Abstract
Keywords
Insulin Insulin receptor HADDOCK Conformational changes Salt bridges
Introduction
Diabetes mellitus is one of the most significant diseases, resulting from severe metabolic imbalances. Regarding this issue, researchers pay attention to pancreas which involves in creating oxidative stress (1). Insufficient levels of insulin or inhibition of antioxidants enzymes such as superoxide dismutase in diabetic patients cause oxidative stress and may damage or kill cells (2).
Insulin is a peptide hormone composed of 51 amino acids with a molecular weight of 5808 Da. It contains two chains: a 21 residue A-chain and a 30 residue B-chain joined by two disulfide bonds. Insulin plays a significant role in regulating carbohydrate and fat metabolism in animal cells. By initiating and promoting biosynthesis and storage of carbohydrates, lipids and proteins, insulin inhibits their degradation and is released back into the blood circulation (3).
Insulin is produced by beta cells in the islets of Langerhans. The Hexamer form of insulin often incorporates zinc which makes it more stable, but it is released into the blood stream as a zinc free monomer. After the release, insulin binds to its receptor on target cells (4-6).
Insulin receptor (IR) is a membrane protein that starts a cascade of events in the presence of insulin (5, 7). IR is a disulfide-bound (αβ)2 homodimer protein composed of two regions including two extracellular subunits with 135 KD R-subunits and two 95 KD transmembrane β- subunits (5, 8).
The extracellular portion of each αβ subunit, i.e., the ectodomain, contains six domains including a leucine-rich repeat domain, L1 ectodomain, a cycteine-rich domain, CR, a second leucine-rich repeat domain L2 and three fibronectin type-III domains: FnIII-1, FnIII-2, and FnIII-3. There is also an insert domain, ID with FnIII-2. The α-chain component of the ID is terminated by a segment known as αCT (α-chain) (α-chain of carboxyl terminal) which consists of residues 704-719 (4, 8, 9).
In the presence of insulin, the IR ectodomain is folded into an inverted ‘V’ shape. Each leg of the inverted ‘V’ consists of the L1-CR-L2 module in an extended conformation juxtaposed against an extended linear arrangement of the three fibronectine two-fold symmetry. At the apex of the inverted ‘V’, the L2 domain of each monomer interacts with the FnIII-1 domain of the another monomer, while at the midpoint of the legs, the L1 domain of each monomer is in contact with the FnIII-2 domain of the secondary monomer (7, 10, 11). In the literature, to distinguish the IR monomers, one of them is denoted by “ ′ “.Unfortunately, the total crystal structure of IR is not available. Michael C. Lawrence and co-workers have released several crystal structures of the insulin receptor from 2006 to 2013 (such as Protein Data Bank accession code 2DTG, 3LOH and 3W11) which were derived from X-ray diffraction data (8).
In the current model of insulin-receptor complex, the binding is mediated by two adjoining structural regions: a low affinity site1 and a high affinity site2 (9).
Extensive studies on mutant receptors have established that site 1 is composed of two distinct regions: i) the center of three β-sheets of L1 domain and the central modules in the CR region of the first monomer, ii) the last 16 residues of the α-CT of the other monomer. Site 2 is possibly formed by loops at the junction of the FnIII-1 and FnIII-2 domains of the opposite monomer (12).
There are two pockets found on the insulin receptor molecule. One formed by L1-CR-L2 (site 1) and (F1-F2-F3)′ (site 2′) domains and the other one formed by (L1-CR-L2)′ (site 1′) and (F1-F2-F3) (site 2) domains.
As mentioned above, there is just one pocket on each leg of IR dimer. The current belief for insulin/receptor binding mechanism is as follows: one insulin molecule docks at the accessible pocket and causes two monomers to close up and to open up the opposite site, making it accessible to other insulin molecules. This situation leads to accelerate dissociation of the first insulin at the site 1/site 2′ (first pocket) (12).
Although several studies have tried to clarify the interaction between insulin and its receptor, the mechanism of this interaction remains elusive because of the receptor’s structural complexity and structural changes during the interaction. In this work, we tried to fractionate the interactions. Therefore, sequential docking method utilization of HADDOCK was used to achieve the mentioned goal.
Experimental
Input files
Input coordinates for human insulin were obtained from the RCSB Data Bank (PDB code 3INC). The IR’s ectodomain which was used in this study contains domains L1, CR, L2, F1, F2 and F3, but lacks a part of the insert domain (residues 656-692 and 711-754) including the CT peptide. Input coordinates for the human IR were obtained from 3.8 Å and 3.9 Å resolution X-ray crystallographic data which include PDB code 3LOH and 3W11, respectively (9). After combination two pdb files of IR using modeler, several servers were used to evaluate the final model such as PROCHECK (13), QMEAN (14), and RAMPAGE (15).
Because of inability of crystallography methods to determine a complete structure of α-CT of IR, 3LOH and 3W11 pdb files were concatenated using modeler 9.12 to create a pdb file contains residues 693-715. A default template has not been determined for residues 716-719 (8).
Determination of the hinge regions
The hinge prediction server, Hinge Prot, was used to predict hinge regions of flexible monomers. HingeProt annotates rigid parts and possible hinges of the supplied protein based on two Elastic Network Models (GNM) (16) and Anisotropic Network Model (ANM) (17).
In this work, each monomer of IR was dissected by HingeProt server and two different sets of output files were provided, i.e., rigid parts, and Hinge residues (Table 1). The detailed procedure is described in the result section. HingeProt cluster the fragments into structurally compact rigid and score the obtained prediction defined based on the reference (18).
Docking protocol of HADDOCK
In order to simulate insulin/IR’s model, we focused on our data-driven docking approach, i.e., HADDOCK using sequential docking method (19-21). HADDOCK is an information- driven flexible docking approach for the modeling of bimolecular complexes. It can account for conformational changes occurring upon binding using explicit flexible during the molecular dynamics refinement. Since molecular dynamics isn’t really suited for docking purpose because sampling in docking would be extremely computational expensive, so HADDOCK could be used to refine model (20).
The docking protocol comprise of three steps, a rigid-body energy minimization, a semi-flexible refinement in torsion angle space and a final refinement in explicit solvent (20). Based on Michael’s literature (22) we decided to choose the order of components involving in the interaction. Insulin bonded to all components of IR according to the following order: L1 domain (first monomer), α-CT, FIII-1, FIII-2, FIII-3 and L1 domains (second monomer), α-CT and FIII-1, FIII-2, FIII-3 domains of the first monomer. We also evaluated simultaneously docking method as an alternative approach, but the result has a lower compatible with experimental data that confirms the order of components (data not shown). Work flows of the sequential docking method have been shown in Figure 1.
Sequential docking method
After uploading pdb file of IR resulting from HingeProt step by step and insulin pdb file, several parameters were changed in HADDOCK according to the following steps; ambiguous restraints of guru-interface were determined based on the active and passive residues resulting from the literature (8, 23) using HADDOCK (http://haddock.science.uu.nl/services/GenTBL/). Unambiguous restraints manually created a restraint between salt bridges and in the hinge regions of IR homodimer. “Center of mass restraints” between domains were turned off and AIRs (Randomly exclude a fraction of the ambiguous restraints) was turned on. “The number of structures” was increased to 10000, 400, and 400 for it0, it1 and water, respectively. The rest of the parameters were left to their default values (24).
To analysis hydrophobic interaction and hydrogen bond, Ligplot was used (25). Secondary structure was evaluated by stride server (26). In order to determine salt bridge and visualization of our model, VMDv 1.9.2 was used (27).
Results and Discussions
Considering a docking based on HADDOCK, we constructed the structural model of an IR homodimer/insulin complex in the presence of insulin pdb file (code: 3INC) and homology modeling based on 3LOH and 3W11 PDBs.
Our built model showed new salt bridges (i) Asp17 salt bridges to Arg554 (F1), the same residue salt bridges to Lys 484 (F1) (ii) the side chain of Lys50 salt bridges to Asp707 (α-CT) (Table 2 and Figure 2) (iii) the side chains of Asp499 (F1) interacted with Arg371 (L2) (iv) while the side chain of Arg345 (L2) interacted with Asp535 (F1) (v) the side chain of Lys166 (L1) interacted with Asp645 (F2) (Table 3 and Figure 3).
New Cartoons, and the A- and B-chain of insulin are secondary structure NewCartoons. New salt bridges between insulin and its receptor depicted as vdw (zoomed view). Blue vdw indicates K50 on insulin which interact with D707 (α-CT; yellow vdw), red vdw corresponds D17 on insulin had salt bridge with R554 (F1; orange vdw) and K484 (F1, pink vdw).
To survey the important role of salt bridges, rigid parts 2 and 3 resulting from HingeProt were added as two separate PDB files. To keep the distance between the mentioned rigid parts in the range of covalent bond, i.e., 1.4 ± 0.2, a restraint was defined in their connected hinge and three restraints defined for salt bridges between rigid part 3 and rigid part 1 (L1) of opposite monomer. Following docking, the distance between two rigid parts was in the range of 50 Å indicating the power of salt bridges to expose rigid part 3 towards L1 on the opposite monomer (Figure 4).
To tackle this high distance, rigid part 2 and 3 were added as a complete PDB file. In spite of the distance between two rigid parts kept, there are not any conformational changes in the second structure of these rigid parts. To survey this event more accurately, the restraints that indicate salt bridge on rigid parts 2 and 3 were ignored. Then rigid parts 2 and 3 were added continually and docking was done in the same state. The new docking results display that their distance was about 4 Å, but instead of the formation of inverted V structure, L shape was occurred (Figure 5).
These results indicated the importance of salt bridges to form an inverted V shape of IR. On the other hand, we suggest that the force which created space between rigid parts 2 and 3 during the application of salt bridges causes to pull rigid part 3 to extracellular regions and to transfer a force to intracellular parts; therefore these processes activate intracellular signaling pathways.
With regard to the importance of α-CT, at first we focused on α-CT role in the complex of IR/insulin homodimer. Residue His710 contains a resonance structure and structural stability relative to the around residues, i.e., Asp707-Tyr708, 714, so His710 has the lowest affinity to create bond and also to participate in the interaction; the amount of affinity became too low especially in the presence of insulin.
To elucidate secondary structure changes, stride server was used. In our results, turn and random coil were converted to extended beta sheet which took place in the most parts of the complex of insulin and IR dimer (Figure 6).
Determination of hinge regions of insulin receptor by HingeProt. The combinations of both modes were used to build a complex of insulin and its receptor
Mode 1 | ||||
---|---|---|---|---|
Rigid Part No | Residues | Score | ||
Hinge residues: 254E, 598E, 655E, 710E | ||||
1 | E: 4-457 | 0.98 | ||
2 | E: 458-655, 693-710, 755-909 | 0.97 | ||
Hinge residues: 457E | ||||
Mode 2 | ||||
Rigid Part No | Residues | Score | ||
1 | E: 4-254 | 0.98 | ||
2 | E: 255-598 | 0.96 | ||
3 | E: 599-655, 755-909 | 0.96 | ||
4 | E: 693-710 | 0.78 |
List of generated salt bridges between insulin and rigid parts 2 &3. R554 & K484 (F1), D707 ( -CT) with residues 17 and 50 of insulin
Interaction sites | Distance (Å) |
---|---|
D17-R554 | 2.68 |
D17-K484 | 2.64 |
K50-D707 | 2.62 |
List of salt bridges between rigid parts 2 & 3. D499 (F1), R345 (F1), K166 (L1), R371 & D535 (F1), D645 (F2).
Interaction sites | Distance (Å) |
---|---|
D499-R371 | 2.73 |
R345-D535 | 2.59 |
K166-D645 | 2.66 |
Comparison of the experimental data between insulin and its receptor interaction sites with docking results of sequential method
Sequential docking methods | ||||||
---|---|---|---|---|---|---|
Name | segment ID | experimental Active residue | number of interaction of HADDOCK predicted active site & experiment | the number of new predicted active site by HADDOCK | ||
Resid | number | |||||
Rigid part 1 (1th monomer) | A | 32,34,36,37,39,65,62,64,88,89,94,96,120,124,153,154,247,246,248,254 | 20 | 17 | 85% | 5 |
Rigid parts 2,3 (1th monomer) | K | 454,394,780,804,282,256,25,255 | 8 | 7 | 88% | 34 |
Rigid part 4 (1th monomer) | J | 705,708,709,712,713 | 5 | 5 | 100% | 8 |
Insulin | D | 28,29,30,31,32,33,34,35,36,37,38,39,40,41,42,1,2,3,4,19,12,13,14,17 | 24 | 16 | 66% | 11 |
Rigid part 1 (2nd monomer) | I | 247,246,248,254 | 4 | 4 | 100% | 10 |
Rigid parts 2,3 (2nd monomer) | E | 484,552,591,602,616,620,62,780,804 | 9 | 6 | 66% | 12 |
The results of HADDOCK by using sequential docking method. Sequential docking method includes step 1: insulin & L1 domain (first monomer), step 2: output file of step1 & α-CT (second monomer), step 3: output file of step2 & FnIII-1 and FnIII-2 (second monomer), step 4: output file of step 3 & L1 domain (second monomer), step 5: output file of step 6 & α-CT (first monomer), step 6: output file of step 5 & FnIII-1 and FnIII-2 (first monomer).
Sequential docking method | ||||||
---|---|---|---|---|---|---|
Step 1 | Step 2 | Step 3 | Step 4 | Step 5 | Step 6 | |
HADDOCK score | -103.7+/-2.7 | -119.8+/-1.9 | -73.5+/-1.4 | -57.0+/-2.2 | -108.3+/-8.2 | -231.6+/-7.0 |
Cluster size | 64 | 129 | 198 | 200 | 191 | 200 |
RMSD* | 0.8+/-0.5 | 0.4+/-0.2 | 0.4+/-0.3 | 0.3+/-0.2 | 0.6+/-0.5 | 0.5+/-0.3 |
VDW energy | -47.9+/-6.6 | -75.8+/-5.2 | -58.4+/-5.3 | -30.8+/-1.1 | -55.4+/-4.8 | -114.4+/-12.7 |
Electrostatic energy | -142.4+/-25.5 | -142.1+/-61.3 | -323.2+/-20.0 | -83.2+/-30.4 | -200.0+/-29 | -1003.7+/-12.1 |
Desolvation energy | -39.1+/-6.0 | -36.1+/-11.1 | 1.2+/-4.0 | -10.2+/-5.2 | -24.7+/-10 | 74.2+/-14.9 |
Restraints violation energy | 117.7+/-46.85 | 205.9+/-54.56 | 482.7+/-3.36 | 5.9+/-0.17 | 119.0+/-7.77 | 93.6+/-24.43 |
Buried Surface Area | 1541.3+/-42.8 | 2028.6+/-62.6 | 2075.4+/-98.3 | 861.1+/-36.9 | 1652.0+/-59.3 | 5280.1+/-161 |
Z-Score | -1.3 | -2.5 | 0.0 | 0.0 | -1.0 | 0.0 |
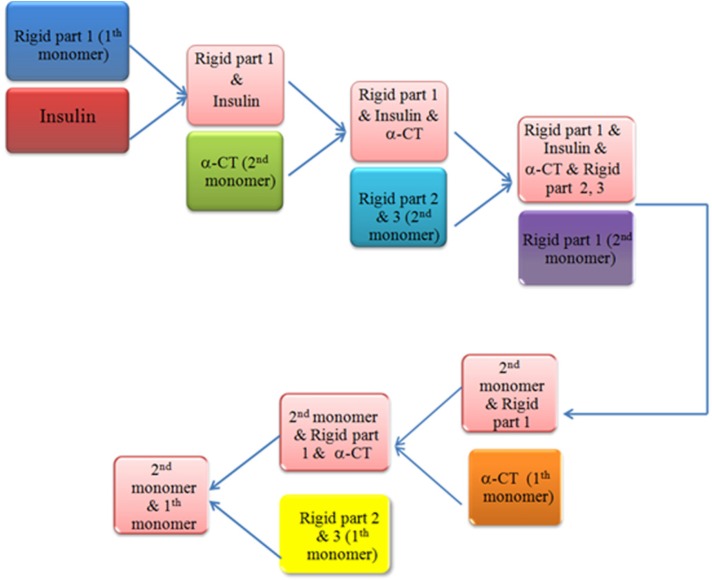
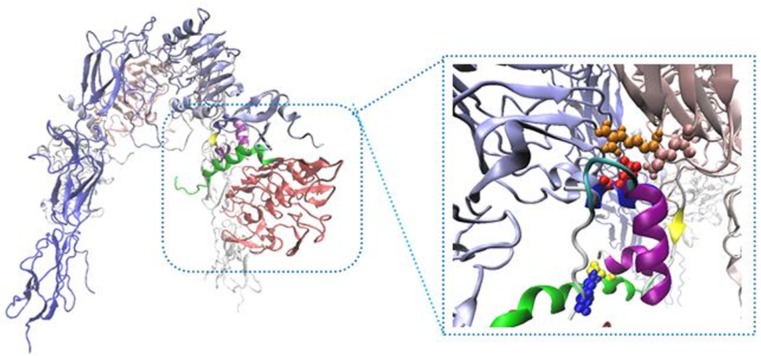
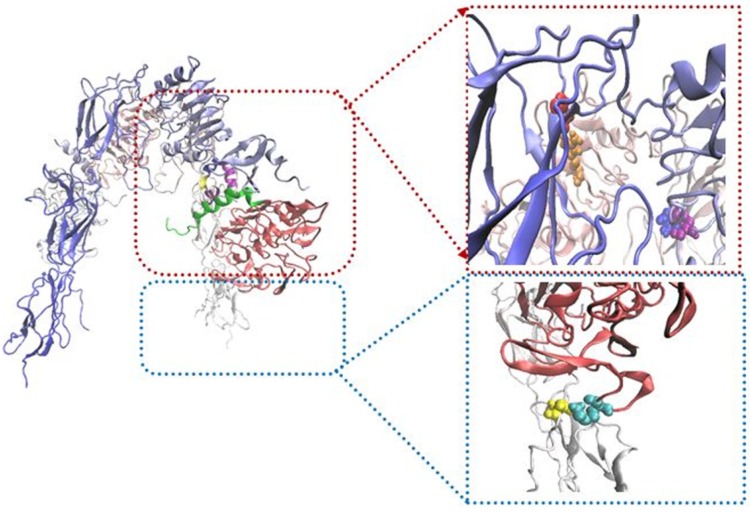
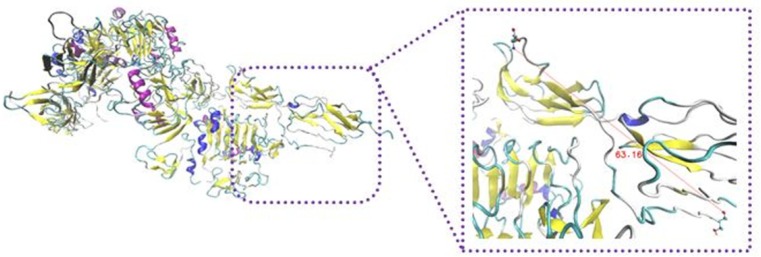
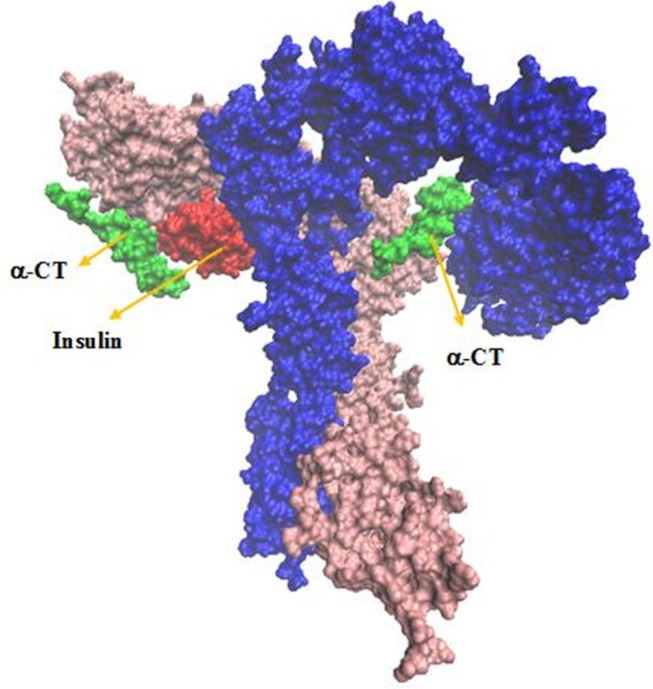
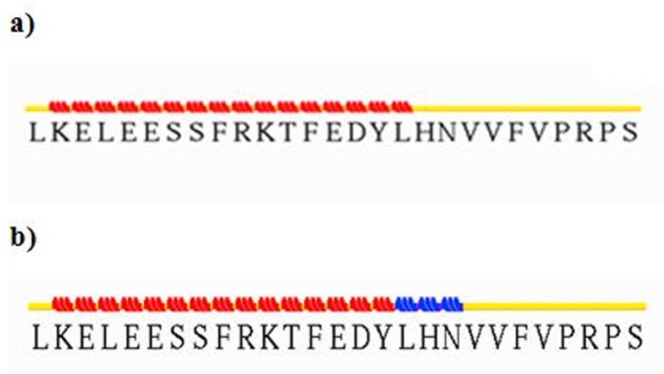
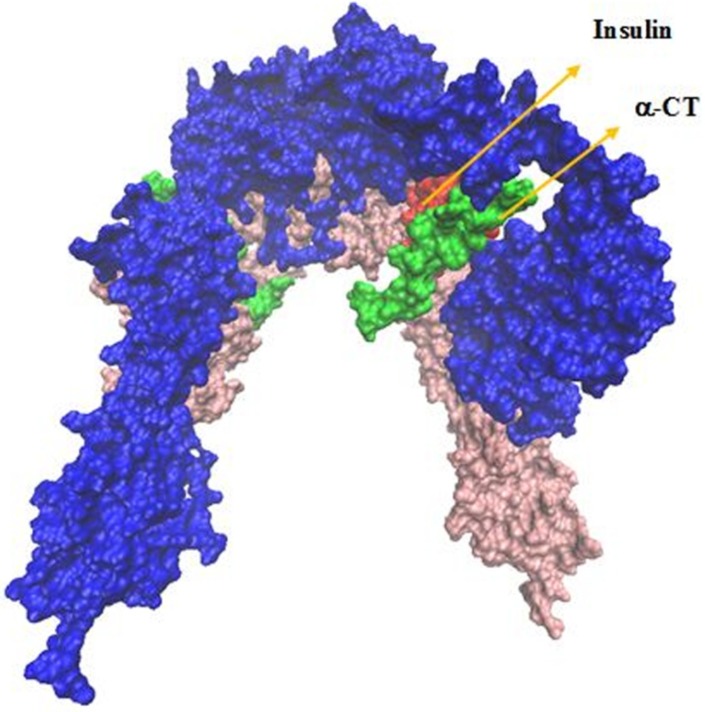
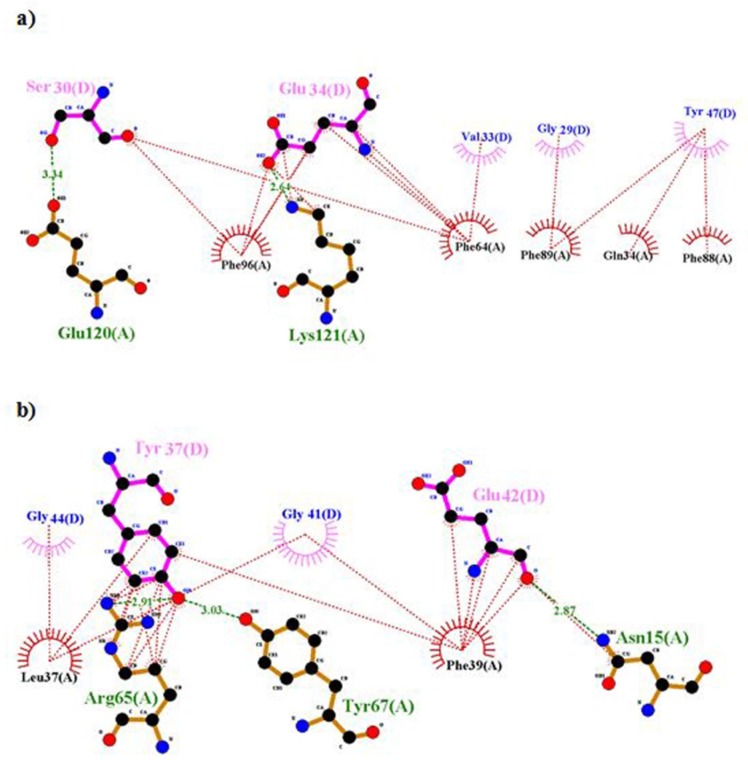
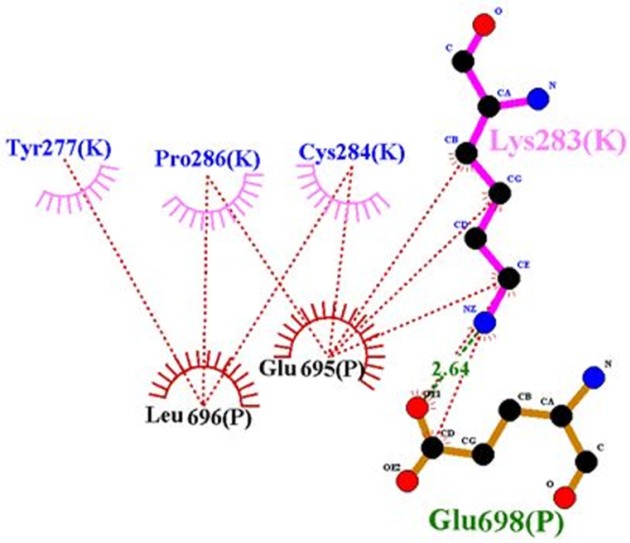
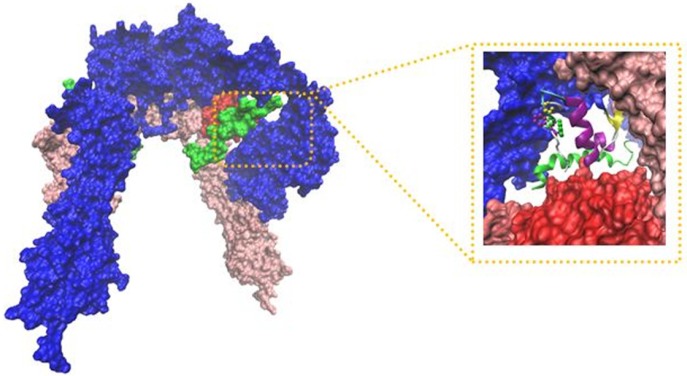
The extended beta sheet of secondary structures of insulin/IR complex is due to the formation of hydrogen bonds between protein atoms. Intracellular hydrogen bonds lead to be more stability in the protein structure (28). Significant hydrogen bonds were created between different regions of IR and insulin. Rigid parts 2 and 3 domains of the second monomer have the major contribution in the hydrogen bond and hydrophobic interactions. Because of closing rigid part 3 to L1 domain of the first monomer and generation hydrophobic interaction and salt bridges between them, both regions contain notable hydrogen and hydrophobic interactions. Our data indicated that the secondary structure of protein became looser than turn and coil shape.
To survey the conformational change in residues Asn711-Val715 of α-CT, docking results were analyzed by Ligplot and stride server. Because of residues 711-715 are hydrophobic amino acids such as Val, Phe, and Asn, so when these residues interacted with hydrophilic residues of insulin like Tyr, Arg, Glu, and His, it causes to lie α-CT in a suitable position relative to insulin and they interact each other appropriately.
To evaluate the accuracy of constructed model, the resulted structure was compared with active sites obtained from Lawrence’s articles (8, 12) (Table 4). Comparing Tables 4 with experimental results show that sequential docking method is a suitable approach to survey the interaction between two molecules because we applied additional information during the docking. This information included the order of components participation in docking processes to create a complex of insulin/IR dimer.
Our analysis in sequential docking showed input active sites of rigid part 4 of the first monomer participated in the interaction completely. Rigid part 4 of the second monomer interacted with insulin; rigid parts 1, 2 and 3 of the first monomer were compatible more than 80% with input data. Insulin was consistent with experimental data more than 60%. Illustration schematic of insulin/IR dimer by using sequential method has been represented in Figure 7.
The origin of the difference between our model and experimental data are discussed in the following:
According to Lawrence’s articles (8), hydrophobic face of α-CT comprising Phe705, Tyr708, Leu709, Val712 and Val713 engages a non-polar groove on L1β2 formed by Leu36, Leu37, Leu62, Phe64, Phe88, Phe89, Val94, and Phe96 (8). But in our model, some of them, i.e., residues 36, 62 and 94 were not involved in the interaction. There are hydrophobic interactions and hydrogen bonds between residues of rigid part 1 such as 34, 37, 39, 65, 64, 96, 89, and 88 with insulin, so these residues have an affinity for insulin and they cannot interact with -CT (Figure 8). But when residues of insulin, i.e., 30, 33, 34, 37, and 38 created hydrophobic interaction and hydrogen bond with rigid part 1, these residues prevented their neighbors, i.e., 31, 32, 35, 36, 39, and 40 to interact with rigid part 1. The same events happened for residue Asn282 in rigid part 3 of the first monomer. Residue 282 interacted with L1 of the first monomer (8), but in our model, hydrophobic interaction and hydrogen bond between its around residues, i.e., 284, 283, and 286 with rigid part 3 of the second monomer prohibited the presence of residue 282 in the suitable interaction (Figure 9).
In the experimental studies, situation and structural change of residues Gly44, Phe45, and Phe46 are unsolved (8), but our results showed the interaction between residues 44-37, 45-14, 45-37, and 46-14, it makes to lie the mentioned residues of insulin in the outside surface of L1-β2, CR and α-CT (Figure 10).
All output files resulting from HADDOCK have been shown in Table 5. According to the results of this table, the number of non-polar (hydrophobic) and charge amino acids interacted with each other in the step 4 are more than its previous and next phases in the sequential docking method. So this step has the most electrostatic and VDW energy and also the lowest buried surface area relative to the whole processes. Since step 4 of sequential docking method has the most negative VDW and electrostatic energy, so we can conclude the interaction sites of this stage is stronger than other phases. Based on this result, previous steps involve in the initiation of interaction between insulin and its receptor, but step 4 leads to make a tight contact due to the highest electrostatic and VDW energy.
Conclusions
We constructed a complex of insulin receptor dimer and insulin based on sequential docking method for which HADDOCK is used as docking platform. We present evidence about a significant role of three salt bridges being involved in the formation of a complex of insulin and its receptor. As a consequence, the role of salt bridges is actually necessary to form an insulin/ IR complex and an inverted V structure to initiate intracellular signaling cascades. In addition to presence salt bridges, α-CT plays an importance role to interact with insulin and also new insulin/IR contacts, particularly at site 2 were foretokened to lie α-CT in a suitable situation relative to insulin and these residues interact with each other, appropriately. These results have been made in the elucidation of the structure-function relationship of insulin and insulin receptor binding, as well as providing a suitable model of the ligand-receptor complex and valuable information which can be applied to design several drugs. Since this part of IR plays a vital role to bind to insulin, so novel anti-diabetic peptides and even small molecules can be designed to improve the affinity binding of α-CT relative to insulin.
Acknowledgements
References
-
1.
Baynes JW, Thorpe SR. The role of oxidative stress in diabetic complications. Curr. Opin. Endocrinol. 1996;3:277-84.
-
2.
Arulselvan P, Subramanian SP. Beneficial effects of Murraya koenigii leaves on antioxi dant defense system and ultra structural changes of pancreatic β-cells in experimental diabetes in rats. Chem-Biol. Interact. 2007;165:155-64. [PubMed ID: 17188670].
-
3.
Saltiel AR, Pessin JE. Insulin signaling pathways in time and space. Trends Cell Biol. 2002;12:65-71. [PubMed ID: 11849969].
-
4.
Menting JG, Yang Y, Chan SJ, Phillips NB, Smith BJ, Whittaker J, Wickramasinghe NP, Whittaker LJ, Pandyarajan V, Wan ZL, Yadav SP, Carroll JM, Strokes N, Roberts CT Jr, Ismail-Beigi F, Milewski W, Steiner DF, Chauhan VS, Ward CW, Weiss MA, Lawrence MC. Protective hinge in insulin opens to enable its receptor engagement. P. Natl. A. Sci. 2014;111:E3395-E404.
-
5.
Nakamura T, Takahashi H, Takahashi M, Shimba N, Suzuki E, Shimada I. Direct Determination of the Insulin− Insulin Receptor Interface Using Transferred Cross-Saturation Experiments. J. Med. Chem. 2010;53:1917-22. [PubMed ID: 20158183].
-
6.
Guedes S, Vitorino R, Domingues MRM, Amado F, Domingues P. Mass spectrometry characterization of the glycation sites of bovine insulin by tandem mass spectrometry. J. Am. Soc. Mass. Spectr. 2009;20:1319-26.
-
7.
Li Q, Wong YL, Kang C. Solution structure of the transmembrane domain of the insulin receptor in detergent micelles. BBA-Biomembranes. 2014;1838:1313-21. [PubMed ID: 24440425].
-
8.
Menting JG, Whittaker J, Margetts MB, Whittaker LJ, Kong GK-W, Smith BJ, Christopher JW, Lenka Ž, Emília K, Jiří J, Shu JC, Donald FS, Guy GD, Andrzej MB, Michael AW, Colin WW, Michael CL. How insulin engages its primary binding site on the insulin receptor. Nature. 2013;493:241-5. [PubMed ID: 23302862].
-
9.
Smith BJ, Huang K, Kong G, Chan SJ, Nakagawa S, Menting JG, Hu SQ, Whittaker J, Steiner DF, Katsoyannis PG, Ward CW, Weiss MA, Lawrence MC. Structural resolution of a tandem hormone-binding element in the insulin receptor and its implications for design of peptide agonists. P. Natl. A. Sci. 2010;107:6771-6.
-
10.
Ward CW, Menting JG, Lawrence MC. The insulin receptor changes conformation in unforeseen ways on ligand binding: sharpening the picture of insulin receptor activation. Bioessays. 2013;35:945-54. [PubMed ID: 24037759].
-
11.
Ward CW, Lawrence MC. Similar but different: ligand-induced activation of the insulin and epidermal growth factor receptor families. Curr. Opin. Struc. Biol. 2012;22:360-6.
-
12.
Lawrence MC, McKern NM, Ward CW. Insulin receptor structure and its implications for the IGF-1 receptor. Curr. Opin. Struc. Biol. 2007;17:699-705.
-
13.
Laskowski RA, MacArthur MW, Moss DS, Thornton JM. PROCHECK: a program to check the stereochemical quality of protein structures. J. Appl. Crystallogr. 1993;26:283-91.
-
14.
Benkert P, Künzli M, Schwede T. QMEAN server for protein model quality estimation. Nucleic. Acids. Res. 2009:322. [PubMed ID: 19042971].
-
15.
Sippl MJ. Recognition of errors in three-dimensional structures of proteins. Proteins. 1993;17:355-62. [PubMed ID: 8108378].
-
16.
Haliloglu T, Bahar I, Erman B. Gaussian dynamics of folded proteins. Phys. Rev. Lett. 1997;79:3090.
-
17.
Eyal E, Yang L-W, Bahar I. Anisotropic network model: systematic evaluation and a new web interface. Bioinformatics. 2006;22:2619-27. [PubMed ID: 16928735].
-
18.
Emekli U, Schneidman-Duhovny D, Wolfson HJ, Nussinov R, Haliloglu T. HingeProt: automated prediction of hinges in protein structures. Proteins. 2008;70:1219-27. [PubMed ID: 17847101].
-
19.
Dominguez C, Boelens R, Bonvin AM. HADDOCK: a protein-protein docking approach based on biochemical or biophysical information. J. Am. Chem. Soc. 2003;125:1731-7. [PubMed ID: 12580598].
-
20.
De Vries SJ, van Dijk M, Bonvin AM. The HADDOCK web server for data-driven biomolecular docking. Nat. Protoc. 2010;5:883-97. [PubMed ID: 20431534].
-
21.
Karaca E, Bonvin AM. A multidomain flexible docking approach to deal with large conformational changes in the modeling of biomolecular complexes. Structure. 2011;19:555-65. [PubMed ID: 21481778].
-
22.
Lawrence MC, McKern NM, Ward CW. Insulin receptor structure and its implications for the IGF-1 receptor. Curr. Opin. Struct. Biol. 2007;17:699-705. [PubMed ID: 17851071].
-
23.
McKern NM, Lawrence MC, Streltsov VA, Lou M-Z, Adams TE, Lovrecz GO, Elleman TC, Richards KM, Bentley JD, Pilling PA, Hoyne PA, Cartledge KA, Pham TM, Lewis JL, Sankovich SE, Stoichevska V, Da Silva E, Robinson CP, Frenkel MJ, Sparrow LG, Fernley RT, Epa VC, Ward CW. Structure of the insulin receptor ectodomain reveals a folded-over conformation. Nature. 2006;443:218-21. [PubMed ID: 16957736].
-
24.
De Vries SJ, van Dijk AD, Krzeminski M, van Dijk M, Thureau A, Hsu V, Wassenaar T, Bonvin A. HADDOCK versus HADDOCK: new features and performance of HADDOCK2 0 on the CAPRI targets. Proteins. 2007;69:726-33. [PubMed ID: 17803234].
-
25.
Wallace AC, Laskowski RA, Thornton JM. LIGPLOT: a program to generate schematic diagrams of protein-ligand interactions. Protein. Eng. 1995;8:127-34. [PubMed ID: 7630882].
-
26.
Heinig M, Frishman D. STRIDE: a web server for secondary structure assignment from known atomic coordinates of proteins. Nucleic Acids Res. 2004;32:W500-W2. [PubMed ID: 15215436].
-
27.
Humphrey W, Dalke A, Schulten K. VMD: visual molecular dynamics. J. Mol. Graphics. 1996:14.
-
28.
Scheiner S. Contributions of NH O and CH O Hydrogen Bonds to the Stability of β-Sheets in Proteins. J. Phys. Chem. B. 2006;110:18670-9. [PubMed ID: 16970498].