Abstract
Keywords
β-Glucosidase Biotransformation Genistein Response surface methodology Antioxidant
Introduction
Among the foods eaten by humans, soybeans contain the highest level of isoflavones, which are phytoestrogen (plant-derived phenolic compounds with structural homology to human estrogen), common in leguminous plants (1). These compounds are currently heralded to offer potential natural alternative therapies for a range of hormone-dependent conditions including menopausal symptoms (2), cardiovascular disease (3), osteoporosis (4) as well as prostate, breast, and colon cancer (5).
Soy isoflavones exist in the form of aglycones (daidzein, genistein and glycitein) and β-glycosides conjugates, which include the glycosides (daidzin, genistin and glycitin), malonylglycosides and acetylglycosides. The content of daidzin and genistin are high in soybeans while the aglycones (daidzein, genistein) are found in trace quantities (6).
Numerous studies have shown that the biological effects of isoflavones are not due to the glycoside forms but mainly to their aglycones. For example, aglycone is able to bind to estrogen receptor and hence mimic estradiol functions in the human body and thus prevent certain cancers (7). The anti-cancer function of soybean isoflavones was shown to be associated with genistein, which inhibits protein tyrosine kinases and DNA topoisomerase, and binds weakly to estrogen receptors (8). It was found that early exposure to genistein enhances cell differentiation of the mammary gland, and may confer a protective effect against carcinogenesis via this process (9). Furthermore, in vitro studies using cultured human breast cancer cells indicate that genistein inhibited the growth of both estrogen receptor-negative and estrogen receptor-positive cell lines (10). Also, genistein exhibited a much greater antioxidant activity than that of genistin (11).
Enzymatic transformation has been known to have several advantages over chemical methods in the transformation process. These advantages include the mild reaction conditions, the high yield of hydrolysis and the reduction of the inhibitory compounds formed (12). For this reasons, we turn our think to the use of β-glucosidase (EC 3.2.1.21, β-glucoside glucohydrolase), which mainly catalyzes hydrolysis of the β-1,4-glycosidic linkage in various disaccharides, oligosaccharides, as well as alkyl- and aryl-β-D-glucosides (13). The current work is the first time to use response surface methodology in the optimization of the biotransformation condition of the major soy isoflavone (genestin) into its aglycone (genestein) in the soy flour extract by commercial β-gluosidase containing enzyme (celluclast BG) and to evaluate the effect of this biotransfomation on the antioxidant activity.
Experimental
Plant material
Defatted soy flour was obtained from the Agricultural Research Center (ARC), Giza, Egypt.
Authentic
Genistein standard and DPPH were purchased from sigma. Authentic sugars; arabinose, galactose, glucose, mannose, rhamnose, and xylose were obtained from E. Merck, Darmstadt, Germany. Cellobiose was obtained from Cambrian chemical. β-Glucosidase containing enzyme (celluclast BG) was obtained from Novozymes.
Isolation of soy isoflavone glycosides
Materials for chromatographic study
Pre-coated TLC plates silica gel 60 F254 (20 × 20 cm, 0.25 mm thickness) and silica gel H type 60 were obtained from E. Merck, Darmstadt, Germany. Sephadex LH-20, for column chromatography (CC) was obtained from Pharmacia Fine Chemicals AB.
Extraction, fractionation and isolation of soy isoflavones
Defatted soy flour (2.5 kg) was extracted with 70% methanol (3 L × 3) on cold till exhaustion. The extract was evaporated under vacuum at 60 °C to remove the methanol. The remaining aqueous extract was lyophilized to give 37 g. Fifteen g of the lyophilized residue was subjected to vacuum liquid chromatography (15 × 5 cm) packed with silica gel-H (55 g). Gradient elution was carried out starting with hexane: chloroform (1:1), the polarity was increased by 10% stepwise addition of chloroform till 100% chloroform followed by 10% stepwise addition of ethyl acetate till 100% ethyl acetate then 5% stepwise addition of methanol till 100% methanol, to obtain 38 fractions. The obtained fractions were monitored by TLC, similar fractions were collected together, and the solvent was evaporated under reduced pressure. Fractions containing major spots were subjected to further fractionation.
Compound 1 (20 mg, yellowish white needle crystals) and compound 2 (10 mg, yellowish white crystals) were obtained upon re-chromatography of fractions (22-27) and (28-38), respectively, on sephadex (1 × 20 cm) using methanol for elution.
Spectral analysis of the isolated compounds
1NMR analysis was measured on 1H-NMR (300 MHz): Varian Mercury-VX-300 spectrophotometer.
Acid hydrolysis of the isolated glycosides
The two isolated glycosides (2 mg) were hydrolyzed following the method of Harborne et al. 1975 (14). The obtained aglycones and sugars were identified by comparison with authentic using TLC.
Assay of the β-glucosidase enzyme
Cellobiose substrate (0.5 mL of 0.4%) in 0.05 M citrate phosphate buffer (pH 4.8) was added to 0.5 mL of celluclast BG enzyme dissolved in 0.5 mL of 0.05 M citrate phosphate buffer (pH 4.8). Then they were incubated for 30 min at 50 °C. The reaction mixture was placed in a boiling water for 5 min to stop the reaction and then immediately cooled in an ice bath. This mixture assayed by a glucose oxidase kit to determine glucose concentration. One unit of β-glucosidase activity was defined as the amount of enzyme that produced 1 µmol of glucose per min from cellobiose (15).
Factors and testing levels for Plackett-Burman experiment for deglycosylation of genistin by the commercial β-glucosidase enzyme.
Factor | Symbol | Low level (-1) | High level (+1) |
---|---|---|---|
Ph | X1 | 4 | 7 |
Temp. (°C) | X2 | 40 | 60 |
Time (h) | X3 | 0.5 | 3 |
Substrate conc. (w/v) | X4 | 0.05 | 0.1 |
Enzyme conc. (IU/mL) | X5 | 0.2 | 0.5 |
Agitation (rpm) | X6 | 100 | 200 |
Buffer strength (M) | X7 | 0.05 | 0.1 |
CaCl2 (mM) | X8 | 0.5 | 1 |
MnCl2 (mM) | X9 | 0.5 | 1 |
Randomized Plakett-Burman experimental design for evaluating factors influencing deglycosylation of genistin by the commercial β-glucosidase containing enzyme.
pH | Temp. | Time (h) | Substrate conc. (mg/mL) | Enzyme conc. (IU) | Agitation (rpm) | Buffer strength (M) | CaCl2 mM | MnCl2 mM | Genistein conc. | |
---|---|---|---|---|---|---|---|---|---|---|
1 | 7 | 40 | 0.5 | 1 | 0.5 | 100 | 0.05 | 1 | 0.5 | 1.245 |
2 | 4 | 60 | 0.5 | 1 | 0.5 | 200 | 0.05 | 0.5 | 1 | 2.574 |
3 | 7 | 60 | 3 | 1 | 0.2 | 100 | 0.05 | 0.5 | 1 | 1.734 |
4 | 4 | 40 | 0.5 | 0.5 | 0.2 | 100 | 0.1 | 0.5 | 1 | 1.1 |
5 | 4 | 60 | 3 | 0.5 | 0.2 | 100 | 0.05 | 1 | 0.5 | 2.68 |
6 | 7 | 40 | 3 | 1 | 0.2 | 200 | 0.1 | 1 | 1 | 2.142 |
7 | 7 | 40 | 0.5 | 0.5 | 0.2 | 200 | 0.05 | 0.5 | 0.5 | 0.633 |
8 | 4 | 60 | 0.5 | 1 | 0.2 | 200 | 0.1 | 1 | 0.5 | 2.028 |
9 | 4 | 40 | 3 | 1 | 0.5 | 100 | 0.1 | 0.5 | 0.5 | 4.05 |
10 | 7 | 60 | 0.5 | 0.5 | 0.5 | 100 | 0.1 | 1 | 1 | 1.044 |
11 | 4 | 40 | 3 | 0.5 | 0.5 | 200 | 0.05 | 1 | 1 | 4.167 |
12 | 7 | 60 | 3 | 0.5 | 0.5 | 200 | 0.1 | 0.5 | 0.5 | 3.378 |
Sorted parameter estimates the effect of each variable on genistin deglycosylation by commercial β-glucosidase enzyme using Placket-Burman design
Box-Behnken factorial experimental design, representing response of genistin deglycosylation by commercial β-glucosidase enzyme as influenced by enzyme conc., reaction time, agitation rate and pH.
Pattern | Enzyme conc. | Agitation rate | Time (h) | pH | Genistein | |
---|---|---|---|---|---|---|
25 | 00-- | 0.75 | 200 | 3 | 4 | 3.73 |
1 | 0+0+ | 1 | 250 | 5 | 4 | 7.93 |
2 | +0+0 | 0.75 | 300 | 4 | 5 | |
3 | -0+0 | 0.75 | 300 | 4 | 3 | |
4 | 0000 | 0.75 | 250 | 4 | 4 | 4.73 |
5 | --00 | 0.5 | 250 | 4 | 3 | 4.299 |
6 | -00+ | 0.75 | 250 | 5 | 3 | 7.016 |
7 | 00+- | 0.75 | 300 | 3 | 4 | 2.383 |
8 | -+00 | 1 | 250 | 4 | 3 | 6.64 |
9 | +0-0 | 0.75 | 200 | 4 | 5 | 5.66 |
10 | -0-0 | 0.75 | 200 | 4 | 3 | 4.483 |
11 | 0—0 | 0.5 | 200 | 4 | 4 | 4.5 |
12 | ++00 | 1 | 250 | 4 | 5 | 5.45 |
13 | 00++ | 0.75 | 300 | 5 | 4 | |
14 | +00+ | 0.75 | 250 | 5 | 5 | |
15 | 0+0- | 1 | 250 | 3 | 4 | 3.58 |
16 | +00- | 0.75 | 250 | 3 | 5 | 2.92 |
17 | -00- | 0.75 | 250 | 3 | 3 | 3.528 |
18 | 0-0+ | 0.5 | 250 | 5 | 4 | |
19 | 0++0 | 1 | 300 | 4 | 4 | 5.908 |
20 | 0-+0 | 0.5 | 300 | 4 | 4 | 3.946 |
21 | 0+-0 | 1 | 200 | 4 | 4 | |
22 | +-00 | 0.5 | 250 | 4 | 5 | 3.348 |
23 | 00-- | 0.75 | 200 | 5 | 4 | 6 |
24 | 0-0- | 0.5 | 250 | 3 | 4 | 2.182 |
Sorted parameter estimates the effect of each variable on genistin deglycosylation by commercial β-glucosidase enzyme using Box-Behnken design.
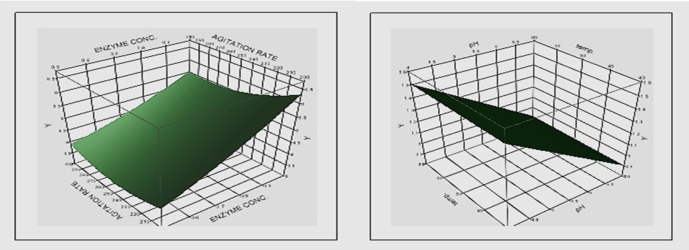
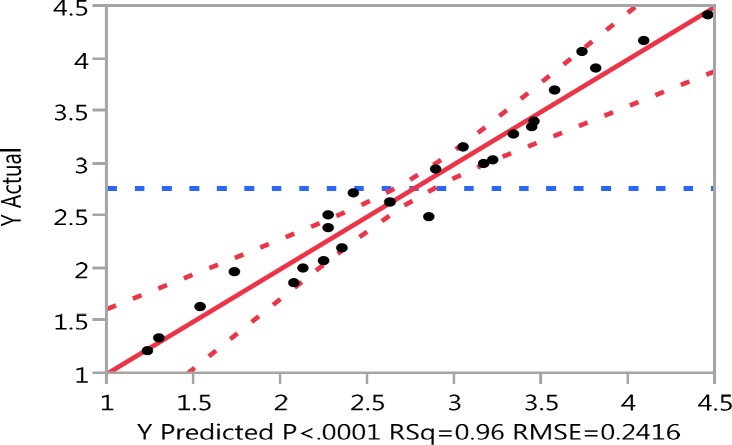
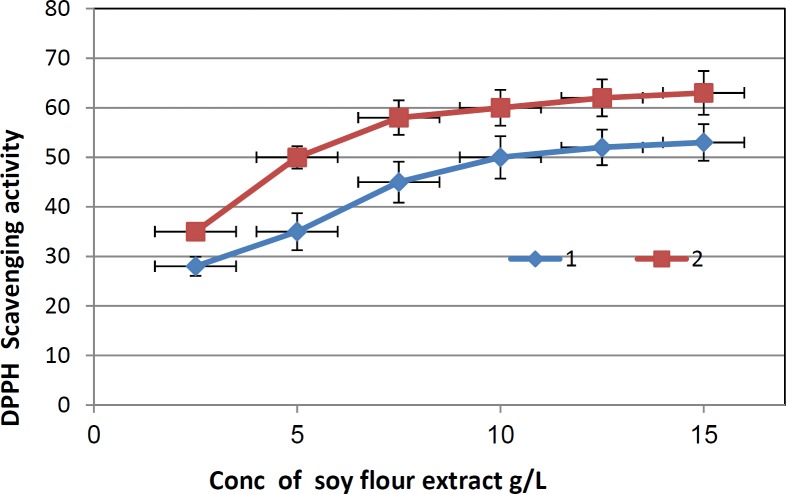
Enzymatic hydrolysis of soy flour
Celluclast BG enzyme in citrate phosphate buffer (0.5 mL) was incubated with 0.5 mL of CaCl2 and 0.5 mL of MnCl2 at room temperature for 60 min. Then, 0.5 mL of this solution was incubated with 0.5 mL of defatted soy flour (substrate) suspended in citrate phosphate buffer under the conditions indicated in the experimental design (Tables 2 and 4). The reactions were terminated by boiling the suspension for 5 min, and then the hydrolyzed solutions were centrifuged at 11,000 rpm for 15 min to collect supernatants containing isoflavones.
HPLC determination of genistein
The genistein content was determined by Shimadzu HPLC system (Kyoto, Japan). Chromatographic separation was carried out on Dikma Diamonsil C18 column (4.6-250 mm Dima Co., Ltd., Orlando, FL). The mobile phase had the following composition: 0.1% (v/v) acetic acid in filtered MilliQ water (solvent A), and 0.1% (v/v) acetic acid in acetonitrile (solvent B). The following gradients for solvent B was applied: 15–25% over 35 min, 25–26.5% over 12 min, and 26.5–50% over 30 sec, followed by isocratic elution for 14.5 min. with a flow rate of 1.0 mL/min and column temperature setting at 40 °C and absorbance was measured at 254 nm (16).
Optimization of genistein production based on multifactorial experiments
Evaluation of the factors affecting genistein production
Nine assigned variables (pH, reaction time, temperature, substrate conc., enzyme conc., agitation rate, CaCl2, MnCl2 and buffer strength) were screened in twelve experimental trials using Plackett-Burman experimental design (17). Table 1 illustrates the examined factors, as well as the levels of each factor used in the experimental design, whereas Table 2 represents the design matrix. The magnitude and the ranking of each variable in Plackett-Burman design were estimated by statistical analyses of the data. The main effect of each variable was calculated as the difference between the average of measurements made at the high value (+) and at the low value (-) (Table 3). All experiments were carried out in triplicate and the concentration of genistein aglycone (mg/g) was taken as the response (dependent variable).
Optimization of the factors affecting enzymatic deglycosylation of genistin
The variables with significant effects on enzyme production, as identified by the Plackett–Burman design were further optimized using a response surface Box–Behnken design (18). The design comprised 25 experiments where pH, reaction time, enzyme conc. and agitation rate were tested at three levels and in multiple combinations with the other parameters (Table 4). The effect of each variable on genistin deglycosylation was estimated by statistical method and the results were given in Table 5 and illustrated in Figure 1. The whole set of experiments was performed in triplicate and the mean response was used for analyses.
Statistical analysis
The optimal value for production of soy isoflavone aglycone was estimated using SAS JMP 8 NULL program tools for a regression analysis of the obtained experimental data. The quality of the fit of the polynomial model was expressed by the coefficient of determination “RSq”. The experiments were performed in triplicate and the mean values were given.
Verification of the model
The optimal conditions realized from the optimization experiment were verified experimentally and compared with the data calculated from the model (Figure 2). The determination coefficient (RSq) was 0.96.
Antioxidant assay
Antioxidant activity of soy flour methanolic extract was determined by the ability to scavenge 2,2-diphenyl-1-picryl-hydrazyl (DPPH) radicals. One mL DPPH (0.1 mM) solution in methanol was mixed with 100 µL of the crude soy extract, vortexed well, and then incubated for 30 min in the dark at room temperature and the absorbance was measured at 517 nm. For the control sample, the crude soy extract was replaced with 100 µL methanol. This antioxidant activity was given as percent (%) DPPH scavenging and calculated as [(control absorbance - extract absorbance)/(control absorbance) × 100].
Percentage of the DPPH scavenging activity was plotted versus the different concentrations of soy flour extracts used (Figure 3) and the concentration causing a 50% reduction of DPPH absorbance was taken as the half maximal inhibitory concentration (EC50) value. EC50 values were obtained by extrapolation of the data and calculated on the basis of the total solid contents of each methanolic extract (19).
Results and Discussion
Identification of the isolated compounds
To confirm the presence of genistin in soy flour, it was subjected to chromatographic fractionation resulted in isolation of two compounds (1 and 2). By comparing 1HNMR spectral data of compounds 1 and 2 with the previously reported data (20), as well as by acid hydrolysis and comparison with authentic aglycones and sugars using TLC, these compounds were identified as genistin and daidzin, respectively.
Compound 1: Rf = 0.40 in ethyl acetate: MeOH: H2O: Formic acid (100: 16: 13: 20 drops), showed dull fluorescence in UV, no color with p-anisaldehyde spray reagent. 1H-NMR (CD3OD): δH 8.15 (1H, s, H-2), 7.36 (2H, d, J=7.6 Hz, H-2′ and H-6′), 6.86 (2H, d, J=7.6 Hz, H-3′ and H-5′). 6.71 (1H, d, J=2.1 Hz, H-8), 6.51 (1H, d, J=2.1 Hz, H-6), 5.19 (1H, d, J=7.6 Hz, H-1′′), 3.43-4.12 (m, Sugar protons).
Compound 2: Rf = 0.45 in ethyl acetate: MeOH: H2O: Formic acid (100: 16: 13: 20 drops), showed dull fluorescence in UV, no color with p-anisaldehyde spray reagent. 1H-NMR (CD3OD): δH 8.208 (1H, s, H-2), 8.126 (1H, d, J=9.2 Hz, H-5), 7.358 (2H, d, J=8.4 Hz, H-2′ and H-6′), 7.223 (1H, dd, J=10.7, 1.5 Hz, H-6), 7.207 (1H, d, J=2.1 Hz, H-8), 6.846 (2H, d, J=8.4 Hz, H-3′ and H-5′), 5.029 (1H, d, J=7.6 Hz, H-1′′), 3.38-3.94 (m, Sugar protons).
Optimization of genistein production
A sequential optimization strategy was applied in this work, where the first phase dealt with screening and identifying the reaction mixture components and conditions affecting genistein aglycone production by the β-glucosidase enzymes. Once the significant factors were determined, the second phase involving ascertaining the combinations leading to the maximum genistein aglycone yield were carried out.
In the first phase, a Plackett-Burman experimental design was applied to reflect the relative importance of various enzymatic biotransformation factors. The data shown in Table 2 revealed a wide variation in genistein conc. (0.633-4.167 mg/g), thereby reflecting the significant effect of the studied factors for attaining a higher productivity. Sorted parameters estimates of the nine tested variables, revealing that enzyme conc., time and agitation rate had a significant positive effect on genistein aglycone production, whereas, pH had a significant negative effect (Table 3).
To improve the pre-optimization formula for the subsequent optimization step, the variables with a negative-effect value obtained from the Plackett-Burman design were fixed at their (-1) coded values, and the variables with a positive-effect value fixed at their (+1) coded value. To identify the optimum response region for genistein aglycone the significant independent variables [reaction time, agitation rate, enzyme concentration and pH] were further explored at three levels. Table 4 presented the design matrix for the variables, given in both coded and natural units, plus the experimental genistein aglycone production results.
Figure 1 shows the relationships between the significant tested factors (enzyme concentration, time, agitation rate and pH) and soy isoflavone aglycone (genistein) yield.
The results obtained (Table 5) revealed that the reaction time is the most effective factor on genistein concentration followed by the enzyme concentration. Increasing the reaction time and enzyme concentration were accompanied by increase in genistein concentration. On the other hand, increasing pH and agitation rate were followed by decrease in genistein concentration. In general, increasing the reaction time to 5 h increased genistein concentration unless the enzyme concentration was decreased to its lower limit (0.5 IU). It is noticed that increasing pH to its high limit (5) was accompanied by decrease in the genistein concentration at agitation rate of 250 and 300 rpm, meanwhile decreasing the agitation rate to its lower limit (200 rpm) reversed this effect at the same enzyme concentration (0.75 IU) and reaction time (4 h).
The optimal levels for the factors were found to be, enzyme concentration (1 IU), time (5 h), agitation rate (250 rpm) and pH (4), where genistein concentration reach up to (7.93 mg/g). On the other hand, the lower aglycone concentration (2.182 mg/g) was obtained at experimental conditions of: enzyme concentration (0.5 IU), time (3 h), agitation rate (250 rpm), and pH (4).
Also, it was found that, the concentration of genistein aglycones in the biotransformed suspension was increased by 9.91 folds compared to the non biotransformed one. Similar results were obtained where the content of daidzein had increased by 34 folds in soybean flour suspension biotransformed by a thermostable β-glucosidase enzyme produced from Paecilomyces thermophile (21). Maitan-Alfenas et al. 2014 (22) found that β-glucosidase increased genistein content from 1.28 mg/g in the non biotransformed to 6.37 mg/g in the biotransformed soy molasses. This result also supported by Pandijtan et al. 2000 (23) who found that β-glucosidase enzyme increased genistein content from 0.028 mg/g in the non biotransformed to 0.583 mg/g in the biotransformed soy protein concentrate.
On the model level, the correlation measures for estimating the regression equation were the determination coefficient (RSq). The closer the value of RSq to 1, the better the correlation between the measured and the predicted values. In this experiment, the value of the determination coefficient (RSq) was 0.96 indicating a high degree of correlation between the experimental and predicted values that confirms the high accuracy of the model and also suggestes that the models are satisfactory and accurate.
Antioxidant activity
Lately it has been reported that reactive oxygen species (ROS) are implicated in a large number of human diseases. When an imbalance between antioxidants and generation of ROS occurs, oxidative damage can occur and generate a large number of health problems such as arteriosclerosis and cancer (24). Therefore, there is increased interest focused on natural antioxidants present in foods and medicinal plants. In this study, the antioxidant properties of the non biotransformed and biotransformed soy flour extracts were evaluated by DPPH method. The two extracts showed a scavenging activity toward the DPPH radical in a dose-dependent manner (2.5-15 mg/mL). EC50 were 10 and 5 mg/mL for non biotransformed and biotransformed soy flour extracts, respectively. The increase in the antioxidant activity is explained by that the hydrolysis of β-glucosidic linkage leads to liberation of hydroxyl groups in free aglycone which might be important to the antioxidant activity of the extract (25).
Conclusion
The estimated optimal values for production of soy isoflavone aglycone were found to be enzyme concentration, (1 IU), time (5 h), agitation rate (250 rpm), and pH 4. The Biotransformation of soy flour by β-glucosidase enzyme using these optimal factors increased genistein concentration by 9.91 folds.
There was 2 fold increase in the antioxidant activities of the biotransformed soy flour suspension comparing to the non biotransformed one.
References
-
1.
Munro IC, Harwood M, Hlywka JJ, Stephen AM, Doull J, Flamm WG, Adlercreutz H. Soy isoflavones: A safety review. Nutr. Rev. 2003;61:1-33. [PubMed ID: 12638461].
-
2.
Cheng G, Wilczek B, Warner M, Gustafsson JA, Landgren BM. Isoflavone treatment for acute menopausal symptoms. Menopause. 2007;14:468-73. [PubMed ID: 17290160].
-
3.
Gil-Izquierdoa A, Peñalvob JL, Gilc JI, Medinaa S, Horcajadad MN, Lafay S, Silberberg M, Llorachh R, Zafrillai P, García-Moraa P, Ferreresa F. Soy isoflavones and cardiovascular disease epidemiological, clinical and -omics perspectives. Curr. Pharm. Biotechnol. 2012;13:624-31. [PubMed ID: 22122477].
-
4.
Taku K, Melbyb MK, Nishic N, Omorid T, Kurzere MS. Soy isoflavones for osteoporosis: An evidence-based approach. Maturitas. 2011;70:333-8. [PubMed ID: 21958941].
-
5.
Akaza H. Prostate cancer chemoprevention by soy isoflavones: Role of intestinal bacteria as the “second human genome”. Cancer Sci. 2012;103:969-75. [PubMed ID: 22372745].
-
6.
Yamabe S, Kobayashi-Hattori K, Kaneko K, Endo H, Takita T. Effect of soybean varieties on the content and composition of isoflavone in rice-koji miso. Food Chem. 2007;100:369-74.
-
7.
Sun YM, Zhang YZ, Liu N, Li DM, Li MZ. Hydrolysis of genistin and daidzin by a beta glucosidase purified from Lentinula edodes. J. Med. Plants Res. 2010;4:2684-90.
-
8.
Sunita P, Pattanayak SP. Phytoestrogens in postmenopausal indications: A theoretical perspective. Pharmacog. Rev. 2011;5:41-7.
-
9.
Lamartiniere CA. Protection against breast cancer with genistein: A component of soy Am. J. Clin. Nutr. 2000;71:1705-9.
-
10.
Mai Z, Blackburn GL, Zhou JR. Genistein sensitizes inhibitory effect of tamoxifen on the growth of estrogen receptor-positive and HER2-overexpressing human breast cancer cells. Mol. Carcinog. 2007;46:534-42. [PubMed ID: 17295235].
-
11.
Kroyer GT. Red clover extract as antioxidant active and functional food ingredient. Innov. Food Sci. Emerg. Technol. . 2004;5:101-5.
-
12.
Bornscheuer UT. Chemical biology: A radical change in enzyme catalysis. Nature. 2016;540:345-6. [PubMed ID: 27974776].
-
13.
Singh G, Verma AK, Kumar V. Catalytic properties, functional attributes and industrial applications of β-glucosidases. Biotech. 2016;6:1-14.
-
14.
Harborne JB, Mabry TJ, Mabry H, editors. The flavonoids. New York, San Francisco, Chapman and Hall, London: Acad. Press, Inc; 1975. 267 p.
-
15.
Zaldívar M, Velásquez JC, Contreras I, Pérez L. Trichoderma aureoviride 7-121, a mutant with enhanced production of lytic enzymes: Its potential use in waste cellulose degradation and/or biocontrol. Electron. J. Biotechnol. 2001;4:13-4.
-
16.
Wang C, Kurzer MS. Phytoestrogen concentration determines effects on DNA synthesis in human breast cancer cells. Nutr. Cancer. 1997;28:236-47. [PubMed ID: 9343831].
-
17.
Liu W, Yu Y, Yang R, Wan C, Xu B, Cao S. Optimization of total flavonoid compound extraction from Gynura medica leaf using response surface methodology and chemical composition analysis. Int. J. Mol. Sci. 2010;11:4750-63. [PubMed ID: 21151469].
-
18.
Ferreira SL, Bruns RE, Ferreira HS, Matos GD, David JM, Brandao GC, da Silva EG, Portugal LA, dos Reis PS, Souza AS. Box-Behnken design: An alternative for the optimization of analytical methods. Anal. Chim. Acta. 2007;597:179-86. [PubMed ID: 17683728].
-
19.
McCue P, Horii A, Shetty K. Mobilization of phenolic antioxidants from defatted soybean powders by Lentinus edodes during solid-state fermentation is associated with enhanced production of laccase. Innov.Food Sci. Emerg. Technol. 2004;5:385-92.
-
20.
Caligiani A, Palla G, Maietti A, Cirlini M, Brandolini V. 1H NMR fingerprinting of soybean extracts, with emphasis on identification and quantification of isoflavones. Nutrients. 2010;2:280-9. [PubMed ID: 22254020].
-
21.
Yang S, Wang L, Yan Q, Jiang Z, Li L. Hydrolysis of soybean isoflavone glycosides by a thermostable β-glucosidase from Paecilomyces thermophile. Food Chem. 2009;115:1247-52.
-
22.
Maitan-Alfenas GP, de A Lage LG, de Almedia MN, Visser EM, de Rezende ST, Guimaraes VM. Hydrolysis of soybean isoflavone glycosides by Debaromyces hansenii UFV-1 immobilized cells and free β-glucosidase. Food Chem. 2014;146:429-36. [PubMed ID: 24176363].
-
23.
Pandjaitan N, Hettiarachchy N, Ju ZY. Enrichment of genistein in soy protein concentrate with β-glucosidase. J. Food Sci. 2000;65:403-7.
-
24.
Shon M, Kim T, Sung N. Antioxidants and free radical scavenging activity of Phellinus baumii (Phellinus of Hymenochaetaceae) extracts. Food Chem. 2003;82:593-7.
-
25.
Okawa M, Kinjo J, Nohara T, Ono M. DPPH (1,1-diphenyl-2-picrylhydrazyl) radical scavenging activity of flavonoids obtained from some medicinal plants. Biol. Pharm. Bull. 2001;24:1202-5. [PubMed ID: 11642334].