Abstract
Keywords
N-(substitutedphenyl)-2-chloroacetamides Lipophilicity RPTLC Pharmacokinetic predictors Cluster Analysis Principal Component Analysis
Introduction
The task of contemporary research is the synthesis of compounds that may exhibit some biological activity. It has been found that most of the bioactive compounds synthesized during the last decade belong to the class of amides (1-5). Among numerous structurally different amide derivatives, the acetamides evince the most diverse pharmacological potential. Their characteristics make them applicable in numerous fields of herbal, veterinary, and human medicine. They have proved as fungicides (6), insecticides (7), bactericides (8), analgesics (9), anticonvulsants (10), antioxidants (11), antituberculotics (12), antidepressants (13), and antitumor agents (14-16). It has also been confirmed that some of acetamides are responsible for the regulation of body temperature (17) and apetite (18). The indisputable fact is that the type and intensity of activity of the acetamides is conditioned by the nature of the substituent attached to the basic molecule, as well as by the kind of interactions that the examined compound achieved at the site of action with the environment (19). Discovering, examining, and developing bioactive candidates (drug) with adequate properties carries a lot of risk, investment, and time (20). Bearing this in mind, the rationalization of future agent design could be accomplished by establishing dependencies between its structure, physicochemical properties and activity. The recognition and selection of relevant molecular descriptors which could be linked to the biological activity make this easier. For that purpose the often applied drug-likeness index are the Lipinski’s rule of five (Ro5) and the Ghose’s rule (GR) (21-25). Among numerous molecular descriptors, lipophilicity acts as the key one for the estimation of the biological activity of the compound. It determines the passage of compounds through the biomembranes and it is usually defined by the partition coefficient, log P (logarithm of the ratio of the concentrations of solute in the system 1-octanol-water) (26, 27). Apart from the standard criteria of lipophilicity, log P, chromatographic retention parameters, RM0 and m, obtained by RPTLC as alternative measures of lipophilicity have been applied (28-32). Also, lipophilicity is closely related to the pharmacokinetic properties (absorption, distribution, metabolism and elimination-ADME) of compound and thereby defines its bioavailability and effectiveness in the organism (33, 34).
Taking into account the diversity of the biological activity of acetamides, the objects of this study are derivatives of N-(substituted phenyl)-2-chloroacetamides. Firstly, the studied compounds were checked whether they fulfill the conditions of drug-likeness within the rules of Lipinski and Ghose. Also, the influence of the molecule’s structure and the applied organic modifier on the lipopohilicity determined by RPTLC was examined. In order to investigate the possibility of application of the chromatographic parameters of the tested acetamides as measures of their lipophilicity and bioavailability, RM0 and m were correlated with partition coefficient, log P, as well as with important pharmacokinetic parameters (Peff, PPB and log BBB) by linear regression analysis. A more detailed insight into the (dis)similarities between parameters of the Lipinski/Ghose, chromatographically/mathematically determined lipophilicity and pharmacokinetic properties of N-(substituted phenyl)-2-chloroacetamides was acquired by applying two methods of multivariate analysis: cluster analysis, CA, and principal component analysis, PCA.
Experimental
Materials and Methods
The structures of the examined derivatives are presented in Table 1. During chromatographic examinations commercial plates (RP-18W/UV254 Macherey-Nagel GmBH and Co., Duren, Germany) were used as the stationary phase. Mixtures of LC grade organic modifiers (J. T. Baker, Deventer, the Netherlands) and filtered bi-distilled water were used as the mobile phase.
RPTLC conditions
The tested chloroacetamides were dissolved in ethanol (analytical purity) in concentration 2 mg mL–1. About 0.2 µL of freshly prepared solution of each compound was spotted on the plates which were then developed by the ascending technique in the unsaturated chambers at room temperature with an aqueous solutions of two organic modifiers: methanol (φ = 0.34 - 0.56, v/v) and acetone (φ = 0.34 - 0.56, v/v). The visualization of the tested compounds on the dried developed plates was performed under a UV light at λ= 254 nm. In both applied modifiers for each composition three chromatograms were developed and then the average Rf values were calculated.
Processing of results
The obtained experimental data were processed by Origin 6.1 software. The values of the partition coefficient, log P, were calculated using virtual Computational Chemistry Laboratory, VCCLAB (35). Molecular descriptors and values of pharmacokinetic predictors were obtained using Molinspiration, ChemSilico and SimulationPlus online programs (36-38). The CA and PCA procedures were performed by Statistica v.12 software (StatSoft Inc., Tulsa, OK, USA).
Results and Discussion
Accordance of N-(substituted phenyl)-2-chloroacetamides with the drug-likeness rules
Relying on the already confirmed biological activity of many chloroacetamide derivatives, the possibility that the studied N-(substituted phenyl)-2-chloroacetamides have bioactive potential was examined unempirically by two drug-likeness index: the Lipinski’s rule of five and the Ghose’s rule. According to the Lipinski’s rule of five, a potentially biologically active substance should have: molecular weight ≤ 500; number of hydrogen bond donor ≤ 5; number of hydrogen bond acceptor ≤ 10 (2×5) and values of calculated log P ≤ 5. Similarly, in compliance with the Ghose’s rule a bioactive agent should possess: molecular weight within 160-480; values of calculated log P between -0.4 and 5.6; the total number of atoms in molecule within 20-70 and molar refractivity in the range 40-130 (21, 22).
In Tables 2 and 3 the appropriate molecular descriptors of the investigated chloroacetamides are listed.
It is noticeable from Table 3 that for the same compound different values of partition coefficient, log P, as standard measure of lipophilicity, were obtained. This is the result of applying different mathematical methods for calculating log P. Independently of the mathematical calculating process the highest value of partition coefficient was obtained for compound with -I as substituent, and the lowest value was registered for compound with -OH group. Also, almost no difference was found for compound with the same substituent in different positions in the values of partition coefficients (-Br and -CN).
In total, the data shown in Tables 2 and 3 indicate that the examined derivatives have theoretical predisposition for some activity in biological medium because they fulfill the Lipinski’s rule of five and also mainly fit into the Ghose’s rule. Due to the fact that lipophilicity represents the most common indicator of the biological activity of the compound, it was further examined in more detail for derivatives of chloroacetamides.
Determination of lipophilicity of chloroacetamide derivatives by RPTLC
The interactions which the compound makes with its surroundings are very similar to those that it can establish with mobile and stationary phase during chromatographic analysis. With this in mind, evaluating lipophilicity of the tested chloroacetamides was carried out by RPTLC in water mixtures of one protic solvent (methanol) and one aprotic solvent (acetone) separately, with changing amount of the used solvents.
From the experimentally obtained Rf values, for each composition of the mobile phase, RM values were calculated according to the Equation:
The relationships between the retention values, RM, of the compound and the volume fraction of the organic modifier in the mobile phase, φ, were given by:
Where intercept, RM0, represents the chromatographic retention constant, while the slope of the linear plot, m, which is the change in RM is caused by the unit of the organic modifier concentration in the mobile phase and depends on the properties of the solute. Commonly, it is related to the specific hydrophobic surface area of the compound (39). Chromatographic parameter m can be applied as alternative measure of lipophilicity, also (40, 41).Values of these parameters obtained for chloroacetamides in the used modifiers are given in Table 4.
High values of the regression coefficients, r, confirmed the validity of the linear dependencies in the chosen field of experimental work, were established between the retention parameter, RM, and the volume fraction of the organic modifier, φ.
It can be noticed from Table 4 that the nature of the substituent affects more the values of chromatographic parameters RM0 and m of analyzed chloroacetamides than the used organic modifier. Moreover, m values follow values of RМ0 and therefore the existence of the relations between these chromatographic parameters was assumed (Table 5).
The presumption that the chromatographic parameters, RM0 and m, depend on the same physico-chemical characteristics is confirmed by high values of the regression coefficient of their linear correlation. This phenomenon was noted in literature, earlier (42). Additionally a separation of the compounds based on the polarity of substituents (Figure 1) was observed in methanol as modifier. This did not happen in acetone because it is less polar than the methanol, and thus less sensitive to detect the differences in the polarity of the substituents (Figure 2).
Relationship between the standard measure of lipophilicity, log P, and chromatographic parameters, RM0 and m
With the idea to examine whether the chromatographic parameters, RM0, and m could be applied as valid measures of lipophilicity of the studied chloroacetamide derivatives, these two parameters were correlated firstly with the software calculated partition coefficient, log P, as a standard criteria of lipophilicity applying linear regression analysis. Derivatives with substituents at the position 3 were not included in correlations, because the mathematical procedure could not register the influence of substituent’s different position on the lipophilicity of compound.
Figures 3 and 4 show obtained dependences AClog P - RM0 and AClog P - m in methanol as a modifier, respectively.
From Figures 3 and 4 the existence of linear dependence can be seen between experimentally (RM0 and m) and mathematically (log P) determined lipophilicity of examined chloroacetamides. As previously noted, a grouping was again registered in methanol, of compounds based on the polarity of their substituent, which was not the case in acetone.
In Table 6, the correlation matrix is given which was obtained as a result of the linear regression analysis between the chromatographic parameters of the examined compounds, RM0 and m, determined in both modifiers used and various log P-values.
Structures of the examined N-(substituted phenyl)-2-chloroacetamides
Computed molecular descriptors of studied N-(substituted phenyl)-2-chloroacetamides
R | MW | nON | nOHNH | natoms | MR |
---|---|---|---|---|---|
H | 169.611 | 2 | 1 | 19 | 43.76 |
4CH3 | 183.638 | 2 | 1 | 22 | 49.66 |
4OCH3 | 199.637 | 3 | 1 | 23 | 51.01 |
4Cl | 204.056 | 2 | 1 | 19 | 48.37 |
4Br | 248.507 | 2 | 1 | 19 | 51.46 |
4F | 187.601 | 2 | 1 | 19 | 44.17 |
4I | 295.507 | 2 | 1 | 19 | 56.26 |
4COCH3 | 211.648 | 3 | 1 | 24 | 55.01 |
4OH | 185.61 | 3 | 2 | 20 | 45.58 |
3CN | 194.621 | 3 | 1 | 20 | 49.86 |
4CN | 194.621 | 3 | 1 | 20 | 49.86 |
3Br | 248.507 | 2 | 1 | 19 | 51.46 |
Software calculated log P-values of tested chloroacetamides
R | AClog P | Alog P | Alog Ps | Mlog P | milog P | kowwin | log Pch.s | Xlog P3 |
---|---|---|---|---|---|---|---|---|
3Br | 2.47 | 2.44 | 2.42 | 2.66 | 2.51 | 2.57 | 2.78 | 2.94 |
H | 1.77 | 1.70 | 1.73 | 1.95 | 1.72 | 1.68 | 1.64 | 1.63 |
4CH3 | 2.08 | 2.18 | 1.87 | 2.25 | 2.17 | 2.23 | 2.31 | 1.99 |
4OCH3 | 1.66 | 1.68 | 1.69 | 1.68 | 1.78 | 1.76 | 1.86 | 1.67 |
4Cl | 2.38 | 2.36 | 2.39 | 2.52 | 2.40 | 2.32 | 2.59 | 2.26 |
4Br | 2.47 | 2.44 | 2.42 | 2.66 | 2.53 | 2.57 | 2.79 | 2.32 |
4F | 1.83 | 1.90 | 2.00 | 2.37 | 1.88 | 1.88 | 1.91 | 1.73 |
4I | 2.70 | 2.72 | 2.94 | 2.81 | 2.81 | 2.85 | 3.21 | 2.28 |
4COCH3 | 1.69 | 1.43 | 1.59 | 1.89 | 1.62 | 1.36 | 1.65 | 1.86 |
4OH | 1.47 | 1.43 | 0.97 | 1.38 | 1.24 | 0.85 | 1.32 | 1.27 |
3CN | 1.58 | 1.57 | 1.53 | 1.59 | 1.45 | 1.78 | 1.50 | 1.82 |
4CN | 1.58 | 1.57 | 1.54 | 1.59 | 1.48 | 1.78 | 1.52 | 1.35 |
Parameters of the chromatographic equations RМ0, m, r and sd obtained for chloroacetamides in used modifiers.
R | methanol | acetone | ||||||
---|---|---|---|---|---|---|---|---|
RM0 | m | r | sd | RM0 | m | r | sd | |
H | 1.171 (±0.123) | -2.309 (±0.268) | 0.980 | 0.046 | 1.106 (±0.132) | -2.225 (±0.285) | 0.984 | 0.026 |
4CH3 | 1.542 (±0.067) | -2.673 (±0.146) | 0.996 | 0.025 | 1.370 (±0.097) | -2.583 (±0.220) | 0.989 | 0.028 |
4OCH3 | 1.247 (±0.122) | -2.478 (±0.265) | 0.983 | 0.045 | 1.130 (±0.115) | -2.431 (±0.258) | 0.983 | 0.033 |
4Cl | 1.788 (±0.140) | -2.944 (±0.306) | 0.984 | 0.052 | 1.636 (±0.139) | -2.945 (±0.313) | 0.984 | 0.040 |
4Br | 1.951 (±0.100) | -3.137 (±0.218) | 0.993 | 0.037 | 1.849 (±0.087) | -3.175 (±0.195) | 0.994 | 0.025 |
4F | 1.354 (±0.088) | -2.486 (±0.192) | 0.991 | 0.033 | 1.341 (±0.042) | -2.440 (±0.095) | 0.998 | 0.012 |
4I | 2.124 (±0.122) | -3.282 (±0.266) | 0.990 | 0.046 | 1.963 (±0.143) | -3.375 (±0.323) | 0.986 | 0.041 |
4COCH3 | 1.275 (±0.121) | -2.549 (±0.263) | 0.984 | 0.045 | 1.118 (±0.077) | -2.396 (±0.173) | 0.992 | 0.022 |
4OH | 1.577 (±0.069) | -3.032 (±0.154) | 0.997 | 0.025 | 0.763 (±0.030) | -1.865 (±0.068) | 0.998 | 0.009 |
3CN | 1.362 (±0.082) | -2.726 (±0.182) | 0.996 | 0.030 | 1.186 (±0.101) | -2.278 (±0.218) | 0.991 | 0.019 |
4CN | 1.382 (±0.039) | -2.749 (±0.086) | 0.999 | 0.014 | 1.074 (±0.117) | -2.033 (±0.263) | 0.976 | 0.033 |
3Br | 1.958 (±0.115) | -3.151 (±0.251) | 0.991 | 0.043 | 1.780 (±0.105) | -3.105 (±0.238) | 0.991 | 0.030 |
Equations of RM0 - m relationships of examined chloroacetamides in used modifiers
modifier | equation | r | sd | |
---|---|---|---|---|
methanol | polar substituents | RM0 = -0.450 – 1.649m | 0.993 | 0.029 |
nonpolar substituents | RM0 = -1.065 – 1.053m | 0.999 | 0.018 | |
acetone | all substituents | RM0 = -0.595 – 0.760m | 0.981 | 0.075 |
Correlation matrix between various log P-values and chromatographic parameters, RM0 and m.
log P | r | |||||
---|---|---|---|---|---|---|
methanol | acetone | |||||
polar substituents | nonpolar substituents | all substituents | ||||
RM0 | m | RM0 | m | RM0 | m | |
AClog P | 0.976 | 0.971 | 0.992 | 0.991 | 0.976 | 0.970 |
Alog P | --- | --- | 0.990 | 0.985 | 0.958 | 0.934 |
Mlog P | 0.876 | 0.860 | 0.946 | 0.944 | 0.957 | 0.929 |
milog P | 0.973 | 0.985 | 0.996 | 0.992 | 0.982 | 0.981 |
Alog Ps | 0.969 | 0.950 | 0.937 | 0.934 | 0.977 | 0.957 |
log Pch.s | 0.940 | 0.960 | 0.994 | 0.990 | 0.976 | 0.981 |
kowwin | 0.764 | 0.732 | 0.987 | 0.983 | 0.954 | 0.910 |
Xlog P3 | 0.844 | 0.858 | 0.955 | 0.958 | 0.927 | 0.958 |
Calculated pharmacokinetic predictors of the examined chloroacetamides
R | Peff | PPB | log BBB |
---|---|---|---|
H | 3.536 | 45.46 | 0.20 |
4CH3 | 4.243 | 76.38 | 0.31 |
4OCH3 | 3.254 | 64.10 | 0.13 |
4Cl | 4.857 | 80.00 | 0.42 |
4Br | 5.213 | 84.80 | 0.47 |
4F | 4.559 | 63.51 | 0.24 |
4I | 5.579 | 91.06 | 0.58 |
4COCH3 | 3.679 | 61.70 | 0.14 |
4OH | 1.956 | 41.59 | 0.04 |
3CN | 2.366 | 62.42 | 0.03 |
4CN | 3.102 | 62.42 | 0.03 |
3Br | 4.546 | 84.80 | 0.47 |
Correlation matrix between important pharmacokinetic predictors and chromatographic parameters, RM0 and m
pharmacokinetic predictor | r | |||||
---|---|---|---|---|---|---|
methanol | acetone | |||||
polar substituents | nonpolar substituents | all substituents | ||||
RM0 | m | RM0 | m | RM0 | m | |
Peff | 0.940 | 0.914 | 0.940 | 0.938 | 0.964 | 0.934 |
PPB | 0.932 | 0.903 | 0.952 | 0.945 | 0.929 | 0.921 |
log BBB | 0.796 | 0.832 | 0.992 | 0.990 | 0.966 | 0.967 |
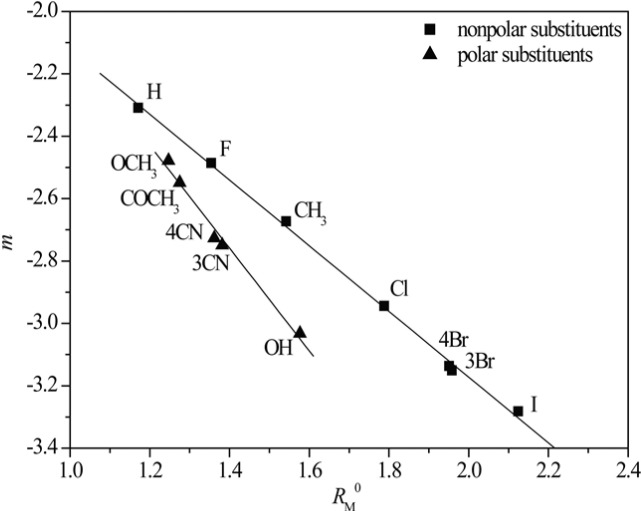
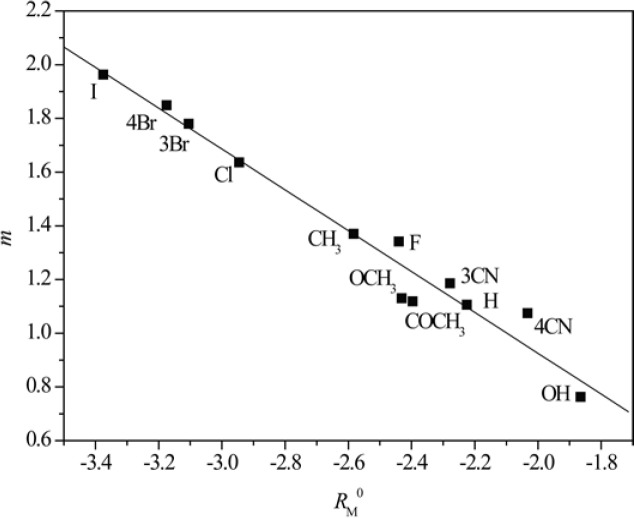
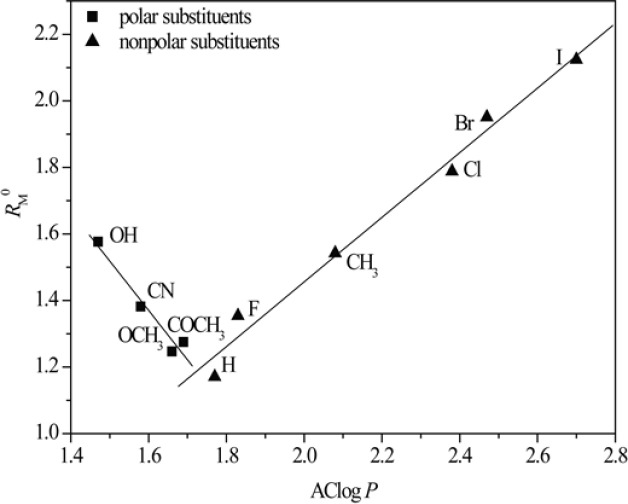
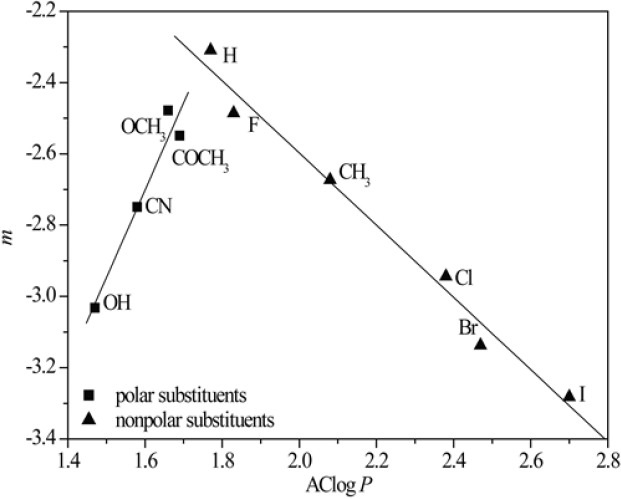
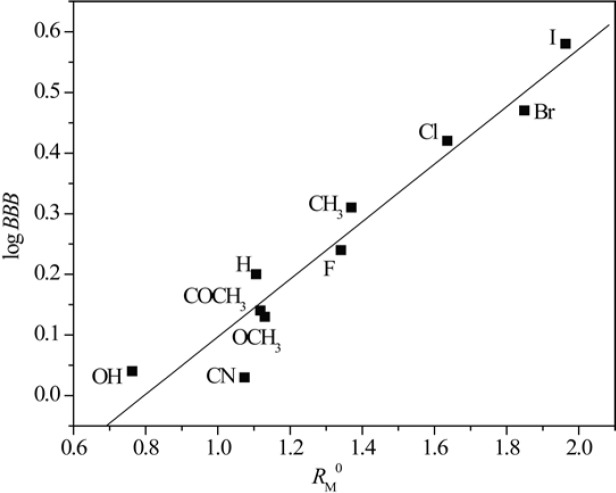
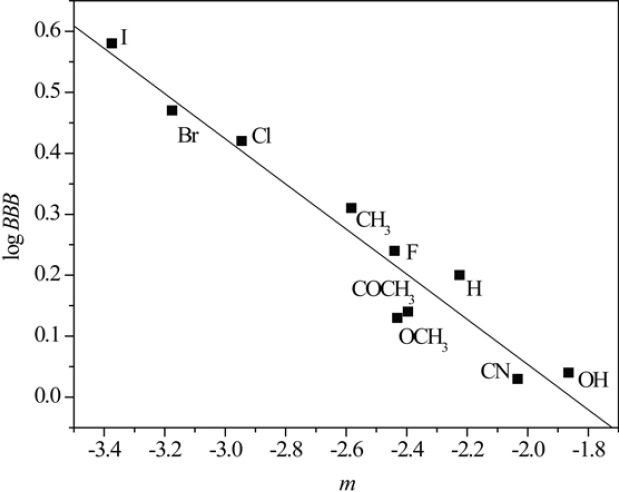
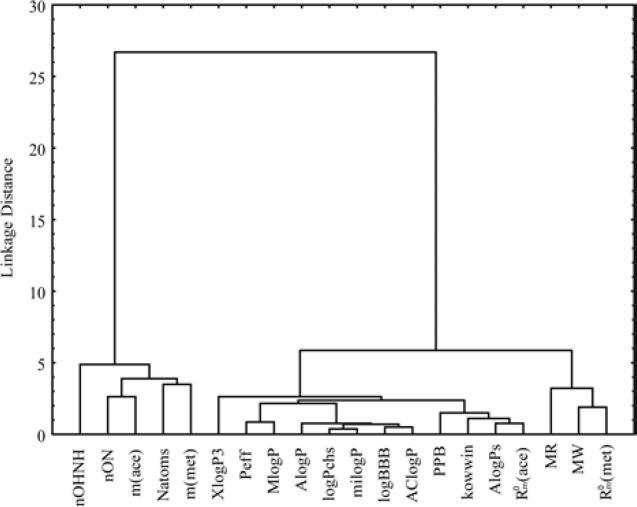
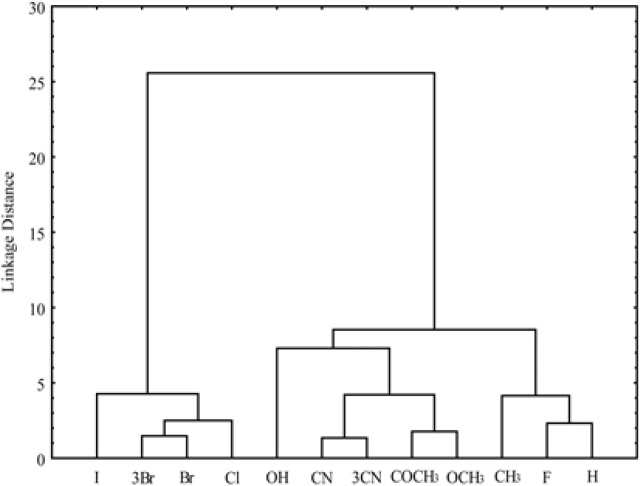
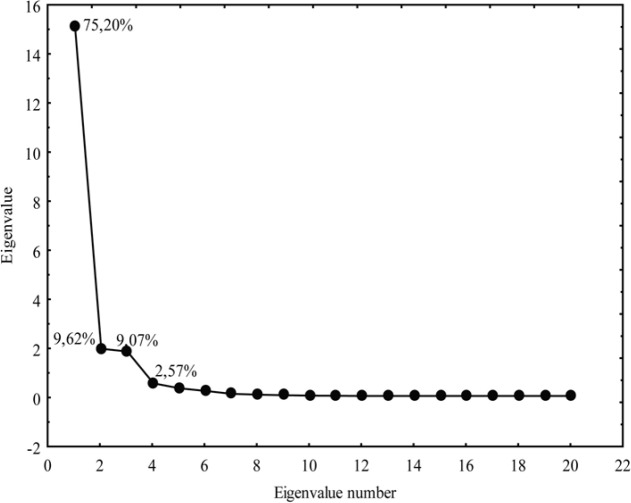
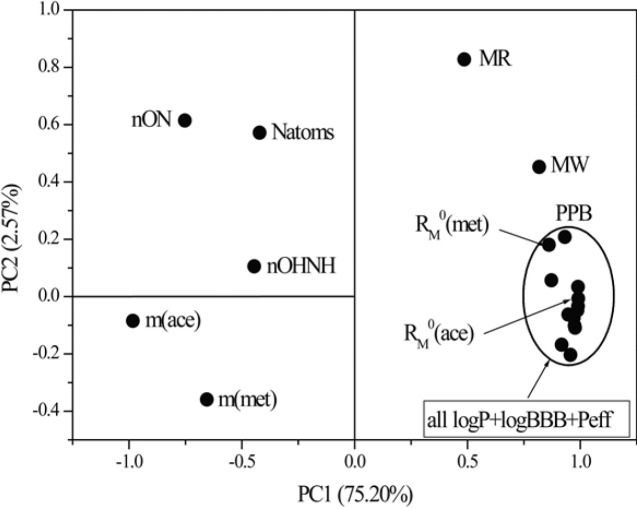
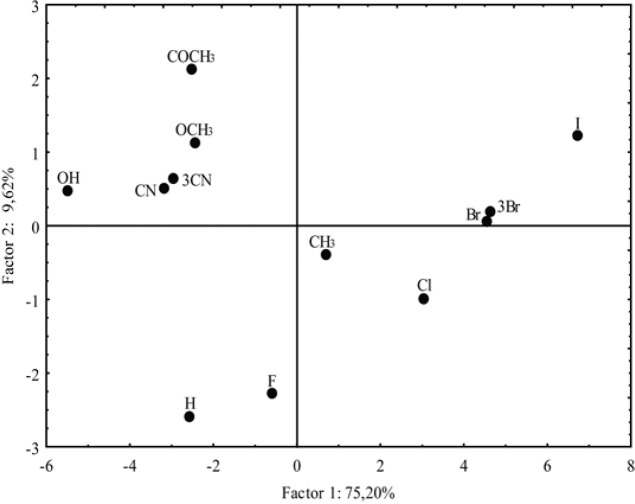
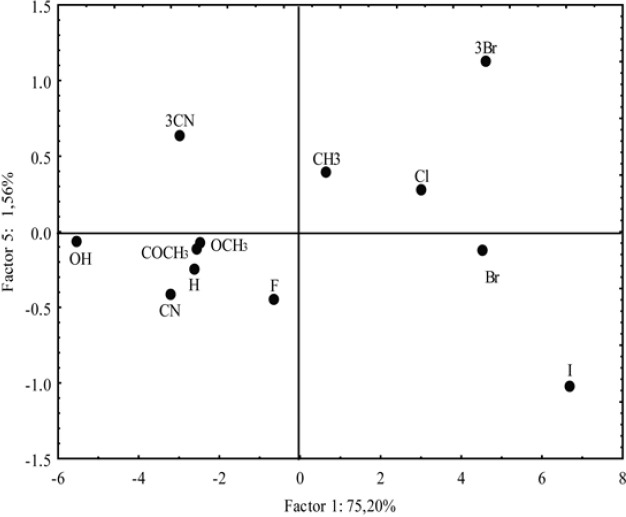
The best agreement with the experimentally determined lipophilicity, parameters RM0 and m was shown by AClog P among all the calculated values of the partition coefficients and the lowest correlation on average was shown by kowwin.
Determination of the pharmacokinetic predictors of chloracetamide derivatives
The early stages of rational design of the biologically active agents require knowledge and understanding of their pharmacokinetics. ADME properties of the compound are crucial for assessing the effectiveness in biological medium (43). One of the most frequent reasons for failure of the bioactive candidates in clinical development is their poor bioavailability. Bioavailability represents the ratio of the unchanged drug administered by the route of interest and after administration direct into the systemic circulation (44). Therefore, it is expected that intravenous administration is preferred (100% bioavailability), but this is often due to practical reasons replaced by oral administration. Consequently, the biologically active compound is subjected to various changes before reaching the site of action and it is necessary to have information about its intestinal absorption. This represents the passage of the compound across the outer mucosal membranes of the gastrointestinal tract (45). The pharmacokinetic parameter that characterizes the rate of human intestinal absorption of compounds after oral administration is the human effective permeability in jejunum, Peff (46). Compounds diffuse through the membrane of enterocytes so their permeability, and hence Peff values increase with their lipophilicity (47).
After reaching the systemic blood circulation, the compound could be more or less bound to plasma proteins. The ratio of its concentration bound to the protein and its total concentration is defined as protein plasma binding affinity, PPB.
Only free fraction of the compound passes through the cell membrane and binds to the appropriate molecular target (48). Hence, the compound’s activity and efficiency depend on the degree and the extent of binding to the plasma proteins. The bound fraction correspond depot which release compound slowly as the unbound form when the unbound form is metabolized. The compound that acts in the central nervous system (CNS) needs to cross the blood–brain barrier (BBB). The possibility of the application of the compound as a potential neurological agent is delineate by the ratio of drug concentration in the brain and drug concentration in the blood log BBB (49). Compounds with log BBB > 0.3 pass through blood brain barrier readily, while log BBB < -1 indicates a blocking of the penetration (50).
Taking into account the significance of knowing the pharmacokinetics in predicting bioactivity of substances, some important pharmacokinetic predictors of the tested chloroacetamide derivatives were calculated applying the software packages Chemsilico and Simulation (Table 7).
As can be seen from Table 7, the most lipophilic derivative (-I as substituent) has the highest value of Peff and as it was expected the lowest absorption evince the most polar compound (-OH group as substituent). Furthermore, while reviewing the values of PPB, it was noticed that the compound with -I as substituent exhibits the greatest affinity for binding to plasma proteins. The largest amounts of the unbound compound arrive to the surrounding tissue in the case of derivative with -OH group. Also, the derivatives with alkyl and halogen substituents show the greatest possibility of application as neurological agents (log BBB > 0.3).
Correlation of pharmacokinetic predictors and chromatographic parameters, RM0 and m
Taking into account the fact that the lipophilicity of the compound is closely related to its pharmacokinetics, chromatographic parameters, RM0 and m, as the presumed measures of lipophilicity of the tested chloroacetamides were correlated with three pharmacokinetic predictors Peff, PPB and log BBB applying linear regression analysis. Figures 5 and 6 illustrate the obtained correlations of RM0 and m determined in acetone with pharmacokinetic predictor log BBB and results of all established relationships are presented in Table 8.
Figures 5 and 6 as well as data in Table 8 confirm that linear regression analysis gave satisfactory correlations of RM0 and m with the selected pharmacokinetic predictors. Also, again it can be observed separating of compounds in methanol which is previously explained.
From results represented in Table 8, it can be seen that the chromatographic parameter, RM0 obtained in both used modifiers achieve better relationships with all pharmacokinetic predictors than parameter m. Also, among all the calculated predictors, Peff gave the highest agreement with both chromatographic parameters. The obtained results indicates that RPTLC parameters could be used successfully in preliminary tests of potential bioactivity of N-(substituted phenyl)-2-chloroacetamides.
Establishing relationships between different indicators of bioactivity applying multivariate methods
In addition to the method of linear regression, in order to gain a better insight into the relationship between the investigated molecular descriptors, experimentally determined measures of lipophilicity, chromatographic retention parameters, RM0and m, were interconnected with the standard measure of lipophilicity, the partition coefficient, log P, important pharmacokinetic predictors and parameters of Lipinski and Ghose, respectively, by applying multivariate analysis. Multivariate methods enable the analysis and classification of numerous experimental results obtained in different ways and the establishing of the relationship between them based on their (dis)similarities. Among the methods of multivariate analysis significantly stand out cluster analysis, CA, and principal component analysis, PCA (51-55).
The data matrix for performing CA and the PCA analysis consists of the columns (variables) which correspond to experimentally and mathematically obtained lipophilicity, pharmacokinetic predictors, parameters of Lipinski and Ghose, while examined chloroacetamide derivatives represented the rows (cases). Prior to the analysis, the data matrix was standardized, because the analyzed data were measured on different scales.
As the measure of dissimilarity between objects, in CA the Euclidean distance was used, while the Ward’s linkage method was applied for testing the linkage measure. As results of cluster analysis for different parameters of bioactivity was obtained dendrogram which is shown in Figure 7 and the other one for the investigated chloroacetamides presented in Figure 8.
Figure 7 shows the separation of the analyzed data into two clusters. The first cluster contains the chromatographic parameter m (obtained in both used modifiers) and parameters of Lipinski and Ghose which mainly refer to the number of atoms (total, hydrogen bond acceptors, hydrogen bond donors). This confirmed the already known phenomenon that the parameter m is directly related to the characteristics of solute (39).
In the second cluster the rest of lipophilicity parameters are placed, all pharmacokinetic predictors and MW, MR. Inside the second cluster subcluster can be noticed, which contain MW, MR and chromatographic constant RM0 obtained in methanol. Separation of MW and MR from other parameters of Lipinski and Ghose confirms the fact that these two properties are more related to biomolecular interactions, hence theoretically influences the lipophilicity (MW) and pharmacokinetic behavior (MR) of the compound (56). Also, it is noticeable that the apartness of the chromatographic parameter m from chromatographic retention constant RM0 and log P what was registered in the literature earlier (57). This grouping of the obtained lipophilic parameters indicates a higher similarity of the chromatographic retention constant RM0 to the log P and a greater influence on the pharmacokinetic predictors than the chromatographic parameter m at given conditions. The cluster analysis of chloroacetamides which can show the influence of nature of the substituents in the molecules on their bioactivity, was resulted with dendrogam which has two clearly defined clusters (Figure 8). In the first cluster derivatives having halogen as substituent are grouped, while the second contains the rest of derivatives. From Figure 8 it can also be noted that the second cluster is divided into two subclusters. One subclusters includes the compounds with polar substituents (-OH, -CN, 3CN, COCH3, OCH3) and in the other are the unsubstituted compound and derivatives with -CH3 and -F as substituents. The derivative with -F as substituent is secluded from other derivatives with halogen substituents given which has a high polarization force.
Besides the cluster analysis, the principal component analysis was conducted. It is a significant multivariate method since it provides identification and elimination of the redundant data from the experimental results, therefore ensuring the required reduction in their number with a minimal wastage of information. By PCA the original data matrix was decomposed into loading (lipophilic parameters, pharmacokinetic predictors, parameters of Lipinski and Ghose) and score (tested chloroacetamides) vectors, whereby new variables-principal components were obtained (Figure 9).
As can be observed from Figure 9, five principal components have described 98.02% (PC1 75.20%, PC2 9.62%, PC3 9.07%, PC4 2.57% and PC5 1.56%) of total variance in the data.
By comparing the obtained PCs of variables (parameters of Lipinski and Ghose, experimentally and mathematically determined lipophilicity and pharmacokinetic predictors), the loading plots were obtained (Figure 10).
This dependence of the two first principal components resulted in the separation of the examined descriptors into two characteristic groups based on the PC1 values. One group is described by the negative PC1 and includes m values, nON, nOHNH and natoms thus demonstrating already observed connection between them. The second involves mathematically obtained log P-values, chromatographic parameters RM0 determined in both used modifiers, pharmacokinetic predictors, MW and MR (positive PC1). By this has been confirmed again that chromatographic constant RM0 corresponds to the parameter log P and to the selected pharmacokinetic predictors in greater extent than parameter m. Such distribution of parameters confirmed the results previously detected by the cluster analysis. As can be seen from Figure 10 within the existing group, the PC2 value can classify the parameters in finer way according to the nature of the information they carry. The chromatographic parameter m obtained in both applied modifiers has negative PC2 and stand apart from the theoretical parameters relating to the number of atoms. On the other hand a very close grouping can be observed of chromatographic constant, RM0 obtained in both of the used modifiers with standard lipophilic parameters and pharmacokinetic predictors. A slightly greater similarity is thereby registered in the case of RM0 obtained in acetone than in methanol. Descriptors MW and MR in the loading plots appear as outliers.
In addition, the PCA enabled important classification of the investigated chloroacetamide derivatives based on differences in their lipophilicity and pharmacokinetics. The correlation of the two main components for the analyzed chloroacetamides (score plot) is illustrated in Figure 11. The nature of the substituent -R caused grouping of the compounds which was registered in literature (58).
Differences between them are reflected in the value of PC1. One group includes derivatives with polar substituents and negative values of PC1, while the second group encompasses compounds with non-polar substituents (except -H and -F) and they are characterized by the positive PC1. The most negative value of PC1 has a compound with -OH group as a substituent and the most positive PC1 is registered for the most lipophilic compound, with more voluminous halogen substituent (-I as substituent). It is obvious that the compounds with the negative value PC1 at the same time are divided based on the value of PC2. Thus, derivatives with polar substituents (positive PC2) are separated from the compounds with -H and -F (negative PC2). Again, the results obtained by the PCA analysis are very similar to the CA results, but the PCA is more subtle given that the derivative with a -CH3 substituent is classified as lipophilic.
Other interdependencies between the main components did not give any significant distribution of the examined compounds. However, regardless of the small value of the PC5 (1.56%) the important distribution of the analyzed chloroacetamides by PC1-PC5 correlation was registered (Figure 12).
Namely, derivatives with -Br and -CN in position 3 (positive PC5) are separated from the derivatives with same substituent in position 4 (negative PC5). Thereby, it is confirmed that the additional advantage of the PCA is representing its high sensitivity for detecting extremely small differences between the compounds with the same substituent in different positions in which the experimental and theoretical methods for determining lipophilicity with sufficient visibility could not be done.
Conclusion
Derivatives of N-(substituted phenyl)-2-chloroacetamides were studied with the goal of predicting their potential biological activity by fulfilling the rules of bioactivity (Lipinski and Ghose) and determining their lipophilicity, experimentally by RPTLC in presence of methanol and acetone, and computationally by applying software package.
By applying the classic linear regression analysis and chemometric methods (CA and PCA) the presumed measures of lipophilicity (biological activity) such as chromatographic retention parameter, RM0 and m, were found to be in a good relationship with partition coefficient, log P, as a standard criteria of lipophilicity (average correlation coefficient, r = 0.947) and with significant pharmacokinetic predictors, Peff, PPB and log BBB (average correlation coefficient, r = 0.931). More concretely, the chromatographic retention constant, RM0 gave stronger dependencies under the existing conditions with the used descriptors than parameter m. In addition, the multivariate methods provided an essential sensitivity for the distribution of data according to their similarities and differences which could be decisive in the preliminary tests of the biological potential of the compounds.
The results of all the conducted correlation methods pointed that the chromatographic parameters, RM0 and m, determined by applying RPTLC offer the possibility for a more complete understanding and reliable prediction of properties (lipohilicity, pharmacokinetics, bioavailability) which indicate the biological activity of N-(substituted phenyl)-2-chloroacetamides at the earliest phase of modern design of bio agents.
Acknowledgements
References
-
1.
Calderone V, Fiamingo FL, Amato G, Giorgi I, Livi O, Martelli A, Martinotti E. 1,2,3-Triazol-carboxanilides and 1,2,3-triazol-(N-benzyl)-carboxamides as BK-potassium channel activators. XII. Eur. J. Med. Chem. 2008;43:2618-26. [PubMed ID: 18400336].
-
2.
Urbahns K, Woltering M, Nikolic S, Pernerstorfer J, Bischoff H, Dittrich-Wengenroth E, Lustig K. Glycine amides as PPARα agonists. Bioorg. Med. Chem. Lett. 2010;20:3376-9. [PubMed ID: 20452212].
-
3.
Banerjee PS, Sharma PK. New antiepileptic agents: Structure-activity relationships. Med. Chem. Res. 2012;21:1491-508.
-
4.
Choi M, Jo H, Park HJ, Sateesh Kumar A, Lee J, Yun J, Kim Y, Han SB, Jung JK, Cho J, Lee K, Kwak JH, Lee H. Design, synthesis, and biological evaluation of benzofuran- and 2,3-dihydrobenzofuran-2-carboxylic acid N-(substituted)phenylamide derivatives as anticancer agents and inhibitors of NF-κB. Bioorg. Med. Chem. Lett. 2015;25:2545-9. [PubMed ID: 25953156].
-
5.
Papadopoulou MV, Bloomer WD, Rosenzweig HS, Wilkinson SR, Kaiser M. Novel nitro(triazole/imidazole)-based heteroarylamides/sulfonamides as potential antitrypanosomal agents. Eur. J. Med. Chem. 2014;87:79-88. [PubMed ID: 25240098].
-
6.
Bardiot D, Thevissen K, De Brucker K, Peeters A, Cos P, Taborda CP, McNaughton M, Maes L, Chaltin P, Cammue BPA, Marchand A. 2-(2-Oxo-morpholin-3-yl)-acetamide derivatives as broad-spectrum antifungal agents. Eur. J. Med. Chem. 2015;58:1502-12.
-
7.
Wan R, Zhang JQ, Han F, Wang P, Yu P, He Q. Synthesis and insecticidal activities of novel 1,3,4-thiadiazole 5-fluorouracil acetamides derivatives: An RNA interference insecticide. Nucleosides Nucleotides Nucleic Acids. 2011;30:280-92. [PubMed ID: 21623542].
-
8.
Arutyunyan NS, Akopyan LA, Nazaryan RL, Gevorgyan GA, Stepanyan GM, Paronikyan RV, Panosyan GA. Synthesis and antibacterial activity of oxalates and acetamides of {2-[2-Sopropyltetrahydropyran-4-yl-4-(4-luorophenyl)]Ethyl}Arylamines. Pharm. Chem. J. 2013;47:490-3.
-
9.
Consalvi S, Alfonso S, Di Capua A, Poce G, Pirolli A, Sabatino M, Ragno R, Anzini M, Sartini S, La Motta C, Di Cesare Mannelli L, Ghelardini C, Biava M. Synthesis, biological evaluation and docking analysis of a new series of methylsulfonyl and sulfamoyl acetamides and ethyl acetates as potent COX-2 inhibitors. Bioorg. Med. Chem. Lett. 2015;23:810-20.
-
10.
Ali R, Siddiqui N. New benzo[d]thiazol-2-yl-aminoacetamides as potential anticonvulsants: synthesis, activity and prediction of molecular properties. Arch. Pharm. 2015;348:254-65.
-
11.
Saeedi M, Goli F, Mahdavi M, Dehghan G, Faramarzi MA, Foroumadi A, Shafiee A. Synthesis and biological investigation of some novel sulfonamide and amide derivatives containing coumarin moieties. Iran. J. Pharm. Res. 2014;13:881-92. [PubMed ID: 25276188].
-
12.
Addla D, Jallapally A, Gurram D, Yogeeswari P, Sriram D, Kantevari S. Design, synthesis and evaluation of 1,2,3-triazole-adamantylacetamide hybrids as potent inhibitors of Mycobacterium tuberculosis. Bioorg. Med. Chem. Lett. 2014;24:1974-9. [PubMed ID: 24679703].
-
13.
Shruthi N, Poojary B, Kumar V, Prathibha A, Hussain MM, Revanasiddappa BC, Joshi H. Synthesis and biological evaluation of N-(substituted phenyl)-2-(5H-[1,2,4]triazino[5,6-b]indol-3-ylsulfanyl)acetamides as antimicrobial, antidepressant, and anticonvulsant agents. Russ. J. Bioorg. Chem. 2015;41:223-30.
-
14.
Knaack M, Emig P, Bats JW, Kiesel M, Müller A, Günther E. Synthesis and characterization of the biologically active 2-[1-(4-chlorobenzyl)-1H-indol-3-yl]-2-oxo-N-pyridin-4-yl acetamide. Eur. J. Org. Chem. 2001;20:3843-7.
-
15.
Ghorab MM, Alsaid MS, Ghabour HA, Fun HK. Synthesis, crystal structure and antitumor activity of novel 2-cyano-N-(quinolin-3-yl)acetamide. Asian J. Chem. 2014;26:7389-92.
-
16.
Chiou CT, Lee WC, Liao JH, Cheng JJ, Lin LC, Chen CY, Song JS, Wu MH, Shia KS, Li WT. Synthesis and evaluation of 3-ylideneoxindole acetamides as potent anticancer agents. Eur. J. Med. Chem. 2015;98:1-12. [PubMed ID: 25988923].
-
17.
Tran PT, Kim HS, Ann J, Kim SE, Kim C, Hong M, Hoang VH, Ngo VTH, Hong S, Cui M, Choi S, Blumberg PM, Frank-Foltyn R, Bahrenberg G, Stockhausen H, Christoph T, Lee J. α-Substituted 2-(3-fluoro-4-methylsulfonamidophenyl)acetamides as potent TRPV1 antagonists. Bioorg. Med. Chem. Lett. 2015;15:2326-30.
-
18.
Orr STM, Beveridge R, Bhattacharya SK, Cameron KO, Coffey S, Fernando D, Hepworth D, Jackson MV, Khot V, Kosa R, Lapham K, Loria PM, McClure KF, Patel J, Rose C, Saenz J, Stock IA, Storer G, Von Volkenburg M, Vrieze D, Wang G, Xiao J, Zhang Y. Evaluation and synthesis of polar aryl- and heteroaryl spiroazetidine-piperidine acetamides as ghrelin inverse agonists. ACS Med. Chem. Lett. 2015;6:156-61. [PubMed ID: 25699143].
-
19.
Moorthy NSHN, Ramos MJ, Fernandes PA. Structural analysis of 2-piperidin-4-yl-actamide derivatives for hERG blocking and MCH R1 antagonistic activities. Curr. Drug Discov. Technol. 2012;9:25-38. [PubMed ID: 22235926].
-
20.
Paul SM, Mytelka DS, Dunwiddie CT, Persinger CC, Munos BH, Lindborg SR, Schacht AL. How to improve RD productivity: The pharmaceutical industry’s grand challenge. Nat. Rev. Drug Discov. 2010;9:203-14. [PubMed ID: 20168317].
-
21.
Ghose AK, Viswanadhan VN, Wendoloski JJ. A knowledge-based approach in designing combinatorial or medicinal chemistry libraries for drug discovery 1 A qualitative and quantitative characterization of known drug databases. J. Comb. Chem. 1999;1:55-68. [PubMed ID: 10746014].
-
22.
Lipinski CA. Lead- and drug-like compounds: The rule-of-five revolution. Drug Discov. Today Technol. 2004;1:337-41. [PubMed ID: 24981612].
-
23.
Ece A, Sevin F. The discovery of potential cyclin A/CDK2 inhibitors: A combination of 3D QSAR pharmacophore modeling, virtual screening, and molecular docking studies. Med. Chem. Res. 2013;22:5832-43.
-
24.
Georgey H. Synthesis, cytotoxic activity and 2D-QSAR study of some imidazoquinazoline derivatives. Molecules. 2014;19:3777-92. [PubMed ID: 24662088].
-
25.
Zhou C, Zou F, Xu Y, Zhang L, Zha X. Identification of new non-steroidal TGR5 agonists using virtual screening with combined pharmacophore models. Med. Chem. Res. 2015;24:2561-72.
-
26.
Fujita T, Iwasa J, Hansch CA. New substituent constant, π, derived from partition coefficients. J. Am. Chem. Soc. 1964;86:5175-80.
-
27.
Leo A, Hansch C, Elkins D. Partition coefficients and their uses. Chem. Rev. 1971;7:525-616.
-
28.
Kalász H, Benko B, Gulyás Zs, Tekes K. Lipophilicity determination using both TLC and calculations. J. Liq. Chromatogr. Relat. Technol. 2009;32:1342-58.
-
29.
Oros Gy, Cserháti T. Relationship between the calculated physicochemical parameters and reversed phase thin-layer chromatographic retention behavior of carboxamide fungicides and related compounds. J. Liq. Chromatogr. Relat. Technol. 2010;33:880-93.
-
30.
Vaštag D, Perišić-Janjić N, Tomić J, Petrović S. Evaluation of the lipophilicity and prediction of biological activity of some N-cyclohexyl-N-substituted-2-phenylacetamide derivatives using RP-TLC. J. Planar Chromatogr. -Mod. TLC. 2011;24:435-40.
-
31.
Odović J, Karljiković-Rajić K, Trbojević-Stanković J, Stojimirović B, Vladimirov S. The lipophilicity examination of some ACE inhibitors and hydrochlorothiazide on cellulose in RP thin-layer chromatography. Iran. J. Pharm. Res. 2012;11:763-70. [PubMed ID: 24250503].
-
32.
Tache F, Naşcu-Briciu RD, Sârbu C, Micǎle F, Medvedovici A. Estimation of the lipophilic character of flavonoids from the retention behavior in reversed phase liquid chromatography on different stationary phases: A comparative study. J. Pharm. Biomed. Anal. 2012;57:82-93. [PubMed ID: 21945453].
-
33.
Waterhouse RN. Determination of lipophilicity and its use as a predictor of blood-brain barrier penetration of molecular imaging agents. Mol. Imaging Biol. 2003;5:376-89. [PubMed ID: 14667492].
-
34.
Milošević N, Janjić N, Milić N, Milanović M, Popović J, Antonović D. Pharmacokinetics and toxicity predictors of new s-triazines, herbicide candidates, in correlation with chromatogrpahic retention constants. J. Agric. Food Chem. 2014;62:8579-85. [PubMed ID: 25093448].
-
35.
-
36.
-
37.
-
38.
-
39.
Szymański P, Skibiński R, Liszka M, Jargieło I, Mikiciuk-Olasik E, Komsta Ł. A TLC study of the lipophilicity of thirty-two acetylcholinesterase inhibitors - 1,2,3,4-tetrahydroacridine and 2,3-dihydro- 1H-cyclopenta[b] quinoline derivatives. Cent. Eur. J. Chem. 2013;11:927-34.
-
40.
Sârbu C, Onişor C, Posa M, Kevresan S, Kuhajda K. Modeling and prediction (correction) of partition coefficients of bile acids and their derivatives by multivariate regression methods. Talanta. 2008;75:651-7. [PubMed ID: 18585127].
-
41.
Oros Gy, Cserháti T. Relationship between the calculated physicochemical parameters and reversed phase thin-layer chromatographic retention behavior of carboxamide fungicides and related compounds. J. Liq. Chromatogr. Relat. Technol. 2010;33:880-93.
-
42.
Biagi GL, Barbaro AM, Sapone A, Recanatini M. Determination of lipophilicity by means of reversed-phase thin-layer chromatography: I Basic aspects and relationship between slope and intercept of TLC equations. J. Chromatogr. A. 1994;662:341-61.
-
43.
Wishart DS. Improving early drug discovery through ADME modelling: An overview. Drugs R D. 2007;8:349-62. [PubMed ID: 17963426].
-
44.
Logan C. Use of animals for the determination of absorption and bioavailability. In: van de Waterbeemd H, Lennernäs H, Artursson P, editors. Drug Bioavailability: Estimation of Solubility, Permeability, Absorption and Bioavailability. Weinheim: Wiley-VCH Verlag GmbH & Co; 2003. p. 132-58.
-
45.
Chiou WL. The rate and extent of oral bioavailability versus the rate and extent of oral absorption: Clarification and recommendation of terminology. J. Pharmacokinet. Pharmacodyn. 2001;28:3-6. [PubMed ID: 11253613].
-
46.
Lennernäs H. Human intestinal permeability. J. Pharm. Sci. 1998;87:403-10. [PubMed ID: 9548891].
-
47.
Zakeri-Milani P, Tajerzadeh H, Islambolchilar Z, Barzegar S, Valizadeh H. The relation between molecular properties of drugs and their transport across the intestinal membrane. DARU. 2006;14:164-71.
-
48.
van de Waterbeemd H, Gifford E. ADMET in-silico modelling: Towards prediction paradise? Nat. Rev. Drug Discov. 2003;2:192-204.
-
49.
Pajouhesh H, Lenz GR. Medicinal chemical properties of successful central nervous system drugs. NeuroRx. 2005;2:541-53. [PubMed ID: 16489364].
-
50.
Vilar S, Chakrabarti M, Costanzi S. Prediction of passive blood-brain partitioning: Straightforward and effective classification models based on in-silico derived physicochemical descriptors. J. Mol. Graph. Model. 2010;28:899-903. [PubMed ID: 20427217].
-
51.
Komsta L, Kobyłka M. Chemometric approach to selectivity in TLC with densitometric detection. J. Chromatogr. Sci. 2013;51:400-5. [PubMed ID: 23035205].
-
52.
Vastag GyGy, Apostolov SLj, Matijević BM, Marinković AD. Chemometric approach in studying of the retention behavior and lipophilicity of potentially biologically active N-substituted-2-phenylacetamide derivatives. J. Braz. Chem. Soc. 2014;25:1948-55.
-
53.
Jevrić LR, Podunavac-Kuzmanović SO, Švarc-Gajić JV, Kovačević SZ, Jovanović BŽ. RP-HPTLC retention data in correlation with the in-silico ADME properties of a series of s-triazine derivatives. Iran. J. Pharm. Res. 2014;13:1203-11. [PubMed ID: 25587308].
-
54.
Kovačević SZ, Jevrić LR, Podunavac-Kuzmanović SO, Lončar ES. Prediction of in-silico ADME properties of 1,2-o-isopropylidene aldohexose derivatives. Iran. J. Pharm. Res. 2014;13:899-908. [PubMed ID: 25276190].
-
55.
Andrić F, Héberger K. Chromatographic and computational assessment of lipophilicity using sum of ranking differences and generalized pair-correlation. J. Chromatogr. A. 2015;1380:130-8. [PubMed ID: 25595531].
-
56.
Viswanadhan VN, Ghose AK, Revankar GR, Robins RK. Atomic physicochemical parameters for three dimensional structure directed quantitative structure-activity relationships Additional parameters for hydrophobic and dispersive interactions and their application for an automated superposition of certain naturally occurring nucleoside antibiotics. J. Chem. Inf. Model. 1989;29:163-72.
-
57.
Vastag Gy, Apostolov S, Matijević B, Petrović S. Establishing dependences between different lipophilic parameters, of new potentially biologically active N-substituted-2-phenylacetamide derivatives by applying multivariate methods. J. Chromatogr. Sci. 2015;53:312-9. [PubMed ID: 24981978].
-
58.
Vastag G, Apostolov S, Perišić-Janjić N, Matijević B. Multivariate analysis of chromatographic retention data and lipophilicity of phenylacetamide derivatives. Anal. Chim. Acta. 2013;767:44-9. [PubMed ID: 23452785].