Abstract
Keywords
Introduction
Anxiety and depression remain two of the most common psychiatric illnesses that affect humans (1). These disorders are associated with significant disability, socio-economic burden and a negative impact on the quality of life (2, 3). Current pharmacological interventions used in the management of these illnesses, unfortunately, often have numerous and serious side-effects, including: drug interactions, delayed response, and cases of non-response to treatment, among others (2,4). The use of traditional medicines continues to be the primary source of medical intervention worldwide, with 38-80% (depending on country of origin) utilizing traditional practices to address healthcare needs (5). Herbal products play an essential role in the drug discovery and development process, accounting for a majority of the approved chemotherapeutic agents on the market (6).
Promising anxiolytic and/or antidepressant effects have been reported from medicinal plants such as Citrus aurantium (7), Vitex negundo (8) and Nymphaea alba (9). The Nymphaeaceae family of aquatic angiosperms, commonly called water lilies, has a wide global distribution throughout the temperate and tropical regions. This family is divided into five subgenera: Barclaya, Euryale, Nuphar, Nymphaea, and Victoria (10). These plants are readily identified by their aquatic locality, large floating or submerged leaves, erect stem supporting a multi-petal (showy) inflorescence and large anchoring rhizomes. Nymphaeaceae subgenus Nymphaea has an extensive ethnobotanical history in several cultures. Current ethnobotanical use of Nymphaea spp. is quite diverse. Following traditional practices the flowers or rhizomes are generally the plant parts utilized in making liquors or aqueous infusions. Examples include the use of N. alba in Eurasia to deaden sexual desire (putative narcotic-type effect) (11) N. odorata by Chippewa Indians to treat mouth and lung disorders such as aphthous stomatitis and tuberculosis (11, 12), and N. ampla in Mayan areas for purported hallucinogenic effects (13, 14). The antioxidant, antibacterial, antiulcer and sedative effects of N. lotus have also been reported (15, 16, 17).
Research into the primary metabolites from members of the Nymphaea genus, and N. lotus in specific, has been reported (18). Additionally, there has been significant research into the secondary metabolites from the genus as well, with an emphasis on phytochemicals affecting inflorescence color variation. In this vein, numerous flavonoids, and anthocyanins in particular, have been isolated (19-25). Representative work from eight species of Nymphaea (N. alba, N. ampla, N. elegans, N gracilis, N. lotus, N. pulchella, N. x marliacea, and N. x marliacea var. Escarboucle) whose phytochemical studies utilized leaf or whole-plant material yielded 24 reported compounds (Table 1.). As these natural products were isolated (Figure 1A-M) from either leaf or whole plant material there is the possibility of co-occurrence within N. lotus. However, of these, there are only five secondary metabolites reported from N. lotus leaf: myricitrin (Figure 1L) 1, 2, 3, 4, 6-pentagalloylglucose (Figure 1J); nympholide A (Figure. 1K); nympholide B (Figure 1J); and myricetin-3’-O-(6”-p-coumaroyl) glucoside (Figure 1M) (16). The flavonol macrocycles nympholide A and nympholide B represent first-in-class compounds isolated from N. lotus (16).
Experimental
Botanical material
The leaves of Nymphaea lotus were collected from Ile Ife, Osun State, and Southwest Nigeria. They were authenticated by comparing with corresponding herbarium specimen (voucher number: LSH. 2012/6) deposited at the Lagos State University herbarium. The leaves were air dried and ground to powder prior to extraction.
Preparation of crude extract
Five kilograms of powdered plant material was macerated with 80% ethanol at room temperature. This was filtered and concentrated to dryness in vacuo at room temperature. The ethanolic extract (380 g) was suspended in water and successively partioned to give hexane extract (56g), dichloromethane (5.5 g), ethyl acetate (5g), butanol (7.4 g) and aqueous (131 g) fractions after removal of solvents in vacuo. The resulting fractions were stored at -10 °C until further use.
Drugs and Treatment
Kynuramine, Clorgyline, Deprenyl (Sigma-Aldrich, St. Louis, MO, USA), Tween 80 (2% Polyoxyethylenesorbitan monooleate), p-Chlorophenylalanine (PCPA) and Yohimbine (YOH) were purchased from Cristália, Itapira, SP, Brazil. Dimethyl Sulfoxide (DMSO; Sigma-Aldrich, St Louis, MO, USA), α-Methyl-p-tyrosine (AMPT; Sigma-Aldrich, St. Louis, MO, USA), Diazepam (DZP), Imipramine (IMI) and Prazosin (PRAZ) were purchased from Cristália, Itapira, SP, Brazil. Recombinant Human Monoamine Oxidase-A and –B (MAO-A and -B) were purchased from BD Biosciences Bedford, MA, USA. For in-vivo assay, drugs were dissolved in a vehicle [a mixture of 0.9% NaCl and 5% Tween-80 (v/v)] and administered (orally - p.o. or intraperitonealy - i.p) in a volume of 1 mL per 100 g of mice body weight. Drugs were dissolved in DMSO to yield a final DMSO concentration of 1.0% in the reaction mixture for in-vitro assay.
Pharmacological approaches
General pharmacological test
General pharmacological tests were conducted using a modified method of Malone [26] to detect general behavioral changes, effective doses and any signs of toxicity. Animals were treated through subcutaneous (s.c), intraperitoneal (i.p) or oral (p.o) route with CEN (5, 25, 125 or 625 mg/kg) or vehicle 10 mL/kg and observed periodically for 7 days.
Sodium pentobarbital sleep induction
Animals were treated orally with vehicle (10 mL/kg), CEN (20, 60 or 180 mg/kg) or diazepam (1 mg/kg) one hour prior to intraperitoneal administration of sodium pentobarbital (50 mg/kg). Sleep latency (time to the loss of righting reflex) and duration (voluntary recovery of the righting reflex) were recorded.
Rota-rod
The effect on motor coordination was assessed using a rota-rod apparatus. The rota-rod apparatus consisted of a base platform and an iron rod of 3 cm diameter and 30 cm length, with a non-slip surface. Mice were selected randomly, subjected to oral treatment with vehicle (10 mL/kg), CEN (20, 60 or 180 mg/kg) or diazepam (1 or 5 mg/kg) and placed individually to walk at 22rpm on the rotating rod one hour after treatment. Fall latency was recorded as an interval between the mounting of the animal on the rod and falling off of it.
Light/dark box test (LDB)
Mice were treated orally with vehicle (10 mL/kg), CEN (20, 60 or 180 mg/kg) or diazepam (DZP 1 or 5 mg/kg) prior to assessment in LDB. The animals were placed at the center of the light area facing the opening of the dark area one hour after treatment. The number of transitions between the two compartments and the time spent in the light area were recorded for five min.
Elevated plus maze test (EPM)
Mice were treated orally with vehicle (10 mL/kg), CEN (20, 60 or 180 mg/kg) or diazepam (DZP, 1 or 5 mg/kg) prior to assessment by elevated plus maze. One hour after treatment the animals were placed individually at the center of the plus maze and observed for five minutes. The number of entries into the open arms and time spent were recorded for statistical analysis.
Forced Swimming Test
Mice were treated orally with vehicle (10 mL/kg), CEN (20, 60 or 180 mg/kg) or imipramine (IMI, 15 mg/kg) and subjected to swimming for six minutes (27). The swimming duration and immobility periods were recorded. The immobility period was considered any time spent by the mouse floating and making passive movements necessary to keep its head afloat.
Phytochemical composition from eight representative members of Nymphaea.
Nymphaea spp. | Natural Product | Reference |
---|---|---|
N. alba | cyanidin 3-(6”-acetylgalactoside) (Figure.1 Gi); delphinidin 3-(2”-galloyl-6”-acetylgalactoside) (Figure.1 Gii); delphinidin 3-(6”-acetylgalactoside) (Figure.1 Giii); cyanidin 3-(2”-galloyl-6”-acetylgalactoside) (Figure.1 Giv); delphinidin 3-(2”-galloylgalactoside) (Figure.1 Gv); delphinidin 3-galactoside (Figure.1 Gvi); cyanidin 3-galactoside (Figure.1 Gvii) | (19) |
N. ampla | 7,3’4’-trihydroxy-5-O-β-D-(2”-acetyl)-xylopyranosylisoflavone (Figure 1 Ai); 7,3’,4’-trihydroxy-5-O-α-L-rhamnopyranosylisoflavone (Figure 1 Aii); quercetin 3-rhamnoside (Figure.1 Bii); quercetin 3-xylopyranoside (Figure 1 Biii); quercetin 3-glucopyranoside (Figure 1 Biv); methyl gallate (Figure.1 C) | (23) |
N. elegans | quercetin 3-rhamnoside (Figure 1 Bii); β-sitosterol (Figure 1 Fi); β-sitosteryl-3-O-β-D-glucopyranoside (Figure 1 Fii) | (23) |
N. gracilis | methyl 3-O-β-D-glucopyranosyloleanolate, (E) 28-O-β-D-glucopyranosyloleanolate (Figure 1 Dii); 28-O-β-D-glucopyranosyl-oleanolate (Figure 1 E); β-sitosterol (Figure 1 Fi); β- sitosteryl -3-O-β-D-glucopyranoside (Figure 1 Fii). | (23) |
N. lotus | myricitrin (Figure 1 I); 1,2,3,4,6-pentagalloyl-D-glucose (Figure 1 L); nympholide A (Figure 1 J); nympholide B (Figure 1 K); | (16,21) |
N. pulchella | 7,3’4’-trihydroxy-5-O-β-D-(2”-acetyl)-xylopyranosylisoflavone (Figure 1 Ai); 7,3’,4’-trihydroxy-5-O-α-L-rhamnopyranosylisoflavone (Figure 1 Aii); kaempferol 3-rhamnopyranoside (Figure 1 Bi); β-sitosterol (Figure 1 Fi); β- sitosteryl-3-O-β-D-glucopyranoside (Figure 1 Fii) | (23) |
N. x marliacea | myricetin 3-O-α-L-rhamnopyranosyl(1→6)β-D-galactopyranoside (Figure 1 H); delphinidin 3-(2”-galloyl-6”-acetylgalactoside) (Figure 1 Gii); delphinidin 3-(6”-acetylgalactoside) (Figure 1 Giii); delphinidin 3-galactoside (Figure 1 Gvi) | (22) |
N. x marliacea var. Escarboucle | delphinidin 3-(2”-galloyl-6”-acetylgalactoside) (Figure 1 Gii); delphinidin 3-(6”-acetylgalactoside) (Figure 1 Giii); cyanidin 3-(2”-galloyl-6”-acetylgalactoside) (Figure 1 Giv); delphinidin 3-(2”-galloylgalactoside) (Figure 1 Gv); delphinidin 3-galactoside (Figure 1 Gvi) | (22) |
The IC50 value of crude leaf extract of Nymphaea lotus (CEN) tested for inhibition of human MAO-A and MAO-B activities in-vitro
Sample | Unit | MAO-A IC50 | MAO-B IC50 |
---|---|---|---|
CEN | μg/mL | 149.503 ± 0.05 | - |
Clorgyline | μM | 0.024 ± 0.03 | - |
Deprenyl | μM | - | 0.072 ± 0.08 |
The effect of crude leaf extract of Nymphaea lotus (CEN) 20, 60 or 180 mg/kg on the level of hippocampal BDNF. Data are expressed as mean of pg/mg of protein ± S.E.M, n = 5 (One-way ANOVA followed by Dunnett´s test
Treatment (p.o) | [BDNF] pg/mg total protein |
---|---|
Vehicle 10 mL/kg | 80.32 ± 3.50 |
CEN 20 mg/kg | 70.72 ± 5.250 |
CEN 60 mg/kg | 84.33 ± 2.20 |
CEN 180 mg/kg | 86.05 ± 6.10 |
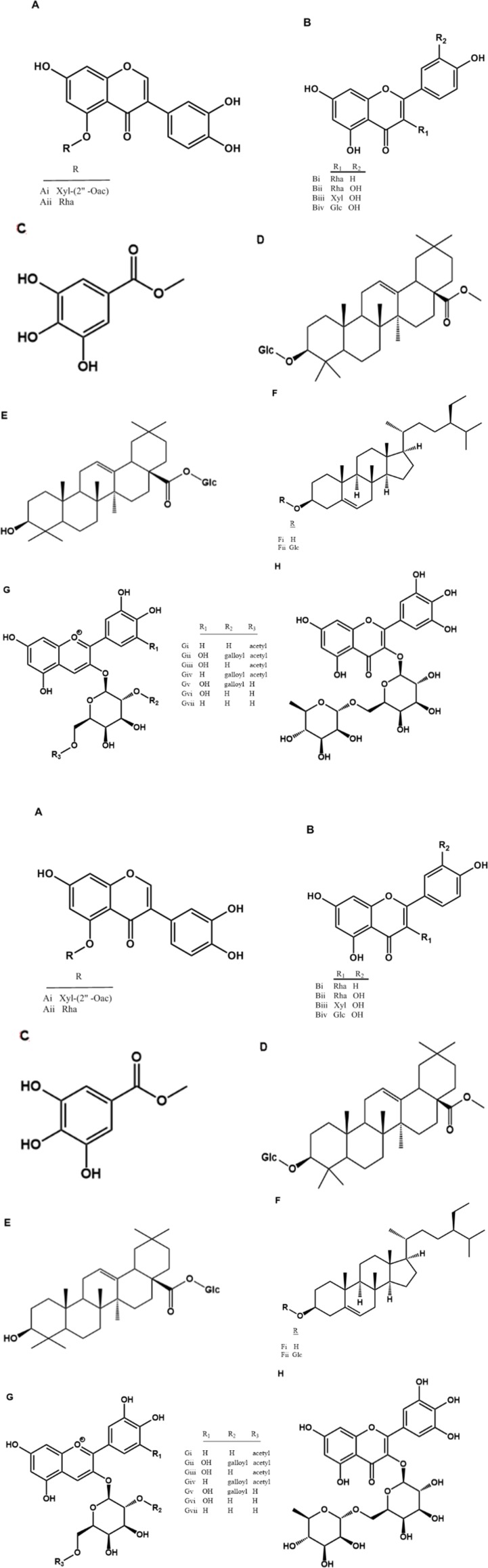
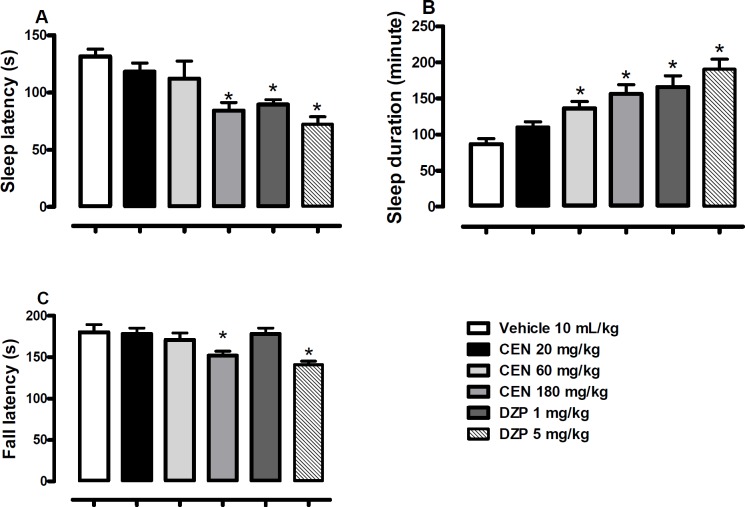
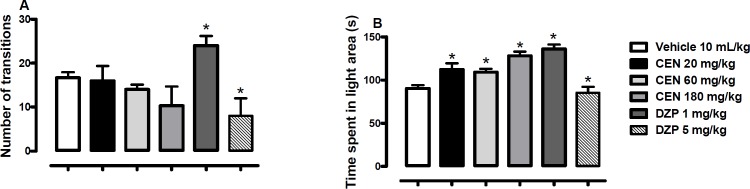
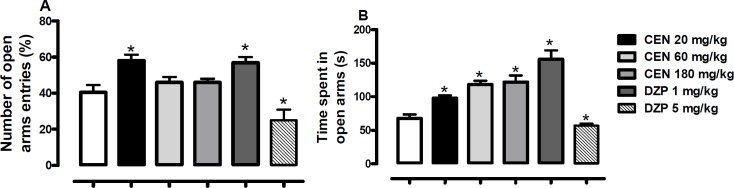
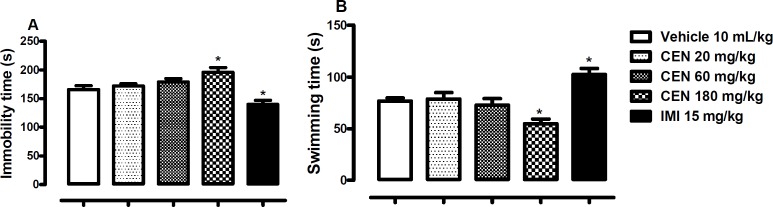
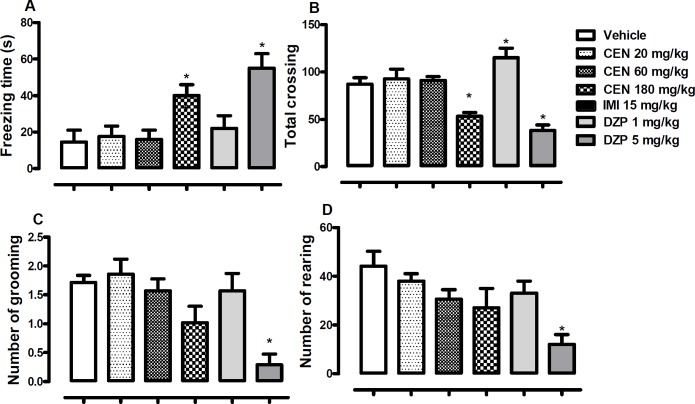
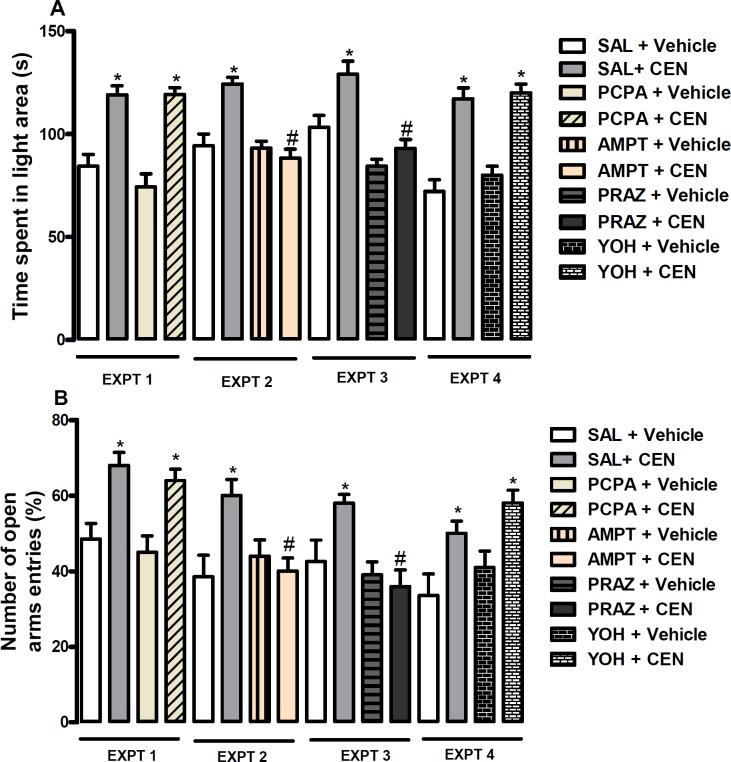
Open field exploratory activity
Mice were treated orally with vehicle (10 mL/kg), CEN (20, 60 or 180 mg/kg) or diazepam (DZP, 1 or 5 mg/kg) and then placed into a circular open field (50 cm high walled area whose 62.80 cm2 floor was divided into eight equal sectors). Parameters of total crossing, freezing time, number of grooming and rearing activities were scored during five minute test periods (27). The apparatus was cleaned with 10% alcohol at the end of each test.
Mechanism of anxiolytic like effect of CEN
Mice were pretreated intraperitoneally with NaCl 0.9% - SAL or PCPA 100 mg/kg (experiment-EXPT 1), NaCl 0.9% - SAL or AMPT 100 mg/kg (experiment-EXPT 2), NaCl 0.9% - SAL or PRAZ 0.5 mg/kg (experiment-EXPT 3) or NaCl 0.9% - SAL or YOH 1 mg/kg (experiment-EXPT 4) prior to oral treatment with vehicle or CEN 20 mg/kg. One hour post-treatment animal were evaluated using a forced swimming test.
Inhibition assay using recombinant human MAO-A and -B
A monoamine oxidase (MAO) inhibition assay utilizing kynuramine as the substrate was employed to investigate the potential activity of CEN on recombinant human MAO-A and MAO-B (28). CEN was assessed for inhibitory activity at a test range of 1-100 mg/mL, with clorgyline (10-10 - 10-5 M) and deprenyl (10-10 - 10-5 M) being employed as MAO-A and MAO-B selective inhibitors, respectively. The substrate concentration of 80 and 50 μM kynuramine was chosen for MAO-A and -B, respectively, based on the previous substrate concentration at half Vmax (29). Inhibition was calculated as percent of product formation compared to the corresponding control (enzyme-substrate reaction) without the inhibitors. The reactions were carried out in 0.1 M potassium phosphate buffer at pH 7.4, with incubation mixtures containing 5µg/mL of MAO-A or 12.5 µg/mL of MAO-B. The total reaction volume was 200 µL yielding a final DMSO concentration (vehicle) of 1.0% in the reaction mixture. Reaction mixtures were pre-incubated for 10 minutes at 37 °C prior to the addition of MAO-A or MAO-B to measure enzymatic activity. The reactions were stopped by the addition of 75 µL of 2N NaOH. The formation of 4-hydroxyquinoline was determined fluorometrically using a SpectraMax M5 fluorescence plate reader (Molecular Devices, Sunnyvale, CA) with 320 nm excitation and 380 nm emission wavelength calculated by the Soft Max Pro program.
Hippocampal protein extraction and BDNF quantification
Mice were treated orally of or vehicle 10 mL/kg, CEN 20, 60 or 180 mg/kg and sacrificed (after one hour) to collect their hippocampus. Hippocampal tissues were homogenized in a lysis buffer (NaCl 1mM; EDTA 4 mM, Tris-HCl 100 mM; albumin 2%, Triton-X 100 2%, thrimerosal 0.01%, pH 7.0, glycerol 10%, protease inhibitor cocktail - GE) in a 1:40 w/v ratio. After centrifugation (16800 x g, 4 °C, 35 min.), the supernatant was collected and stored at -80 °C. Hippocampal brain derived neurotrophic factor (BDNF) was measured using an ELISA kit (BDNF Emax® ImmunoAssay System kit, Promega, Madison, WI, USA) according to the manufacturer’s instructions.
The total protein level of sample was normalized and measured using the Bradford method
(30).
Statistical analysis
Parametric data are expressed as means ± SEM following appropriate statistical analysis, specifically: unpaired Student’s t-test, one way ANOVA followed by the Dunnett´s test as post hoc test or two way ANOVA followed by the Bonferroni as post hoc test. Significance difference between or among groups were set at p < 0.05 (31). GraphPad Prism statistical software version 6.03 for Windows (GraphPad Software, Inc., San Diego, CA, USA) was used for data analysis.
Results
General pharmacological test of CEN effect onmice
There were no observations of abdominal contortion, environmental alienation, ataxia, sedation, or loss of paw grip by 5, 25 and 125 kg/mg) administration. An increase in exploratory activity was observed after oral administration of CEN 5, 25 and 125 mg/kg). CEN 625 mg/kg) induced sedation, analgesia and loss of paw grip via i.p and s.c. irrespective of the administration route and dose. No deaths were observed in the test population during the seven day observation period following CEN
administration.
Effect of CEN on hypnotic effect of barbiturate and rota-rod test performance
Oral administration of CEN 180 mg/kg) or diazepam (DZP, 1 and 5 mg/kg) elicited a decrease in the sleep latency [F (5, 54) = 7.0, p < 0.05, one way ANOVA, Figure. 2A]. CEN elicited an increase in sleep duration [F (5, 54) = 10.7, p < 0.05, one way ANOVA, Figure. 2B]. The Dunnett´s post-hoc test revealed a significant decrease in sleep latency by CEN 180 mg/kg (p < 0.05) and an increase in sleep duration by CEN 60 and 180 mg/kg (p < 0.05). A significant decrease in fall latency was observed for CEN 180 mg/kg) and diazepam (DZP, 5 mg/kg) in the rota-rod test when compared to the control animals [F (5, 54) = 5.3, p < 0.05, one way ANOVA, Figure 2C].
Effects of CEN on mice in the light/dark box (LDB)
CEN 20, 60 or 180 kg/mg) administration did not statistically alter the number of transition [F (5, 54) = 3.4, p > 0.05, Figure 3A] in the LDB test; the control, diazepam, did at both test concentrations (1 and 5 mg/kg). However, the time spent in the light areas of the LDB was increased by CEN 20, 60 and 180 kg/mg) administration [F (4, 35) = 13.1, p < 0.05, Figure. 3B].
Effects of CEN on mice in the elevated plus maze
Oral administration of CEN increased the number of open arm entries [F (5, 54) = 10.9, p > 0.05, Figure 4A] at a dose of 20 mg/kg. The time spent on the open arms was also increased by CEN administration [F (5, 54) = 22.4, p < 0.05, Figure 4B]. The DZP 1 mg/kg increased the number of open arm entries [F (5, 54) = 10.9, p < 0.05, Figure 4A] and time spent on the open arms [F (5, 54) = 10.9, p < 0.05, Figure 4B]. At 5 mg/kg, DZP reduced the number of open arm entries [F (5, 54) = 10.9, p < 0.05, Figure 4A] and time spent on the open arms
Effects of CEN on mice in the Forced Swimming Test (FST)
Oral administration of CEN 180 mg/kg elicited significant increase in immobility time [F (4, 45) = 10.0, p < 0.05, Figure 5A] and decrease in swimming time [F (4, 45) = 9.8, p < 0.05, Figure. 5B] in the FST. The by IMI 15 mg/kg reduced immobility time (p < 0.05, Figure 5A) and increased swimming time (p < 0.05, Figure 5B).
Effect of CEN on mice behaviour in the open field
CEN 180 mg/kg) and diazepam (DZP, 5 mg/kg) treatments increased observed freezing time [F (5, 54) = 6.4, p < 0.05, Figure 6A]. Administration of CEN did not alter observed open field crossing by mice in a statistically significant manner [F (5, 54) = 15.3, p > 0.05, Figure 6B], however, DZP 1 mg/kg increased total crossing, while DZP 5 mg/kg reduced total crossing.
Mechanism of anxiolytic like property of CEN
The effect of pretreatment with SAL or PRAZ and treatment with vehicle or CEN at 20 mg/kg on time spent in the light area of LDB (dependent variable, Figure 7A) and percentage of open arms entries, Figure 7B) of the EPM were assessed. The data obtained on time spent in the light area of LDB did not reveal an interaction between pretreatment and treatment (p > 0.05, two-way ANOVA). In Figure 7A, Bonferroni post hoc test showed an increase in the time spent in the light area by CEN (i.e SAL + CEN vs SAL + Vehicle, p < 0.05) treatment. Unlike PCPA (EXPT 1, Figure 7 A and B) and YOH (EXPT 4, Figure 7 A and B) pretreatments, the effect of CEN on time spent in the light area of LDB and percentage of open arms entries in EPM was blocked by AMPT (i.e SAL + CEN vs AMPT + CEN, p < 0.05, EXPT 1, Figure 7A and B, respectively) and PRAZ (i.e SAL + CEN vs PRAZ + CEN, p < 0.05, EXPT 1, Figure 7A and B, respectively) pretreatments.
Effects of CEN on the activities of MAO enzymes
CEN did not show statistically significant inhibition of either MAO-A or MAO-B (Table 2). The controls, clorgyline and deprenyl demonstrated potent inhibition of their respective targets, MAO-A and MAO-B, respectively (Table 2).
Effects of CEN on hippocampal brain-derived neurotrophic factor (BDNF)
Oral administration of CEN (20, 60 or 180 mg/kg) did not statistically alter hippocampal level of BDNF [F (3, 16) = 2.3, p > 0.05, Table 3].
Discussion
There continues to be a critical need for effective therapeutic agents capable of producing antidepressant or anxiolytic effects to reduce the global burden of these disorders (32, 33). Medicinal plants, and the natural products they produce, remain an indispensable resource of potential therapeutic agents capable of these effects. However, to utilize these natural products in the drug discovery and development process a key step is identifying desired biological activity. With this in mind, this study reports, in-vivo assessment of N. lotus leaf extract (CEN) for anxiolytic and antidepressant activity.
Behavioral alteration in the general pharmacological tests included an increase in exploratory activity following oral administration of CEN without any observation of abdominal contortion, environmental alienation, ataxia, loss of paw grip, or sedation at any but the highest dose. The highest dose of N. lotus leaf extract (625 mg/kg) induced sedation, however there were no signs of overt toxicity or death of test animals in the course the study. The oral route of administration and the dose ranges of CEN in our subsequent experiments were based on the popular application of the leaf extract.
Barbiturate sleep induction is an established animal model used to investigate potential CNS depressive or stimulating agents. Potentiation of the hypnotic effect of barbiturate is an indication of central nervous system depressive activity (33). Like diazepam, oral administration of CEN elicited a reduction in latency and increase in the duration of sodium pentobarbital-induced sleep. This similarity to diazepam accentuated the importance of assessing the effects on motor coordination.
The rota-rod test is a test of motor coordination (34-37) intoxication (38), sedation and strength (39). The fall latency from a rotating rod is scored. A decrease in latency to fall indicates motor incoordination. In the current study, CEN at 180 mg/kg and diazepam at 5 mg/kg reduced the measured latency thereby suggesting interference with motor coordination by these compounds at the respective doses. This data may support the ethnobotanical use of N. lotus for sedative effects (40).
The light/dark box (LDB) assay is a well-established method to assess potential anxiolytic activities in-vivo (41). This model assesses the behavioral aversion of the test animal in an unknown, possibly dangerous environment, conflicting with the innate desire to explore the new environment. Suppression of aversive behavior by test compounds may indicate possible anxiolytic activity. This is assessed by measuring changes in the number of transitions between the light and dark areas of the LDB, as well as, relative time spent in light and dark areas. In this study, although the number of transitions was not statistically significant, the time spent in the light area of the LDB was significantly increased following CEN treatment at all tested doses. To corroborate these results a secondary anxiety model, the elevated plus maze (EPM) (42), was used to assess possible anxiolytic activity of N. lotus. Once more, at all tested doses (20, 60 and 180 mg/kg), N. lotus treated mice spent statistically more time in the open/exposed areas of the maze. At the 20 mg/kg dose the treated mice had increased EPM arm entries which were statistically significant when compared to the control. These results, together, indicate possible anxiolytic effects by N. lotus.
The forced swim test (FST) was utilized to assess antidepressant effects of N. lotus in mice. Oral administration of CEN at 20 and 60 mg/kg did not statistically alter the immobility or swimming time. However, CEN at 180 mg/kg increased immobility and decrease swimming time suggesting possible prodepressive effects at this dose. As this same dose induced motor incoordination on the rota-rod test an open field test was administered to discern differences between behavioral effects and motor effects.
The open field test (OFT) is the most basic measure of interest is locomotion. This can be influenced by motor output, exploratory drive, and fear-related behavior among other variables (43). However, more importantly OFT data can indicate possible sedative or myorelaxant activities that may affect other assay (such as FST). Administration of CEN at 180 mg/kg increased the freezing time and reduced total crossing, indicating that CEN may possess sedative or myorelaxant activities. When combined with the observed rota-rod motor incoordination this seems consistent. Possible neural mechanisms of CEN activity were evaluated using pharmacological tools p-chlorophenylalanine methyl ester (PCPA), α-methyl-p-tyrosine (AMPT), prazosin (PRAZ), and yohimbine (YOH). The depletion of serotonin with PCPA and pretreatment with alpha-2 adrenergic antagonist, YOH, did not block the anxiolytic-like effect of CEN. On the contrary, depletion of catecholamine with AMPT and pretreatment with alpha-1 adrenergic antagonist, PRAZ, attenuated anxiolytic-like effect of CEN in mice when assessed in both the light/dark box and the elevated plus maze. Lastly, as the mechanism of anxiolytic activity of CEN could involve inhibition of MAO activity, this too was assessed in-vitro against human MAO-A and MAO-B; however there was no significant inhibitory activity.
Conclusion
In conclusion, these results indicate that N. lotus may possess anxiolytic properties, with possible adrenergic involvement, and that additional research is warranted.
References
-
1.
Prince M, Patel V, Saxena S, Maj M, Maselko J, Philips MR, Rahman A. No health without mental health. Lancet. 2007;370:859-77. [PubMed ID: 17804063].
-
2.
World Health Organization (WHO). 54th World Health Assembly. 2001.
-
3.
Kaplan RM, Anderson JP. The General Health Policy Model: An Integrated Approach: Quality of Life Assessments in Clinical Trials. New York: Raven Press, Ltd; 1990.
-
4.
Markou A, Cryan JF. Stress, anxiety and depression: Toward new treatment strategies. Neuropharmacology. 2012;62:1-2. [PubMed ID: 21983630].
-
5.
World Health Organization (WHO). WHO Traditional Medicine Strategy 2002-2005. 2002.
-
6.
Newman DJ, Cragg GM. Natural Products as Sources of New Drugs over the 30 Years from 1981 to 2010. J. Nat. Prod. 2012;75:311-35. [PubMed ID: 22316239].
-
7.
Costa ARA, Cury TC, Cassettari BO, Takahira RK, Flório JC, Costa M. Citrus aurantium L essential oil exhibits anxiolytic-like activity mediated by 5-HT1A-receptors and reduces cholesterol after repeated oral treatment. BMC Comp. Alt. Med. 2013;13:42.
-
8.
Adnaik RS, Pai PT, Sapakal VD, Naikwade NS, Magdum CS. Anxiolytic activity of Vitex Negundo Linn In experimental models of anxiety in mice. Int. J. Green Pharm. 2009;3:243-7.
-
9.
Thippeswamy BS, Mishra B, Veerapur VP, Gupta G. Anxiolytic activity of Nymphaea alba Linn in mice as experimental models of anxiety. Ind. J. Pharm. 2011;43:50-5.
-
10.
Borsch T, Hilu KW, Wiersema JH, Lohne C, Barthlott W, Wilde V. Phylogeny of Nymphaea (Nymphaeaceae): Evidence from substitutions and microstructural changes in the chloroplast trnT-trnF region. Int. J. Plant Sci. 2007;168:639-71.
-
11.
Lewis WH, Elvin-Lewis MPF. Medical Botany: Plants Affecting Human Health. 2003.
-
12.
Foster S, Duke JA. Easter/Central Medicinal Plants and Herbs. Pet. 2000;3:18-9.
-
13.
Diaz JL. Ethnopharmacology of sacred psychoactive plants used by the indians of Mexico. Ann. Rev. Pharm. Toxicol. 1977;17:647-75.
-
14.
Ott J. Ethneogenic drugs, their plant sources and history. Pharmacotheon. 1993;7:424-5.
-
15.
John-Africa LB, Idris-Usman MS, Adzu B, Gamaniel KS. Protective effects of the aqueous extract of Nymphaea lotus L (Nymphaeaceae) against ethanol-induced gastric ulcers. Int. J. Bio. Chem. Sci. 2012;6:1917-25.
-
16.
Elegami AA, Bates C, Gray AI, Mackay SP, Skellern GG, Waigh RD. Two very unusual macrocyclic flavonoids from the water lily Nymphaea lotus. Phytochem. 2003;63:727-31.
-
17.
Afolayan AJ, Sharaibi OJ, Kazeem MI. Phytochemical Analysis and in-vitro Antioxidant Activity of Nymphaea lotus L. Int. J. Pharm. 2013;9:297-304.
-
18.
Park Y, Towantakavanit K, Kowalska T, Jung S, Ham K, Heo G, Cho J, Yun J, Gorinstein S. Bioactive Compounds and Antioxidant and Antiproliferative Activities of Korean White Lotus Cultivars. J. Med. Food. 2009;12:1057-64. [PubMed ID: 19857070].
-
19.
Fossen T, Andersen OM. Cyanidin 3-(6’’-acetylgalactoside) and other anthocyanins form reddish leaves of the water lily, Nymphaea alba. J. Hort. Sci. Biotech. 2001;76:213-5.
-
20.
Fossen T, Andersen OM. Delphinidin 3’-galloylgalactosides from blue flowers of Nymphaea caerulea. Phytochem. 1999;50:1185-8.
-
21.
Mukherjee PK, Mukherjee D, Maji AK, Rai S, Heinrich M. The sacred lotus (Nelumbo nucifera) – phytochemical and therapeutic profile. J. Pharm. Pharm. 2009;61:407-22.
-
22.
Fossen T, Andersen OM. Acylated anthocyanins from leaves of the water lily, Nymphaea x Marliacea. Phytochem. 1997;46:353-7.
-
23.
Marquina S, Bonilla-Barbosa J, Alvarez L. Comparative phytochemical analysis of four Mexican Nymphaea species. Phytochem. 2005;66:921-7.
-
24.
Agnihotri VK, ElSohly HN, Khan SI, Smillie TJ, Khan IA, Walker LA. Antioxidant constituents of Nymphaea caerulea flowers. Phytochem. 2008;69:2061-6.
-
25.
Zhu M, Zheng X, Shu Q, Li H, Zhong P, Zhang H, Xu Y, Wang L, Wang L. Relationship between the Compsition of Flavonoids and Flower Colors Variation in Tropical Water Lily (Nymphaea) Cultivars. PLoS ONE. 2012;7:34335.
-
26.
Malone MH. Pharmacological approaches to natural products screening and evaluation, in Wagner H, Wolf P (eds), Natural products and plant drugs with pharmacological, biological or therapeutical activity. Springer-Verlag, Berlin. 1977:23-53.
-
27.
Fajemiroye JO, Galdino PM, Alves SF, de Paula JA, de Paula JR, Ghedini PC, Costa EA. Involvement of 5-HT1A in the anxiolytic-like effect of dichloromethane fraction of Pimenta pseudocaryophyllus. J. Ethnopharm. 2012;3:872-7.
-
28.
Chaurasiya ND, Ibrahim MA, Muhammad I, Walker LA, Tekwani BL. Monoamine oxidase inhibitory constituents of propolis: kinetics and mechanism of inhibition of recombinant human MAO-A and MAO-B. Molecules. 2014;19:18936-52. [PubMed ID: 25412041].
-
29.
Parikh S, Hanscom S, Gagne P, Crespi C, Patten CA. Fluorescent-Based, High-Throughput Assay for Detecting Inhibitors of Human Monoamine Oxidase A and B; S02T081R2. BD Biosciences Discovery Labware. MA, USA: Woburn; 2002.
-
30.
Bradford MM. A rapid and sensitive method for the quantitation of microgram quantities of protein utilizing the principle of protein dye binding. Anal. Biochem. 1976;72:248-54. [PubMed ID: 942051].
-
31.
Sokal RR, Rohlf FJ. Biometry: the principles and practice of statistics. Biological Research. 2 ed. New York: W.H. Freeman and Company; 1981. 859 p.
-
32.
Bebbington PE. Population surveys of psychiatric disorder and the need for treatment. Soc. Psych. Psych. Epidem. 1990;25:33-40.
-
33.
Fajemiroye JO, Galdino PM, Alves SF, Paula JAM, de Paula JR, Ghedini PC, Costa EA. Involvement of 5-HT1A in the anxiolytic-like effect of dichloromethane fraction of Pimenta pseudocaryophyllus. J. Ethnopharm. 2012;141:872-7.
-
34.
Jones BJ, Roberts DJ. The quantiative measurement of motor incoordination in naive mice using an acelerating rotarod. J. Pharm. Pharm. 1968a;20:302-4.
-
35.
Jones BJ, Roberts DJ. A rotarod suitable for quantitative measurements of motor incoordination in naive mice. Naun. Arch. Exp. Pat. Pharm. 1968b;259:211.
-
36.
Pritchett K, Mulder GB. The rotarod Contemporary Topics in Laboratory. An. Sci. 2003;42:49.
-
37.
Watzman N, Barry H, Buckley JP, Kinnard WJ. Semiautomatic System for Timing Rotarod Performance. J. Pharm. Sci. 1964;53:1429-30. [PubMed ID: 14253619].
-
38.
Boehm SL, Schafer GL, Phillips TJ, Browman KE, Crabbe JC. Sensitivity to ethanol-induced motor incoordination in 5-HT (1B) receptor null mutant mice is task-dependent: implications for behavioral assessment of genetically altered mice. Behav. Neurosci. 2000;114:401-9. [PubMed ID: 10832800].
-
39.
Anthony JA, Olubunmi JS, Mutiu IK. Phytochemical Analysis and in-vitro Antioxidant Activity of Nymphaea lotus L. Int. J. Pharm. 2013;9:297-304.
-
40.
Perrine JW, Takesue EI. Use of the rotarod in determining grip strength in rats with adjuvant-induced arthritis. Arch. Intern. Phar. Ther. 1968;174:192-8.
-
41.
Crawley J, Goodwin FK. Preliminary report of a simple animal behavior model for the anxiolytic effects of benzodiazepines. Pharm. Biochem. Behav. 1980;13:167-70.
-
42.
Hogg S. A review of the validity and variability of the elevated plus-maze as an animal model of anxiety. Pharm. Biochem. Behav. 1996;54:21-30.
-
43.
Gould TD, Dao DT, Kovacsics CE. Mood and Anxiety Related Phenotypes in Mice: Characterization Using Behavioral Tests. Neuromethods. 2009;42:1-20.
-
44.
Carlini EA, Burgos V. Screening farmacológico de ansiolíticos: Metodologia laboratorial e comparacão entre diazepam e clorobenzepam. Rev. Ass. Med. Bras. 1979;1:25-31.
-
45.
Bogo V, Hill TA, Young RW. Comparison of accelerod and rotarod sensitivity in detecting ethanol- and acrylamide-induced performance decrement in rats: review of experimental considerations of rotating rod systems. Neurotox. 1981;2:765-87.
-
46.
Crawley JN, Goodwin FK. Preliminary report of a simple animal behavior for the anxiolytic effects of benzodiazepines. Pharm. Biochem. Behav. 1980;13:167-70.
-
47.
Pellow S, Chopin P, Briley M, File SE. The validation of open: closed arm entries in an elevated plus-maze: a novel test of anxiety in the rat. J. Neurosci. Meth. 1985;14:49-167.
-
48.
Porsolt RD, Le Pichon M, Jalfre M. Depression: A new animal model sensitive to antidepressant treatments. Nature. 1977;266:730-2. [PubMed ID: 559941].