Abstract
Keywords
Proteasome inhibitor Small molecule α β-unsaturated nitro Michael acceptor Aryl-2-nitrovinyl
Introduction
The ubiquitin-proteasome system (UPS) is the major intracellular protein degradation pathway in eukaryotic cells. This regulated pathway is responsible for more than 80% of intracellular protein degradation and controls most of the key cellular functions (1, 2). Studies have shown the essential role of UPS in most vital cellular functions, such as cell cycling, signal transduction, immune responses, and apoptosis (3). Protein degradation in the UPS consists of two major steps: the modification of a substrate by addition of poly ubiquitin chain; and subsequent degradation of ubiquitinated protein by 26S proteasome which is a multicatalytic proteinase complex (4, 5). Because of the functional role of this enzyme in cell survival, it has emerged as a promising therapeutic target and proteasome inhibitors have been investigated extensively as potential antitumor, antiviral, anti-inflammatory, and immunosuppressive agents. Furthermore, studies have indicated that tumor cells are more sensitive to proteasome inhibitors than normal cells, and therefore, the proteasome is regarded as one of the most promising antitumor drug targets (6, 7).
The 26S proteasome is a large multi catalytic protein complex, consisting of a cylindrical 20S proteolytic core and two 19S regulatory particles. The 20S core which is responsible for protein degradation comprises of 2 pairs of 14 different subunits and the active sites of the 20S proteasome are located on the β1, β2, and β5 subunits, with caspase-like (C-L), trypsin-like (T-L), and chymotrypsin-like (ChT-L) activities, respectively (3). In all three β-subunits the cleavage of peptide bonds occurs through a mechanism in which the hydroxyl group of N-terminal catalytic threonine residue (Thr1) serves as the nucleophile (2).
The high rate of protein production in cancer cells and the need for proteasome function in these cells for keeping up the cancer cell survival and proliferation, makes the proteasome pathway a validated target for cancer therapy. After the FDA approval of bortezomib, as a clinically effective reversible inhibitor of the β5 subunit, various proteasome inhibitors have been studied to combat the existing limitations. However, investigations for safer and less toxic alternatives with better pharmacokinetic properties are proceeding (8). Most proteasome inhibitors are composed of a short peptide backbone with an electrophilic trap to capture the hydroxyl group of the N-terminal threonine active site (9). However, these peptide-based drugs are susceptible to degradation and pose short in-vivo half-life. As peptide-based drugs suffer from some other limitations like chemical instability, immunogenicity and poor membrane permeability, efforts have been made to develop non-peptide synthetic proteasome inhibitors in the recent years (10).
The majority of proteasome inhibitors bear an electrophilic trap like epoxyketone, boronic acid, and aldehyde functional groups in their structures (11). In 1997 Bogyo et al. (12) introduced a new class of proteasome inhibitors with vinyl sulfone structure acting as a Michael acceptor pharmacophore. Furthermore, Overkleeft et al. (13) proposed various Michael acceptor containing peptide-based structures as proteasome inhibitors. On the other hand, studies of Baldisserotto et al. (14) showed that compounds bearing α,β-dehydro-phenylalanine are good substrates for Michael addition for catalytic threonine. In sight of these facts and aiming to find non-peptide small molecules, we have considered the synthesis and evaluation of proteasome inhibitory activity of small molecules bearing an α, β-unsaturated nitro functional group as a Michael acceptor in their structure. In this study, a series of substituted aryl-2-nitrovinyl derivatives were designed and synthesized. Docking studies were conducted for the synthesized compounds and the biological activities were also evaluated and compared to a known proteasome inhibitor, bortezomib.
Experimental
General methods
All the chemicals and solvents were purchased from Merck (Darmstadt, Germany). The melting points of the compounds were measured on 9100 Electrothermal melting point apparatus. Elemental analyses were performed by a Costech ECS 4010 CHNS analyzer. The 1H-NMR spectra were recorded on a 400 MHz Bruker spectrometer. IR spectra were recorded on Perkin Elmer IR spectrophotometer as potassium bromide discs. GC–MS analysis were carried out by using a 7000 Agilent triple Quadrupole MS system coupled with a 7890A GC, equipped with a split/splitless injection port, an autosampler model Agilent 7693, and electronic ionization. A HP-5MS 5% Phenyl Methyl Silox, Agilent 19091s-433 capillary column was used (30 m × 0.25 mm I.D. and 0.25 μm film thickness). Helium gas with a purity of 99.99% and a flow rate of 1 mL/min was used as the carrier gas.
General procedure for the synthesis of para-substituted nitrostyrene (1a-e)
Nitromethane (0.1 moles) and the appropriate aldehyde (0.1 moles) were dissolved in 20 mL of methanol in a beaker that was kept cool by a mixture of ice and salt. A cold solution of sodium hydroxide (10 mL, 0.2 M) was added from an addition funnel to the stirring solution of nitromethane and benzaldehyde at a rate that the temperature was kept below 15 °C. A precipitate was formed and got thick during the addition of sodium hydroxide solution. After fifteen minutes remaining in the lab, about 60 mL of ice water was added to the mixture and the pasty mass was converted to a clear solution. The reaction mixture was added dropwise to a solution of HCl (20 mL of concentrated HCl diluted to 50 mL with water). A solid crystalline mass was formed and separated by decantation. The residue was filtered and washed with water and recrystallized from ethanol (15).
(2-nitrovinyl)benzene (1a): Yellow solid. Yield: 83%. mp 57-58 °C. IR (KBr): 1635, 1523, 1348, 973, 778. GC-MS (m/z): 149.1 [M]+. 1H-NMR (400 MHz, CDCl3): 7.43-7.56 (5H, m, CHPh), 7.59 (1H; d, J = 13.7 Hz; =CHα), 8.01 (1H; d, J = 13.7 Hz; =CHβ). Anal. Calcd. For C8H7NO2 (149.15): C, 64.42; H, 4.73; N, 9.39; O, 21.45. Found: C, 64.37; H, 4.69; N, 9.41; O, 21.38.
1-chloro-4-(2-nitrovinyl)benzene (1b): Crystalline (needle shape), light yellowish solid. Yield: 46%. mp 111-113 °C. IR (KBr): 1627, 1587, 1525, 1343, 979. GC-MS (m/z): 183.0 [M]+. 1H-NMR (400 MHz, CDCl3): 7.44 (2H; d, J = 8.6 Hz; CHAr-O-Cl), 7.49 (2H; d, J = 8.6 Hz; CHAr-m-Cl), 7.56 (1H; d, J = 13.7 Hz; =CHα), 7.96 (1H; d, J = 13.7 Hz; =CHβ). Anal. Calcd. For C8H6ClNO2 (183.59): C, 52.34; H, 3.29; Cl, 19.31; N, 7.63; O, 17.43. Found: C, 52.32; H, 3.30; Cl, 19.30; N, 7.63; O, 17.41.
1-methyl-4-(2-nitrovinyl)benzene (1c): Yellow solid. Yield: 57%. mp 105-106 °C; IR (KBr): 1628, 1514, 1345, 967, 815. GC-MS (m/z): 163.1 [M]+. 1H-NMR (400 MHz, CDCl3): 2.41 (3H, s, Me), 7.26 (2H; d, J = 8Hz; CHAr-o-Me), 7.45 (2H; d, J = 8Hz; CHAr-m-Me), 7.57 (1H; d, J = 13.6 Hz; =CHα ), 7.99 (1H; d, J = 13.6 Hz; =CHβ). Anal. Calcd. For C9H9NO2 (163.17): C, 66.25; H, 5.56; N, 8.58; O, 19.61. Found: C, 66.22; H, 5.57; N, 8.56; O, 19.60.



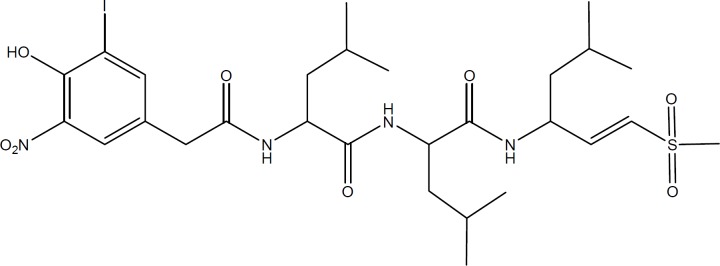
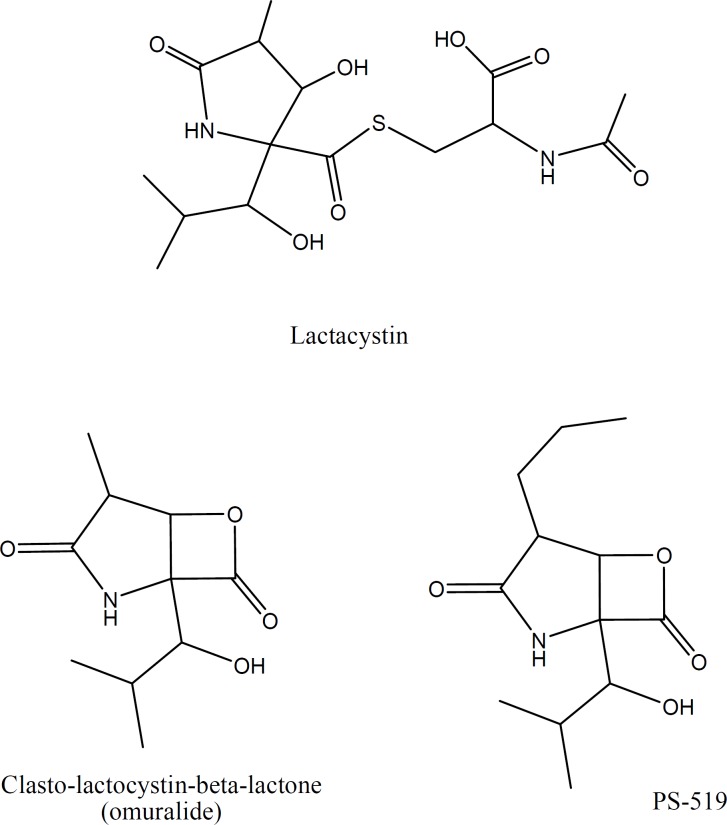
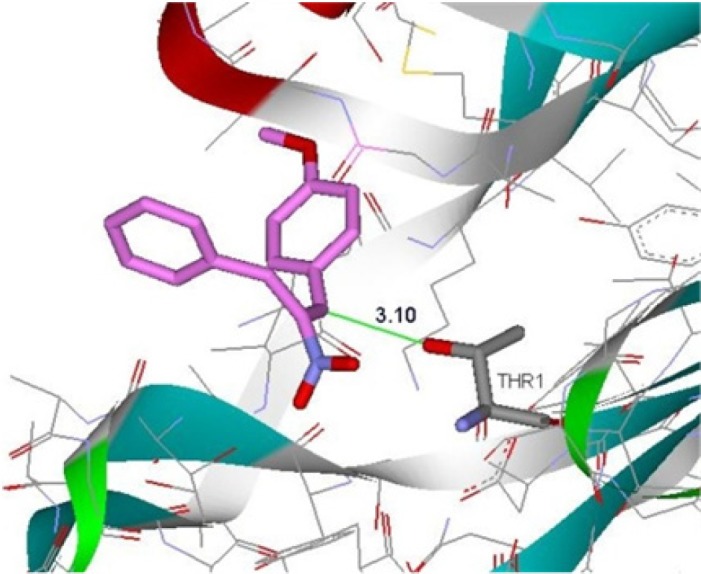
Cytotoxicity and proteasome ChT-L inhibitory activities of substituted aryl-2-nitrovinyl derivatives on MCF-7 and PC-3 cell lines.
1-methoxy-4-(2-nitrovinyl) benzene (1d): Crystalline (needle shape) yellow solid. Yield: 96%. mp 84-86 °C; IR (KBr): 1624, 1509, 1419, 1324, 973. GC-MS (m/z): 179.1 [M]+. 1H-NMR (400 MHz, CDCl3): 3.87 (3H, s, OMe), 6.95 (2H; d, J = 8.8 Hz; CHAr-o-OMe), 7.49-7.54 (3H, m, CHAr-m-OMe and =CHα), 7.97 (1H; d, J = 13.6 Hz; =CHβ). Anal. Calcd. For C9H9NO3 (179.17): C, 60.33; H, 5.06; N, 7.82; O, 26.79. Found: C, 60.31; H, 5.08; N, 7.80; O, 26.79.
N, N-dimethyl-4-(2-nitrovinyl)benzenamine (1e): Red solid. Yield: 30%. mp 182-184 °C; IR (KBr): 1668, 1547, 1493, 1339, 981. GC-MS (m/z): 192.1 [M]+. 1H-NMR (400 MHz, CDCl3): 3.08 (6H, s, CHN(Me)2), 6.9 (2H; d, J = 8.9 Hz; CHAr-o-N(Me)2), 7.43 (2H; d, J = 8.9 Hz; CHAr-m-N(Me)2), 7.50 (1H, d, J = 13.4 Hz; =CHα), 7.97 (1H, d, J = 13.4 Hz; =CHβ). Anal. Calcd. For C10H12N2O2 (192.21): C, 62.49; H, 6.29; N, 14.57; O, 16.65. Found: C, 62.48; H, 6.29; N, 14.55; O, 16.66.
General procedure for the synthesis of 2-nitro-3-arylprop-2-en-1-ol (2a-d)
To a stirred solution of substituted nitrostyrene (10 mmol) in THF (25 mL) at room temperature, imidazole (1 equivalent) and anthranilic acid (10 mol%) were added. Aqueous solution of formaldehyde (37%, 25 mL) was then added and the mixture was stirred at room temperature overnight. After completion of the reaction (monitored by TLC), the mixture was acidified with 5 M HCl (20 mL) and the aqueous layer was extracted with EtOAc (3 × 25 mL). The organic layers were washed with brine (50 mL), dried with anhydrous Na2SO4, and concentrated .The residue was purified by column chromatography (silica gel, EtOAc–hexanes, 0–30%, gradient elution) to obtain pure product (16).
2-Nitro-3-phenylprop-2-en-1-ol (2a): Yellow oil. Yield: 53%. IR (neat): 3437, 1658, 1531, 1238, 1027, 783. GC-MS (m/z): 179.1 [M]+. 1H-NMR (400 MHz, CDCl3): 2.67 (1H, s, br), 4.72 (2H, s, CH2), 7.48-7.58 (5H, m, CHPh), 8.22 (1H, s, =CH). Anal. Calcd. For C9H9NO3 (179.17): C, 60.33; H, 5.06; N, 7.82; O, 26.79. Found: C, 60.35; H, 5.06; N, 7.79; O, 26.80.
2-Nitro-3-(4-chlorophenyl)prop-2-en-1-ol (2b): Pale yellow oil. Yield: 32%. IR (neat): 3441, 1636, 1506, 1453, 1091, 841. GC-MS (m/z): 213.0 [M]+. 1H-NMR (400 MHz, CDCl3): 2.66 (1H, s, br), 4.67 (2H, s, CH2), 7.46 (2H; d, J = 8 Hz; CHAr-o-Cl), 7.52 (2H; d, J = 8 Hz; CHAr-m-Cl), 8.16 (1H, s, =CH). Anal. Calcd. For C9H8ClNO3 (213.62): C, 50.60; H, 3.77; Cl, 16.60; N, 6.56; O, 22.47. Found: C, 50.59; H, 3.78; Cl, 16.62; N, 6.54; O, 22.46.
2-Nitro-3-(4-tolyl)prop-2-en-1-ol (2c): Yellow solid. Yield: 38%. mp 52–54 °C. IR (KBr): 3444, 1660, 1539, 1342, 1030, 833. GC-MS (m/z): 193.1 [M]+. 1H-NMR (400 MHz, CDCl3): 2.42 (3H, s, Me), 2.67 (1H, s, br), 4.71 (2H; s, CH2), 7.29 (2H; d, J = 8 Hz; CHAr-o-Me), 7.47 (2H; d, J = 8 Hz; CHAr-m-Me), 8.19 (1H, s, =CH). Anal. Calcd. For C10H11NO3 (193.2): C, 62.17; H, 5.74; N, 7.25; O, 24.84. Found: C, 62.15; H, 5.74; N, 7.23; O, 24.85.
2-Nitro-3-(4-methoxyphenyl)prop-2-en-1-ol (2d): Yellow solid. Yield: 54%. mp 71–73 °C. IR (KBr): 3424, 1636, 1512, 1382, 1024, 831. GC-MS (m/z): 209.1 [M]+. 1H-NMR (400 MHz, CDCl3): 2.62 (1H, s, br), 3.48 (3H, s, OMe), 4.73 (2H, s, CH2), 6.99 (2H; d, J = 8 Hz; CHAr-o-OMe), 7.50 (2H; d, J = 8 Hz; CHAr-m-OMe), 8.19 (1H, s, =CH). Anal. Calcd. For C10H11NO4 (209.2): C, 57.41; H, 5.30; N, 6.70; O, 30.59. Found: C, 57.43; H, 5.31; N, 6.69; O, 30.60.
Synthesis of (2-nitroethyl)benzene (3)
To the stirred mixture of nitrostyrene (1a, 10 mmol), silica gel (20 g, column chromatography grade), 2-propanol (30 mL), and chloroform (160 mL) was added NaBH4 (40 mmol) in small portions over a period of 15 min at room temperature. The mixture was stirred for additional 15 min until the yellow color was completely disappeared. Excess NaBH4 was decomposed by addition of diluted HCl (0.1 N) and the mixture was filtered and washed with CH2C12. The combined filtrate was washed with brine, dried (Na2SO4) and then evaporated to give (2-nitroethyl)benzene as a clear oil (17).
IR (neat): 3063, 3031, 1608, 1555, 1380, 752. 1H-NMR (400 MHz, CDCl3): 5.44 (2H, s, CH2), 7.45-7.50 (5H, m, CHPh). Anal. Calcd. For C8H9NO2 (151.16): C, 63.56; H, 6.00; N, 9.27; O, 21.17. Found: C, 63.55; H, 6.02; N, 9.26; O, 21.14.
General procedure for the synthesis of aryl-2-nitroallylbenzene (4a-4e)
A mixture of (2-nitroethyl)benzene (15 mmol), dimethylamine hydrochloride (30 mmol), potassium fluoride (2.25 mmol) and appropriate aldehyde (15 mmol) in toluene (20 mL) was refluxed with a Dean–Stark trap for 24 h. The reaction mixture was then diluted with toluene (100 mL) and washed with 10% HCl solution (3 × 30 mL). The organic phase was dried over anhydrous Na2SO4 and solvent evaporated in-vacuo. The product was purified by recrystallization in a mixture of EtOAc/hexane or column chromatography over silica gel (EtOAc/hexane, 7/3) (18).
3-(phenyl)-2-nitroallylbenzene (4a): Yellow oil. Yield: 67%. IR (neat): 1649, 1533, 1392, 1331, 779. GC-MS (m/z): 239.1 [M]+. 1H-NMR (400 MHz, CDCl3): 4.27 (2H, s, CH2), 7.21-7.27 (5H, m, CHbenzyl ring), 7.42-7.47 (5H, m, CHPh), 8.31 (1H, s, =CH). Anal. Calcd. For C15H13NO2 (239.27): C, 75.30; H, 5.48; N, 5.85; O, 13.37. Found: C, 75.28; H, 5.49; N, 5.83; O, 13.35.
3-(4-chlorophenyl)-2-nitroallylbenzene (4b): Light brown solid. Yield: 78%. mp 98–100 °C. IR (KBr): 1651, 1520, 1325, 1021, 877. GC-MS (m/z): 273.1 [M]+. 1H-NMR (400 MHz, CDCl3): 4.22 (2H, s, CH2), 7.14 (2H; d, J = 8.4 Hz; CHAr-o-Cl), 7.29 (2H; d, J = 8.4 Hz; CHAr-m-Cl), 7.42-7.44 (5H, m, CHPh), 8.31 (1H, s, =CH). Anal. Calcd. For C15H12ClNO2 (273.71): C, 65.82; H, 4.42; Cl, 12.95; N, 5.12; O, 11.69. Found: C, 65.80; H, 4.40; Cl, 12.94; N, 5.14; O, 11.70.
3-(4-methylphenyl)-2-nitroallylbenzene (4c): Yellow-green solid. Yield: 73%. mp 105–107 °C. IR (KBr): 1665, 1520, 1393, 1329, 782. GC-MS (m/z): 253.1 [M]+. 1H-NMR (400 MHz, CDCl3): 2.32 (3H, s, Me), 4.22 (2H, s, CH2), 7.09-7.14 (4H, m, CHAr-o-Me and CHAr-m-Me), 7.40-7.46 (5H, m, CHPh), 8.28 (1H, s, =CH). Anal. Calcd. For C16H15NO2 (253.3): C, 75.87; H, 5.97; N, 5.53; O, 12.63. Found: C, 75.88; H, 5.96; N, 5.53; O, 12.61.
3-(4-methoxyphenyl)-2-nitroallylbenzene (4d): Yellow solid. Yield: 57%. mp 53–56 °C. IR (KBr): 1661, 1523, 1342, 1303, 878. GC-MS (m/z): 269.1 [M]+. 1H-NMR (400 MHz, CDCl3): 3.85 (3H, s, OMe), 4.31 (2H, s, CH2), 6.95 (2H; d, J = 8 Hz; CHAr-o-OMe), 7.20-7.39 (5H, m, CHPh), 7.45 (2H; d, J = 8 Hz, CHAr-m-OMe), 8.35 (1H, s, =CH). Anal. Calcd. For C16H15NO3 (269.3): C, 71.36; H, 5.61; N, 5.20; O, 17.82. Found: C, 71.35; H, 5.62; N, 5.23; O, 17.80.
N, N-dimethyl-4-(2-nitro-3-phenylprop-1-enyl)benzenamine (4e): Dark red solid. Yield: 76%. mp 110–113 °C. IR (KBr): 1599, 1530, 1386, 1252, 875. GC-MS (m/z): 282.1 [M]+. 1H-NMR (400 MHz, CDCl3): 3.02 (6H, s, N(Me)2), 4.34 (2H, s, CH2), 6.65 (2H; d, J = 9 Hz; CHAr-o-N(Me)2), 7.22-7.34 (5H, m, CHPh), 7.40 (2H; d, J = 9 Hz, CHAr-m- N(Me)2), 8.35 (1H, s, =CH). Anal. Calcd. For C17H18N2O2 (282.34): C, 72.32; H, 6.43; N, 9.92; O, 11.33. Found: C, 72.30; H, 6.44; N, 9.94; O, 11.32.
Synthesis of 3-methyl-1-nitrobutane (5)
Isopentyl iodide (50.0 mmol) dissolved in DMF (40 mL) was added dropwise to a stirred solution of DMF (150 mL) and sodium nitrite (100.0 mmol) at 0 °C. The mixture was stirred overnight at room temperature. Water (500 mL) was then added to the mixture and it was extracted with petroleum ether (4 × 70 mL). The combined organic phase was washed with water (2 × 50 mL) and dried with Na2SO4. The solvent was evaporated under reduced pressure to give 3-methyl-1-nitrobutane as colorless oil.
IR (neat): 2962, 2870, 1785, 1555, 1473, 1275, 1135. 1H-NMR (400 MHz, CDCl3): 0.89 (6H; d, J = 6.6 Hz; -CH3-isopropyl), 1.58-1.66 (1H, m, -CH-isopropyl), 1.85 (2H; q, J = 7.3 Hz; -CHβ), 4.34 (2H; t, J = 7.3 Hz, CH2). Anal. Calcd. For C5H11NO2 (117.15): C, 51.26; H, 9.46; N, 11.96; O, 27.32. Found: C, 51.27; H, 9.46; N, 11.95; O, 27.30.
General procedure for the synthesis of Aryl-4-methyl-2-nitropent-1-ene (6a-b)
To a stirred solution of 3-methyl-1-nitrobutane (28 mmol) and appropriate aldehyde (28 mmol) in MeOH (20 mL), AcOH (1.08 mL, 18.8 mmol) and n-BuNH2 (1.86 mL, 18.8 mmol) were added at 0 °C under argon. After being stirred for 36 h at room temperature, the reaction mixture was poured into water and then extracted with EtOAc. The organic layer was washed with water and brine, dried over Na2SO4 and concentrated. The resultant residue was purified by column chromatography on silica gel (EtOAc/hexane, 1/20) (19).
1-methyl-4-(4-methyl-2-nitropent-1-enyl)benzene (6a): Colorless oil. Yield: 40%. IR (neat): 1640, 1524, 1384, 1188, 770. GC-MS (m/z): 219.1 [M]+. 1H-NMR (400 MHz, CDCl3): 0.96 (6H; d, J = 6.8 Hz; -(CH3)2-isopropyl), 2.1 (1H; n, J = 6.8 Hz; -CH-isopropyl), 2.40 (3H, s, Me), 2.83 (2H; d, J = 7.2 Hz; CH2), 7.25 (2H; d, J = 8 Hz; CHAr-o-Me), 7.36 (2H; d, J = 8 Hz; CHAr-m-Me), 8.04 (1H, s, =CH). Anal. Calcd. For C13H17NO2 (219.28): C, 71.21; H, 7.81; N, 6.39; O, 14.59. Found: C, 71.19; H, 7.80; N, 6.39; O, 14.60.
1-methoxy-4-(4-methyl-2-nitropent-1-enyl)benzene (6b): Yellow oil. Yield: 44%. IR (neat): 1599, 1506, 1302, 1025, 832. GC-MS (m/z): 235.1 [M]+. 1H-NMR (400 MHz, CDCl3): 0.95 (6H; d, J = 6.8 Hz; -(CH3)2-isopropyl), 2.0 (1H; n, J = 6.8 Hz; -CH-isopropyl), 2.42 (2H; d, J = 7.2 Hz; CH2), 3.86 (3H, s, OMe), 6.97 (2H; d, J = 8 Hz; CHAr-o-OMe), 7.42 (2H; d, J = 8 Hz; CHAr-m-OMe), 8.05 (1H, s, =CH). Anal. Calcd. For C13H17NO3 (235.28): C, 66.36; H, 7.28; N, 5.95; O, 20.40. Found: C, 66.35; H, 7.29; N, 5.96; O, 20.39.
Cell viability assays
Human breast cancer (MCF-7), and human prostate cancer (PC-3) cells were cultured in their respective media supplemented with 10% fetal bovine serum (FBS). The cells were cultured at 1 × 104 cells per well, in a 96-well plate and incubated for 24-48 h to obtain cell attachments. Afterwards, the cells were treated with different concentrations of test compounds for 48 h and then incubated with 20 µL of 5 mg/mL 3-(4,5-dimethylthiazol-2-yl)-2,5-diphenyl tetrazolium bromide (MTT) solution for 4 h. After aspiration of the MTT solution, 100 µL of DMSO was added to each well to dissolve formazan crystals. The absorbance of each well was measured at the wavelength of 550 nm using an enzyme-linked immunosorbent assay reader. IC50 values were calculated from the curves generated by plotting the percentage of the viable cells versus the test concentration on a logarithmic scale using SigmaPlot 10.0 software.
Cell-based proteasome inhibition assay
The effect of the derivatives on the 20S proteasome activity was determined using the 20S proteasome activity assay kit from Sigma (USA). This assay measures the ChT-L protease activity in cultured cells and is based on the detection of the fluorophore R110 after cleavage from the labeled substrate LLVY-R110. MCF-7 and PC-3 cells were pretreated with different concentrations of corresponding compounds and proteasome activities were determined according to the manufacturer′s protocol. Proteasome activity generates strongly green fluorescent R110, which was monitored at an excitation wavelength of 480 nm and emission of 590 nm on a microplate fluorescence reader. All data were calculated by subtracting the blank control. The data are presented as percent inhibition at a specific concentration (10 µM for MCF-7 and 25 µM for PC-3), and IC50 values obtained from dose–response curves utilizing curve-fitting software package SigmaPlot 10.0 software.
Docking studies
The crystal structure of 20S proteasome (PDB code: 4INR) was obtained from the RCSB Protein Data Bank. The molecular docking study was performed using AutoDock Vina v.1.1.2. Protein was prepared for docking by removing co-crystallized ligand and all water molecules from crystal protein. Polar hydrogens were added and nonpolar hydrogens were merged, finally Kallman unitedatom charge and atom type parameter were added to 4INR. Grid box dimensions (20 × 20 × 20) were set surrounding active site (20).
Results and Discussion
Chemistry
The routes of synthesis for substituted aryl-2-nitrovinyl derivatives are outlined in Schemes 1–3. The para-substituted nitrostyrenes (1a-e) were prepared by the reaction of nitromethane with the appropriate aldehyde. The obtained substituted nitrostyrenes (1a-d) were then converted to 2-nitro-3-arylprop-2-en-1-ols (2a-d) in the presence of imidazole, anthranilic acid, and formaldehyde solution at room temperature according to the synthetic route shown in Scheme 1.
For synthesis of aryl-2-nitroallylbenzenes (4a-e), first (2-nitroethyl) benzene (3) was prepared by reduction of nitrostyrene (1a) in the presence of silica gel and NaBH4 in 2-propanol and chloroform. After that, compound 3 was reacted with appropriate aldehyde by adding dimethylamine hydrochloride and potassium fluoride in toluene.
Aryl-4-methyl-2-nitropent-1-ene (6a-b) was synthesized by the reaction of 3-methyl-1-nitrobutane (5) with the appropriate aldehyde in methanol, in the presence of n-BuNH2 and acetic acid at 0 °C and under argon. 3-methyl-1-nitrobutane (5) was prepared by the reaction of isopentyl iodide with sodium nitrite in DMF at 0 °C.
Biological evaluation
Proteasome inhibition have been proposed as a promising therapeutic target in medicinal chemistry and various reversible and irreversible proteasome inhibitors have been introduced in the literature (21). Most proteasome inhibitors are small peptides containing an electrophilic functional group at one end of their structures which form covalent bonds with the catalytic Oγ-threonine in the active site of 20S proteasome (10). Vinyl sulfones (Figure 1) were introduced as a pharmacophore in irreversible proteasome inhibitors by Bogyo et al. (12), and it is suggested that the hydroxyl group of the threonine residue in the active site of proteasome reacts with the double bond of vinyl sulfone moiety through a Michael addition mechanism.
Conjugation of vinyl group with sulfone moiety makes these compounds good Michael acceptors and thus good proteasome inhibitors. In the present study we designed and synthesized a group of nitrovinyl compounds (α, β–unsaturated nitro compounds) which are chemically eligible Michael acceptors and they can then be considered as potential proteasome inhibitors. The reported structure activity relationship for different classes of proteasome inhibitors such as peptide-aldehydes, vinyl sulfones, peptide boronates (bortezomib) and epoxyketones indicate that the presence of an amino acid residue, such as leucine or phenylalanine, in the vicinity of the pharmacophore group will increase the efficiency of proteasome inhibitors. Therefore, we tried three residues next to the nitrovinyl moiety and investigated their impact on proteasome inhibitory activity. The three residues were isopropyl, benzyl and hydroxymethyl, and they were placed on the alpha carbon with respect to the nitro group to avoid any possible steric hindrance on beta carbon.
The synthesized derivatives were tested for the antiproliferative and ChT-L proteasome inhibitory properties on two cancer cell lines including MCF-7 and PC-3 cells. A list of the designed compounds and their biological activity are summarized in Table 1. In order to investigate the nature of the interaction of these small molecules with the receptor, various functional groups with different lipophilicity, size and electronic properties were substituted at the R1 (Cl, CH3, OCH3, and N (CH3)2) and R2 (isopropyl, benzyl, or CH2OH) positions.
As shown in Table 1, most of the substituted aryl-2-nitrovinyl derivatives, with the exception of compounds 1e and 4e showed promising inhibitory activities toward ChT-L in both cell lines, inhibiting >40% of the proteasome activity at 10 and 25 µM concentrations for MCF-7 and PC-3, respectively. The 2-nitro-3-(4-methoxyphenyl)prop-2-en-1-ol (compound 2d) was the most potent proteasome inhibitor with IC50 values of 0.71 and 17.79 μM against MCF-7 and PC-3, respectively. These α,β–unsaturated nitro compounds also exhibited satisfactory cytotoxic effects against both MCF-7 (IC50 < 25) and PC-3 (IC50 < 80) cancer cell lines.
Among the R2 substituted derivatives, compounds with a CH2OH group on the α-carbon position of the nitro group (2a, 2c, 2d), generally showed the highest ChT-L inhibitory effects. These compounds exhibited lower antiproliferative and proteasome inhibitory IC50 values on both cell lines. Introducing benzyl ring and isopropyl group at the R2 position resulted in lower activities (i.e. 4c and 6a versus 2c) and the following order was seen for proteasome inhibitory potencies in the R2 position: CH2OH > H > CH2-Ph > CH (CH3)2; suggesting that parameters such as steric and electronic properties of the side chain on α-carbon position of the α,β-unsaturated nitro group may affect the activity of the compounds. Despite the fact that the best amino acid residue next to the pharmacophore group in boronates and vinyl sulfones seem to be isobutyl group, the presence of a hydroxymethyl group in the vicinity of pharmacophore moiety is also observed in the structures of Lactacystin (Figure 2) and its derivatives which are natural products with proteasome inhibitory activity.
Maintaining the R2 position constant, various para-substituted (2-nitrovinyl)benzene derivatives were synthesized and evaluated for ChT-L inhibitory properties. The presence of OMe group at R1 position (1d, 2d, 4d, and 6b) significantly improved the antiproliferative and ChT-L proteasome inhibitory activities in each series. The replacement of OMe with other substituents including Me, Cl, and N (CH3)2 reduced proteasome inhibitory effects whereas no inhibitory effects (IC50 > 50 μM) were observed for 1e and 4e in both cell lines, bearing N (CH3)2 group at R1 position. These results propose that the electronic properties of the substituent and steric hindrance at R1 position may have marked effects on ChT-L proteasome inhibitory properties.
Docking studies
Molecular docking studies were conducted to understand the interactions between the efficient derivatives and the active site of β5-subunit of the proteasome (Thr1). According to the results of docking studies (Figure 3), the distance between β-position of Michael acceptor and OH group of Thr1 in the active site of enzyme is about 3.1 Ǻ that can suggest the possibility of covalent interactions. The Gibbs free energy binding (ΔG), obtained from the result of molecular docking, showed that the more active derivatives have a satisfactory affinity to β5-subunit of proteasome with free binding energy of –6 kcal/mol.
Conclusion
In the present study a series substituted aryl-2-nitrovinyl derivatives have been synthesized and their inhibitory activities were assessed against β5-subunit of the 20S proteasome in two cell lines. The tested derivatives inhibited proteasome activity and cell proliferation with good IC50 values on both cell lines. Among the tested compounds, compound 2d proved to be the most potent proteasome inhibitor with IC50 value of 0.71 and 17.79 μM against MCF-7 and PC-3, respectively.
Acknowledgements
References
-
1.
Frankland-Searby S, Bhaumik SR. The 26S proteasome complex: An attractive target for cancer therapy. Biochim. Biophys. Acta. 2012;1825:64-76. [PubMed ID: 22037302].
-
2.
Kisselev AF, van der Linden WA, Overkleeft HS. Proteasome inhibitors: An expanding army attacking a unique target. Chem. Biol. 2012;19:99-115. [PubMed ID: 22284358].
-
3.
Delcros JG, Floc′h MB, Prigent C, Arlot-Bonnemains Y. Proteasome inhibitors as therapeutic agents: Current and future strategies. Curr. Med. Chem. 2003;10:479-503. [PubMed ID: 12570694].
-
4.
Gallastegui N, Groll M. The 26S proteasome: Assembly and function of a destructive machine. Trends Biochem. Sci. 2010;35:634-42. [PubMed ID: 20541423].
-
5.
Groll M, Huber R. Inhibitors of the eukaryotic 20S proteasome core particle: A structural approach. Biochim. Biophys. Acta. 2004;1695:33-44. [PubMed ID: 15571807].
-
6.
Kisselev AF, Goldberg AL. Proteasome inhibitors: From research tools to drug candidates. Chem. Biol. 2001;8:739-58. [PubMed ID: 11514224].
-
7.
Genin E, Reboud-Ravaux M, Vidal J. Proteasome inhibitors: Recent advances and new perspectives in medicinal chemistry. Curr. Top. Med. Chem. 2010;10:232-56. [PubMed ID: 20166955].
-
8.
Bettignies G, Coux O. Proteasome inhibitors: Dozens of molecules and still counting. Biochimie. 2010;92:1530-45. [PubMed ID: 20615448].
-
9.
Pevzner Y, Metcalf R, Kantor M, Sagaro D, Daniel K. Recent advances in proteasome inhibitor discovery. Expert Opin. Drug Discov. 2013;8:537-68. [PubMed ID: 23547800].
-
10.
Beck P, Dubiella C, Groll M. Covalent and non-covalent reversible proteasome inhibition. Biol. Chem. 2012;393:1101-20. [PubMed ID: 23091276].
-
11.
Crawford LJ, Walker B, Irvine AE. Proteasome inhibitors in cancer therapy. J. Cell Commun. Signal. 2011;5:101-10. [PubMed ID: 21484190].
-
12.
Bogyo M, McMaster JS, Gaczynska M, Tortorella D, Goldberg AL, Ploegh H. Covalent modification of the active site threonine of proteasomal beta subunits and the Escherichia coli homolog HslV by a new class of inhibitors. Proc. Natl. Acad. Sci. USA. 1997;94:6629-34. [PubMed ID: 9192616].
-
13.
Linden WA, Geurink PP, Oskam C, van der Marel GA, Florea BI, Overkleeft HS. Proteasome selectivity towards Michael acceptor containing oligopeptide-based inhibitors. Org. Biomol. Chem. 2010;8:1885-93. [PubMed ID: 20449494].
-
14.
Baldisserotto A, Marastoni M, Lazzari I, Trapella C, Gavioli R, Tomatis R. C-terminal constrained phenylalanine as a pharmacophoric unit in peptide-based proteasome inhibitors. Eur. J. Med. Chem. 2008;43:1403-11. [PubMed ID: 17996987].
-
15.
Worrall DE. Organic Synthesis, Collective. New York: Wiley Publishers; 1941. 413 p.
-
16.
Bakthadoss M, Sivakumar N, Devaraj A. First synthesis of bromo and chloro derivatives of Baylis–Hillman adducts derived from nitroolefins: Application towards the synthesis of a Dendrimer core. Sythesis. 2011;4:611-8.
-
17.
Sinhababu AK, Boschardt RT. Silica gel-assisted reduction of nitrostyrenes to 2-aryl-l-nitroalkanes with sodium borohydride. Tetrahedron Lett. 1983;24:227-30.
-
18.
Kodukulla R, Trivedi GK, Jyoti DV, Hari HM. Synthesis, chemical transformation and antimicrobial activity of a novel class of nitroolefins: 1,3-diaryl-2-nitroprop-l-enes. Synth. Commun. 1994;24:819-32.
-
19.
Asada M, Iwahashi M, Obitsu T, Kinoshita A, Nakai Y, Onoda T, Nagase T, Tanaka M, Yamaura Y, Takizawa H, Yoshikawa K, Sato K, Narita M, Ohuchida S, Nakai H and Toda M. 3-(2-Aminocarbonylphenyl)propanoic acid analogs as potent and selective EP3 receptor antagonists Part 1: Discovery and exploration of the carboxyamide side chain. Bioorg. Med. Chem. 2010;18:80-90. [PubMed ID: 20004584].
-
20.
Trott O, Olson AJ. AutoDock Vina: Improving the speed and accuracy of docking with a new scoring function, efficient optimization, and multithreading. J. Comput. Chem. 2010;31:455-61. [PubMed ID: 19499576].
-
21.
Eldridge AG, O′Brien T. Therapeutic strategies within the ubiquitin proteasome system. Cell Death Differ. 2010;17:4-13. [PubMed ID: 19557013].