistribution in the cell suspension was then measured fluorimetrically using a Shimadzu RF5000U fluorescence spectrophotometer set at 495 nm excitation and 530 nm emission wavelengths (17, 21).
Lipid Peroxidation
Evaluation of lipid peroxidation in lymphocytes was conducted by determining the amount of thiobarbituric acid reactive substances (TBARS) formed during the decomposition of lipid hydro peroxides by following the absorbance at 532 nm in a Beckman DU-7 spectrophotometer (17).
GSH and GSSG
GSH and GSSG were determined according to the spectrofluorometric method (22). Each sample was measured in quartz cuvettes using a fluorimeter set at 350 nm excitation and 420 nm emission wavelengths.
ADP/ATP ratio Assay
Changes in the ADP/ATP ratio have been used to differentiate modes of cell death and viability. ADP/ATP ratio was assessed by ADP/ATP Ratio Assay kit (MAK135 sigma, USA) in lymphocytes using luminometer. ADP/ATP ratio was performed according to the manufacturer’s instructions (23).
Mitochondrial Assessments
Lymphocytes Lysis and Isolation of Mitochondria
Mitochondria were isolated from the lymphocytes by mechanical lysis and differential centrifugation. Briefly, lymphocytes were washed with cold PBS at 4 ºC and centrifuged at 450 × g. The pellet was resuspended in cold isolation buffer (75 mmol/L sucrose, 20 mmol/L HEPES, 225 mmol/L mannitol, 0.5 mmol/L EDTA, pH 7.2), and the cells were disrupted by homogenization. Nonlysed lymphocytes and nuclei were spun down by centrifugation at 750 × g for 20 min. The supernatant was further spun at 10,000 × g for 10 min twice. The pellet, designated as the mitochondrial fraction, was suspended in assay buffer (140 mmol/L KCl, 10 mmol/L NaCl, 2 mmol/L MgCl2, 0.5 mmol/L KH2PO4, 20 mmol/L HEPES, 0.5 mmol/L EGTA; adjusted to pH 7.2 with KOH) (24, 25).
Succinate Dehydrogenases Activity
Complex II Enzyme Activity was measured by Complex II Enzyme Activity Microplate Assay Kit (ab109908, United Kingdom). Each well in the kit has been coated with an anti-Complex II monoclonal antibody (mAb) which purifies the enzyme from a complex sample. After this in-well purification the production of ubiquinol by the enzyme is coupled to the reduction of the dye DCPIP (2,6-diclorophenolindophenol) and a decreases in its absorbance at 600 nm, which in turn recycles the substrate ubiquinone (26).
Mitochondrial Swelling Assay
Mitochondria suspensions (at 100 µg protein per well) were incubated in 96-well plates at 25 ºC in swelling buffer (140 mmol/L KCl, 10 mmol/L NaCl, 2 mmol/L MgCl2, 0.5 mmol/L KH2PO4, 20 mmol/L HEPES, 0.5 mmol/L EGTA; adjusted to pH 7.2 with KOH) supplemented with 1 mg/mL rotenone and 10 mmol/L succinate. Mitochondrial swelling was measured spectrophotometrically in duration 1 h. Mitochondrial swelling results in a decrease in absorbance monitored at 540 nm (27).
Cytochrome C Release Assay
The concentration of cytochrome C was determined through using the Quantikine Human Cytochrome C Immunoassay kit (Minneapolis, Minn). Cytochrome C measurement was performed according to the manufacturer’s instructions (28).
Statistical Analysis
Statistical analyses were performed by GraphPad Prism 6. Results were presented as mean ± SED. Assays were performed in triplicate and the mean was used for statistical analysis. All graphs were expressed as mean ± SEM and P < 0.05 was considered statistically significant.
Results
ROS formation
There is equilibrium between ROS formation and endogenous antioxidant defense mechanisms, but if this balance is disturbed, it can produce oxidative stress. As shown in Figure 1A, isolated lymphocytes from oncology nurses in comparison with healthy unexposed individuals showed significant increase at ROS formation.
MMP Collapse
To monitor the mitochondrial membrane potential, lymphocyte mitochondria were stained with rhodamine 123. Isolated lymphocytes from oncology nurses in comparison with healthy unexposed individuals showed a significant collapse at the mitochondrial membrane potential. These changes were in the accordance with the order of ROS formation of the isolated lymphocytes from oncology nurses (Figure 1B).
Lysosomal Integrity
As shown in Figure 2A there was a loss of lysosomal membrane integrity measured by redistribution of fluorescent dye acrdine orange in the lymphocytes isolated from nurses occupationally exposed to anticancer drugs. While no significant lysosomal damage was detected in lymphocytes isolated from healthy unexposed nurses.
ADP/ATP ratio
ATP and ADP were measured using a bioluminescence assay based on luciferin/luciferase reaction. The ADP and ATP were measured at both groups (Figure 2B). As shown in Figure 2B there was a significant decrease in ATP and a significant increase in ADP levels only in lymphocytes isolated from oncology nurses (P < 0.05) while no significant changes at ADP/ATP ratio observed in healthy unexposed staff lymphocytes.
Lipid Peroxidation and Glutathione Levels
Lipid per-oxidation in lymphocytes was determined by measuring MDA formation. As shown in Figure 3, significant MDA formation only observed in the lymphocytes isolated from nurses occupationally exposed to anticancer drugs. While no significant MDA formation was detected in lymphocytes isolated from healthy unexposed nurses.
In addition, as shown in Figure 3 reduced (GSH) and oxidized (GSSG) glutathione levels were determined under the same experimental conditions and correlated with MDA formation and other measured parameters. A significant decrease in reduced glutathione (GSH) level was observed in the lymphocytes isolated from nurses occupationally exposed to anticancer drugs followed by a significant increase of oxidized (GSSG) glutathione levels compared to those of healthy unexposed staff under the similar condition.
Mitochondrial Assessment
SDH Activity
Evaluations of SDH Activity in isolated mitochondria obtained from both oncology and healthy nurses were carried out by studying succinate dehydrogenase activity using the Complex II Enzyme Activity Microplate Assay Kit. Succinate dehydrogenase activity significantly reduced only in mitochondria from oncology but not in healthy unexposed nurses’ lymphocytes (Figure 4A).
Mitochondrial Swelling
Mitochondrial swelling has been considered as an indication of the opening of the MPTP, which results in depolarization of mitochondrial membrane potential. We observed that mitochondrial swelling occurred only in mitochondria obtained from oncology but not healthy unexposed nurses’ lymphocytes. We also recorded mitochondrial membrane potential decrease using rhodamine 123 and ROS formation using DCF-DA in the isolated mitochondria obtained from blood lymphocytes of both groups (data not shown) (Figure 4B).
Cytochrome C Release
The process of cell death involves the release of cytochrome C from the mitochondria, which subsequently causes apoptosis. Our results showed significant mitochondrial swelling and collapse of the mitochondrial membrane potential only in mitochondria isolated from oncology nurses’ lymphocytes (data not shown). These events could result in mitochondrial permeability transition and release of cytochrome C from mitochondria into the cytosolic fraction. As shown in Figure 4C, significant release of cytochrome C was shown in mitochondria isolated from nurses’ lymphocytes compared to unexposed blood lymphocyte mitochondria.
Discussion
We hypothesized that exposure to antineoplastic drugs leads to increased toxicity in blood lymphocytes in the hospital nurses or staff occupationally exposed to anticancer drugs. Through observation and biochemical experiments, our study results proved that toxicity parameters increased in blood lymphocytes of exposed group compared to those of unexposed nurses. Through different studies show that surface contamination, and accordingly the potential for occupational exposure to antineoplastic drugs, occurs at every stage of the hospital medication system (2). Those job categories most likely in risk of exposure are nurse, pharmacy technician, pharmacy receiver, and pharmacist. Over 11 job categories (not including housekeeping) per site are potentially at risk of exposure. Several studies have shown that exposure to antineoplastic drugs can cause toxic effects (2). Dermal exposure has been suggested to be the main route of exposure (29). In a recently study, was assessed dermal exposure to cyclophosphamide. Surprisingly, exposure levels detected on the hands during nursing tasks were higher than those measured in the hospital pharmacy during preparation of cyclophosphamide. The positive urine samples with detectable cyclophosphamide and other anti-neoplastic drugs were observed in hospital personals (29). Also studies show that the prevalence of needle stick injury (NSI) in Iranian nurses is high that likelihood exposure to anti-neoplastic drugs has increased (30).
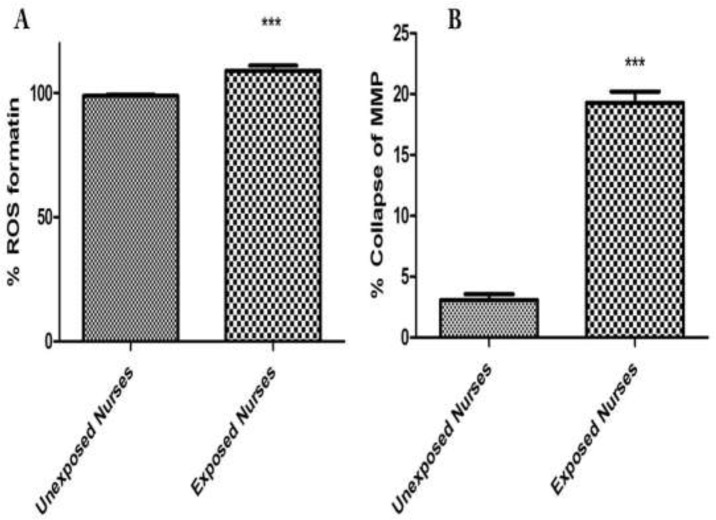
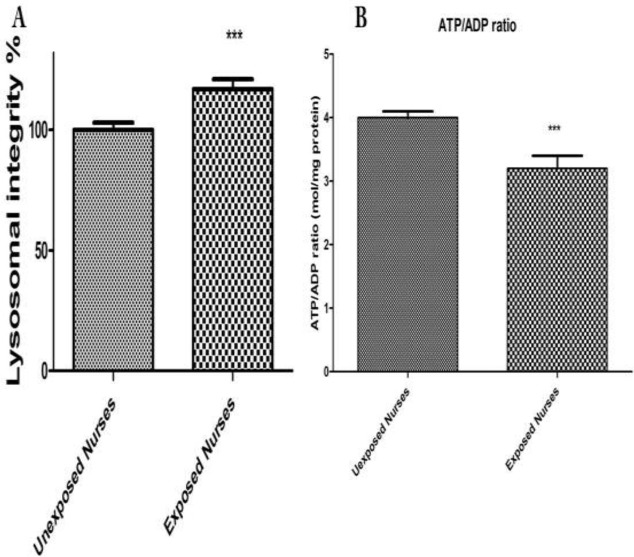
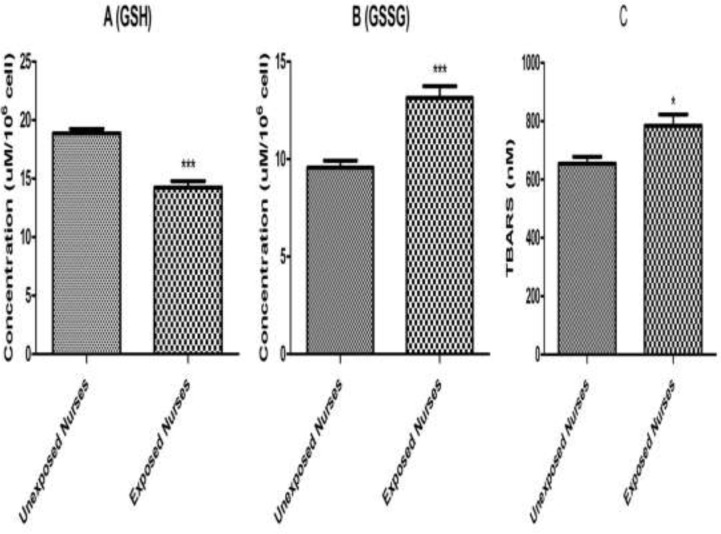
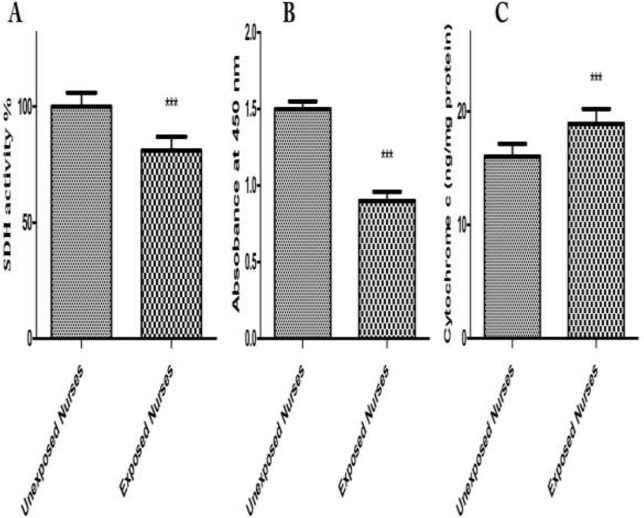
ROS formation was compared between occupationally exposed nurseꞌs lymphocytes and unexposed. Referring to the results of ROS formation status in the present study, it was shown that cellular and mitochondrial ROS formation was significantly increased in the lymphocytes of occupationally exposed group, compared to those of unexposed control (P < 0.05) (Figure 1A). Neoplastic disease studies revealed that treatment with anti-neoplastic drugs increases ROS and reduces plasma levels of vitamins C and E as well as of glutathione peroxidase (31). Consumption of variety of anti-cancer drugs have been associated with oxidative stress. For example, cisplatin induces formation of reactive oxygen species (ROS) in renal proximal tubular cell mitochondria, eliciting oxidative alterations in lipids, proteins, and DNA of this organelle (32), while doxorubicin induced cytotoxicity has been associated with ROS production and in particular to presence of the superoxide anion radical and of hydrogen peroxide in cardiomyocytes (33). This drug is also able to produce reactive nitrogen species. The oxidant RNS such as peroxynitrite is known to induce protein oxidation and nitration in the absence of GSH, eliciting mitochondrial dysfunction and eventually leading to irreversible damage and severe loss of cellular ATP (34). A recent study has also indicated that exposed nurses to antineoplastic drug suffer from oxidative stress compared to unexposed nurses (35). It is worth noting that both drugs are prepared, handled, and administered by nursing personnel in hospitals. Our results proved that content of glutathione and ATP has decreased in occupationally exposed nurses lymphocytes in comparison to unexposed nurses (Figure 3 and Figure 2B). The decreases in lipid peroxidation level found in our study may be explained by an increase in ROS formation produced by anti-neoplastic drugs in occupationally exposed nurseꞌs lymphocytes, such as superoxide anion and hydrogen peroxide, which are known to attach to membrane lipids, initiating lipid peroxidation. Similarly, increased ROS formation may directly oxidize the prosthetic protein group or else reacts directly with the peptide chain, leading to conformational and functional changes in cellular organelles such as mitochondria and lysosomes (36-38). The results of this study also showed occurrence of mitochondrial and lysosomal damages in occupationally exposed nurseꞌs lymphocytes, compared to the control group (P < 0.05) (Figure 1B and Figure 2A).
Previous studies indicated an increased risk of a prolonged time to pregnancy among nurses with relatively high exposure to antineoplastic agents compared with referent nurses (39). In addition to the toxicity to reproduction system, antineoplastic drugs have been found to have carcinogenic potential. Nine antineoplastic drugs have been classified by IARC to be carcinogenic to humans. The leukemia risk of an oncology nurse after 40 years of dermal exposure to cyclophosphamide was estimated to be on average of 0.27 (range = 0 - 40) extra cases per million oncology nurses (40).
Mitochondria perform significant roles in cellular energy metabolism, free radicals generation, control of cell death, growth, development, integration of signals from mitochondria to nucleus and nucleus to mitochondria, and various metabolic pathways (41, 42). Introduction of mtDNA mutations in transformed cells has been associated with increased ROS production and tumor growth. Studies revealed that increased and altered mtDNA plays a role in the development of cancer (43). Mitochondrial alterations have been identified in several cancers (44). An increase in mtDNA copy number was observed in patients with a higher risk for breast cancer (45). Our results on isolated mitochondria obtained from occupationally exposed nurseꞌs lymphocytes showed significant mitochondrial toxic changes such as mitochondrial swelling, inhibition of SDH and cytochrome C release (Figure 4). Mitochondrial alterations have been associated with some forms of cancer and an increased risk of certain age-related disorders such as heart disease, Alzheimer disease, Parkinson disease and immunotoxicity (46). Additionally, our results suggest that the gradual and progressive occurrence of these alterations in exposed oncology drugs may play a role in progression of mentioned diseases.
Our results showed that occupationally exposed nurseꞌs lymphocytes were more susceptible to oxidative stress than unexposed nurses. Finally, isolated mitochondria from lymphocytes could be a biomarker for determination people at risk of oxidative diseases such as cancer and aging.
Acknowledgements
References
-
1.
Sessink PJ, Bos RP. Drugs hazardous to healthcare workers. Drug Safety. 1999;20:347-59. [PubMed ID: 10230582].
-
2.
Hon C-Y, Teschke K, Chua P, Venners S, Nakashima L. Occupational exposure to antineoplastic drugs: identification of job categories potentially exposed throughout the hospital medication system. Safety and health at work. 2011;2:273-81. [PubMed ID: 22953211].
-
3.
Siderov J, Kirsa S, McLauchlan R. Surface contamination of cytotoxic chemotherapy preparation areas in Australian hospital pharmacy departments. Journal of Pharmacy Practice and Research. 2009;39:117-21.
-
4.
Schmaus G, Schierl R, Funck S. Monitoring surface contamination by antineoplastic drugs using gas chromatography-mass spectrometry and voltammetry. American journal of health-system pharmacy. AJHP: official journal of the American Society of Health-System Pharmacists. 2002;59:956-61. [PubMed ID: 12040735].
-
5.
Cavallo D, Ursini CL, Perniconi B, Di Francesco A, Giglio M, Rubino FM, Marinaccio A, Iavicoli S. Evaluation of genotoxic effects induced by exposure to antineoplastic drugs in lymphocytes and exfoliated buccal cells of oncology nurses and pharmacy employees. Mutation Research/Genetic Toxicology and Environmental Mutagenesis. 2005;587:45-51.
-
6.
Touzin K, Bussieres J-F, Langlois È, Lefebvre M. Evaluation of surface contamination in a hospital hematology–oncology pharmacy. Journal of Oncology Pharmacy Practice. 2008.
-
7.
Gabano E, Ravera M, Osella D. The drug targeting and delivery approach applied to Pt-antitumour complexes A coordination point of view. Current medicinal chemistry. 2009;16:4544-80. [PubMed ID: 19903151].
-
8.
Bouvard V, Baan R, Straif K, Grosse Y, Secretan B, El Ghissassi F, Benbrahim-Tallaa L, Guha N, Freeman C, Galichet L. A review of human carcinogens—Part B: biological agents. The lancet oncology. 2009;10:321-2. [PubMed ID: 19350698].
-
9.
Musak L, Smerhovsky Z, Halasova E, Osina O, Letkova L, Vodickova L, Polakova V, Buchancova J, Hemminki K, Vodicka P. Chromosomal damage among medical staff occupationally exposed to volatile anesthetics, antineoplastic drugs, and formaldehyde. Scandinavian journal of work, environment & health. 2013:618-30.
-
10.
Boughattas AB, Bouraoui S, Debbabi F, El Ghazel H, Saad A, Mrizak N. Évaluation du risque génotoxique chez les infirmiers manipulant les cytostatiques. in Annales de Biologie Clinique. 2010.
-
11.
Kopjar N, Garaj-Vrhovac V, Kašuba V, Rozgaj R, Ramić S, Pavlica V, Želježić D. Assessment of genotoxic risks in Croatian health care workers occupationally exposed to cytotoxic drugs: a multi-biomarker approach. International journal of hygiene and environmental health. 2009;212:414-31. [PubMed ID: 19049854].
-
12.
Testa A, Giachelia M, Palma S, Appolloni M, Padua L, Tranfo G, Spagnoli M, Tirindelli D, Cozzi R. Occupational exposure to antineoplastic agents induces a high level of chromosome damage Lack of an effect of GST polymorphisms. Toxicology and applied pharmacology. 2007;223:46-55. [PubMed ID: 17631926].
-
13.
Wang X, Oldani MJ, Zhao X, Huang X, Qian D. A review of cancer risk prediction models with genetic variants. Cancer informatics. 2014;13:19.
-
14.
Pourahmad J, Salimi A. Isolated Human Peripheral Blood Mononuclear Cell (PBMC), a Cost Effective Tool for Predicting Immunosuppressive Effects of Drugs and Xenobiotics. Iranian journal of pharmaceutical research: IJPR. 2015;14:979. [PubMed ID: 26664364].
-
15.
Hagmar L, Brøgger A, Hansteen I-L, Heim S, Högstedt B, Knudsen L, Lambert B, Linnainmaa K, Mitelman F, Nordenson I. Cancer risk in humans predicted by increased levels of chromosomal aberrations in lymphocytes: Nordic study group on the health risk of chromosome damage. Cancer research. 1994;54:2919-22. [PubMed ID: 8187078].
-
16.
Speit G, Ladeira C, Linsenmeyer R, Schütz P, Högel J. Re-evaluation of a reported increased micronucleus frequency in lymphocytes of workers occupationally exposed to formaldehyde. Mutation Research/Genetic Toxicology and Environmental Mutagenesis. 2012;744:161-6.
-
17.
Salimi A, Vaghar-Moussavi M, Seydi E, Pourahmad J. Toxicity of methyl tertiary-butyl ether on human blood lymphocytes. Environmental Science and Pollution Research. 2016;23:8556-64. [PubMed ID: 26797945].
-
18.
Kalantari H, Jalali M, Jalali A, Salimi A, Alhalvachi F, Varga B, Juhasz B, Jakab A, Kemeny-Beke A, Gesztelyi R. Protective effect of Cassia fistula fruit extract on bromobenzene-induced nephrotoxicity in mice. Human & experimental toxicology. 2011;30:1710-5. [PubMed ID: 21247991].
-
19.
Salimi A, Eybagi S, Seydi E, Naserzadeh P, Kazerouni NP, Pourahmad J. Toxicity of macrolide antibiotics on isolated heart mitochondria: a justification for their cardiotoxic adverse effect. Xenobiotica. 2016;46:82-93. [PubMed ID: 26068526].
-
20.
Pourahmad J, Eskandari MR, Shakibaei R, Kamalinejad M. A search for hepatoprotective activity of aqueous extract of Rhus coriaria L against oxidative stress cytotoxicity. Food and Chemical Toxicology. 2010;48:854-8. [PubMed ID: 20036300].
-
21.
Seydi E, R Rasekh H, Salimi A, Mohsenifar Z, Pourahmad J. Selective Toxicity of Apigenin on Cancerous Hepatocytes by Directly Targeting their Mitochondria. Anti-Cancer Agents in Medicinal Chemistry (Formerly Current Medicinal Chemistry-Anti-Cancer Agents). 2016;16:1576-86.
-
22.
Hissin PJ, Hilf R. A fluorometric method for determination of oxidized and reduced glutathione in tissues. Analytical biochemistry. 1976;74:214-26. [PubMed ID: 962076].
-
23.
Salimi A, Ayatollahi A, Seydi E, Khomeisi N, Pourahmad J. Direct toxicity of amyloid beta peptide on rat brain mitochondria: preventive role of Mangifera indica and Juglans regia. Toxicological & Environmental Chemistry. 2015;97:1057-70.
-
24.
Rotem R, Heyfets A, Fingrut O, Blickstein D, Shaklai M, Flescher E. Jasmonates: novel anticancer agents acting directly and selectively on human cancer cell mitochondria. Cancer research. 2005;65:1984-93. [PubMed ID: 15753398].
-
25.
Salimi A, Roudkenar MH, Sadeghi L, Mohseni A, Seydi E, Pirahmadi N, Pourahmad J. Ellagic acid, a polyphenolic compound, selectively induces ROS-mediated apoptosis in cancerous B-lymphocytes of CLL patients by directly targeting mitochondria. Redox biology. 2015;6:461-71. [PubMed ID: 26418626].
-
26.
Salimi A, Saharkhiz MP, Motallebi A, Seydi E, Mohseni AR, Nazemi M, Pourahmad J. Standardized Extract of the Persian Gulf Sponge, Axinella Sinoxea Selectively Induces Apoptosis through Mitochondria in Human Chronic Lymphocytic Leukemia Cells. Journal of Analytical Oncology. 2015;4:132-40.
-
27.
Talari M, Seydi E, Salimi A, Mohsenifar Z, Kamalinejad M, Pourahmad J. Dracocephalum: novel anticancer plant acting on liver cancer cell mitochondria. BioMed research international. 2014;2014.
-
28.
Salimi A, Motallebi A, Ayatollahi M, Seydi E, Mohseni AR, Nazemi M, Pourahmad J. Selective toxicity of persian gulf sea cucumber holothuria parva on human chronic lymphocytic leukemia b lymphocytes by direct mitochondrial targeting. Environmental Toxicology. 2016.
-
29.
Fransman W, Vermeulen R, Kromhout H. Dermal exposure to cyclophosphamide in hospitals during preparation, nursing and cleaning activities. International archives of occupational and environmental health. 2005;78:403-12. [PubMed ID: 15887018].
-
30.
Askarian M, Shaghaghian S, McLaws M-L. Needlestick injuries among nurses of Fars province, Iran. Annals of epidemiology. 2007;17:988-92. [PubMed ID: 17923420].
-
31.
Andreadou I, Sigala F, Iliodromitis EK, Papaefthimiou M, Sigalas C, Aligiannis N, Savvari P, Gorgoulis V, Papalabros E, Kremastinos DT. Acute doxorubicin cardiotoxicity is successfully treated with the phytochemical oleuropein through suppression of oxidative and nitrosative stress. Journal of molecular and cellular cardiology. 2007;42:549-58. [PubMed ID: 17223128].
-
32.
Santandreu FM, Roca P, Oliver J. Uncoupling protein-2 knockdown mediates the cytotoxic effects of cisplatin. Free Radical Biology and Medicine. 2010;49:658-66. [PubMed ID: 20595066].
-
33.
Deng S, Kruger A, Kleschyov AL, Kalinowski L, Daiber A, Wojnowski L. Gp91phox-containing NAD (P) H oxidase increases superoxide formation by doxorubicin and NADPH. Free Radical Biology and Medicine. 2007;42:466-73. [PubMed ID: 17275678].
-
34.
Wagner BA, Evig CB, Reszka KJ, Buettner GR, Burns CP. Doxorubicin increases intracellular hydrogen peroxide in PC3 prostate cancer cells. Archives of biochemistry and biophysics. 2005;440:181-90. [PubMed ID: 16054588].
-
35.
Gómez-Oliván LM, Miranda-Mendoza GD, Cabrera-Galeana PA, Galar-Martínez M, Islas-Flores H, SanJuan-Reyes N, Neri-Cruz N, García-Medina S. Oxidative stress induced in nurses by exposure to preparation and handling of antineoplastic drugs in Mexican hospitals: a multicentric study. Oxidative medicine and cellular longevity. 2014.
-
36.
Denicola A, Radi R. Peroxynitrite and drug-dependent toxicity. Toxicology. 2005;208:273-88. [PubMed ID: 15691591].
-
37.
Kang KA, Hyun JW. Oxidative stress, Nrf2, and epigenetic modification contribute to anticancer drug resistance. Toxicological research. 2017;33:1. [PubMed ID: 28133507].
-
38.
Tran Q, Lee H, Park J, Kim S-H, Park J. Targeting Cancer Metabolism-Revisiting the Warburg Effects. Toxicological Research. 2016;32:177. [PubMed ID: 27437085].
-
39.
Fransman W, Roeleveld N, Peelen S, de Kort W, Kromhout H, Heederik D. Nurses with dermal exposure to antineoplastic drugs: reproductive outcomes. Epidemiology. 2007;18:112-9. [PubMed ID: 17099323].
-
40.
Meijster T, Fransman W, van Hemmen J, Kromhout H, Heederik D, Tielemans E. A probabilistic assessment of the impact of interventions on oncology nurses’ exposure to antineoplastic agents. Occupational and environmental medicine. 2006;63:530-7. [PubMed ID: 16551759].
-
41.
Singh AK, Pandey P, Tewari M, Pandey HP, Shukla HS. Human mitochondrial genome flaws and risk of cancer. Mitochondrial DNA. 2014;25:329-34. [PubMed ID: 25204536].
-
42.
Kim A. Mitochondria in Cancer Energy Metabolism: Culprits or Bystanders? Toxicological research. 2015;31:323.
-
43.
Chatterjee A, Mambo E, Sidransky D. Mitochondrial DNA mutations in human cancer. Oncogene. 2006;25:4663-74. [PubMed ID: 16892080].
-
44.
Pourahmad J. Natural compounds target mitochondrial alterations in cancer cell: new avenue for anticancer research. Iranian journal of pharmaceutical research: IJPR. 2014;13:1.
-
45.
Shen J, Platek M, Mahasneh A, Ambrosone CB, Zhao H. Mitochondrial copy number and risk of breast cancer: a pilot study. Mitochondrion. 2010;10:62-8. [PubMed ID: 19788937].
-
46.
Zapico SC, Ubelaker DH. mtDNA mutations and their role in aging, diseases and forensic sciences. Aging and disease. 2014;4:364-80.