Abstract
Keywords
Acute Kidney Injury Bilateral Renal Ischemia Erythropoietin Cognitive Impairments Memory
Introduction
Acute kidney injury (AKI) is a common serious condition that some of critically ill patients especially in intensive care units suffer from that (1). Renal ischemia reperfusion is a leading cause of AKI (2, 3). Despite advancements in renal replacement therapy, the mortality rate during AKI has remained unchanged, which is largely due to the remote organ dysfunction (4). Accordingly, understanding the specific extrarenal manifestations of AKI would facilitate the design of therapeutic interventions to reduce morbidity and mortality after AKI.
Particularly, AKI can adversely affect the brain (4, 5). Cognitive changes following renal disease have been demonstrated recently. Patients with AKI commonly develop encephalopathy and altered higher mental functions (6). Hemodialysis and chronic kidney disease (CKD) patients suffer moderate to severe cognitive impairment which is dependent of the severity of CKD (7, 8). Despite of these findings, the reasons of cognitive alterations are largely unknown. Experimental evidences have established increased neuronal pyknosis, microgliosis, and inflammation in CA1 region of hippocampus which plays an important role in learning, memory and anxiety (9, 10). These evidences caused to select the hippocampus for investigation in this research.
There are some evidences indicating the beneficial effects of EPO including anti-apoptotic, anti-inflammatory, antioxidant, and angiogenic effects (11-13). Furthermore, the expression of EPO and EPOR (erythropoietin receptor) mRNA has been showed in the hippocampus which is increased after hypoxia (14, 15). Several lines of evidences demonstrate neuroprotection effects of EPO in different animal models of brain injury such as spinal cord injury (16-18), stroke (19, 20) and autoimmune encephalomyelitis (21, 22). Recently, brain-derived neurotrophic factor (BDNF) is also known to be useful to neuronal functions and protect the CNS against a variety of brain injuries (23-25).
Encouraged by these reports, in the present study, we first investigated the effect of AKI on cognitive performance of rats and potential neuroprotective impact of EPO to suggest a possible therapeutic intervention in clinical practice, then to determine the possible molecular mechanism responsible for AKI-induced alterations of memory function, we examined BDNF expression in the hippocampus of the experimental groups.
Experimental
All animal study protocols were approved by the animal care and use committee of the Kerman Medical University (Ethics Code: KNRC/92/6). Male Wistar rats (8-10 weeks old, 180-220 g) were housed under standard conditions (12/12 light dark cycle) throughout the study.
Experimental groups
In the present study, 80 male Wistar rats were randomly allocated into 8 experimental groups. The rats subjected to BRI/reperfusion were divided into four groups of n = 10, in which 0.5 mL of normal saline alone or normal saline containing EPO (1000 U/kg) was i.p. injected 30 min before the induction of renal ischemia followed by the reperfusion periods of 24 h (BRI-24 h +V group and BRI-24 h +EPO group, respectively) and 1 week (BRI-1w+V group and BRI-1w +EPO group, respectively). Sham-operated rats were also divided into four groups of n = 10 that received 0.5 mL of normal saline alone or normal saline containing EPO (1000 IU/kg) with periods equivalent to reperfusion of 24 h (sham-24 h+ V group and sham-24 h+ EPO) group, respectively) or 1 week (sham-1w +V group and sham-1w +EPO group, respectively).
Surgery and experimental protocol
Each rat was anesthetized deeply by ether and the abdominal region was shaved, soaked with betadine and covered with a sterile draper, leaving only its abdomen exposed for a midline electrosurgical laparotomy. In rats subjected to renal ischemia/reperfusion, there was occlusion of the right and left renal arteries and veins for 1 h in the BRI +V group by using a non-traumatic clamp, occlusion was verified visually by a color change of the kidney to a paler shade, and reperfusion was verified by a darkening.
In the sham +V group, all surgical procedures were performed but renal pedicles were only manipulated, and were maintained under anesthesia for the duration of the experiment. The abdominal incision was sutured at two layers by 2-0 silk. The whole surgical procedure was performed under sterile condition, and the rat was allowed to recover from the anesthesia prior returning to an individual cage. The surgical protocol was approved by the Shiraz University of Medical Sciences.
Treatment
The animals received saline (i.p.), or EPO (1,000 U/kg, single dose, (i.p) 30 min before surgery. EPO was provided as a gift from Pouyesh Darou product Company. The dose of EPO used was based on that found to be maximally effective in our previous published study (26).
Assessment of Renal Function
At the end of the experiments, 1 mL blood samples were collected from anesthetized rats 24h and 1w after the reperfusion period. Plasma concentrations of BUN (blood urea nitrogen) and creatinine (Cr) were used as a marker of renal function. The measurements were performed by a lab technician blind to the groups.
Behavioral assessments
Passive Avoidance (PA) Learning
This test was used to evaluate fear learning in AKI rats and the possible effect of EPO on 24 h and 1w after reperfusion. The apparatus was a shuttle-box device with dimensions of 100*25*25 which consisted of two compartments, one dark and one illuminated part separated by a guillotine door, and a stainless rod grid serving as the base. The adaptation phase was followed by a single trial in which the animals were placed in the lit arena, after 10s, the door was opened and the animal was allowed to go to the dark compartment. Then the door was closed without electric shock and after 20s, the animal was placed in the cage. If the rats did not enter the dark chamber within 60s, they were eliminated from the test and replaced with a new rat. After 30 min of adaptation, for the learning trial this procedure was repeated. A mild shock was immediately administered (0.5 mA, 1.5 s) to the rat the third time that the animal entered the dark sector. This procedure was repeated until the animal did not enter the dark box in a 60s period. The number of shocks received was recorded in this phase.
In the retention trials, 24h after training, the test was performed to evaluate memory; in this step the animal was placed in the light arena and the latency to step into the dark sector as retention time (step through latency (STL), Time in dark compartment (TDC) and number of entrance into dark sector were measured as indicators of contextual memory) was recorded to a maximum of 300s (27).
Morris Water Maze (MWM)
To assess spatial learning and memory, the animals were tested by a MWM as previously described (28). Briefly, the test chamber was a circular tank (140 cm diameter, 45 cm height, a black pool), surrounded by extra-tank visual cues. A visible or submerged platform (15 cm wide, 35 cm height) was placed 1.5 cm above or below the water surface. Water temperature was maintained among 21–23 °C.
Rats’ behavior was recorded with the Ethovision system. The following parameters were recorded for each rat: total distance and time spent to reach the platform in three consecutive trials, number of crosses in the correct quadrant in the retention phase, percentage of time, and distance traveled in the correct quadrant.
In the training phase, each rat underwent three blocks of trials, each comprising four trials (inter-trial interval = 30 s). In each trial, animals were placed in one of the four quadrants facing towards the maze. Each rat was given 60s to find the platform, and if they did not find it, they were put on the platform by the examiner. After 30 s, the rat was again put to the trial.
After 2 h of the last block, the rats underwent a probe trial during which the platform was removed from the tank and the number of crosses in the correct quadrant and total time spent in target quadrant was recorded and analyzed for each rat.
Histological Assessment
The rats were decapitated and their brains were removed 24 h and 1w after reperfusion. After tissue processing, paraffinzed brains were cut onto 6µm sections on a rotary microtome and the sections were stained with cresyl violet (Nissl staining) (29).
Molecular experiment
Tissue dissection and preparation for Western blot
For molecular experiment, the rats were allocated in sham +V, BRI +V, and BRI +EPO groups. All of the animals were anesthetized with atmosphere CO2 and decapitated 24 h after surgery. Their brains were rapidly extracted and placed on ice. The both whole hippocampi were freshly harvested and maintained in a microtube at −80 °C until homogenization for further western blot assay (30).
BDNF immunoblot analysis
Dissociated hippocampus tissues were homogenized in ice-cold buffer containing 10 mM Tris–HCl (pH 7.4), 1 mM EDTA, 0.1% SDS, 0.1% Na-deoxycholate, 1% NP-40 with protease inhibitors (1 mM phenylmethylsulfonyl fluoride, 2.5 μg/mL of leupeptin, 10 μg/mL of aprotinin) and 1 mM sodium orthovanadate (a phosphatase inhibitor). The homogenate was centrifuged at 15,000 rpm for 20 min at 4 °C. The resulting supernatant was retained as the whole cell fraction. Protein concentrations were measured using the Bradford method (Bio-Rad Laboratories, Muenchen, Germany). Equal amounts of protein were resolved electrophoretically on a 9% SDS polyacrylamide gel electrophoresis (SDS-PAGE) SDS-PAGE gel and transferred to PVDF (polyvinylidenefluoride) membranes. After blocking (overnight at 4 °C) with 5% non-fat dried milk in Tris-buffered saline with Tween 20 (blocking buffer, TBS-T, 150 mM NaCl, 20 mM Tris–HCl, pH 7.5, 0.1% Tween 20) for 2 h, at room temperature and then, the membranes were incubated overnight by a primary rabbit polyclonal antibody for BDNF (1:1000, sc-20981; Santa Cruz Biotechnology, Santa Cruz, USA) at 4 °C. After washing in TBS-T buffer (three times for 5 min each, at room temperature) the blots were incubated for 2 h at room temperature with an anti-rabbit IgG secondary antibody conjugated with horseradish peroxidase (1:15,000; GE Healthcare Bio-Sciences). Both primary and secondary antibodies were diluted in blocking buffer. The antibody-antigen complexes were revealed using the ECL system (Amersham Biosciences) and images were captured on a Gel Doc imaging system (Bio-Rad, Hercules, CA, USA), converted to a tiff file, and analyzed with Lab Work analyzing software (UVP, UK) was used to analyze the intensity of the expression. β-actin immunoblotting (antibody from Cell Signaling Technology, INC. Beverly, MA, USA; 1:1000) was used to control for loading (30).
Statistical Analysis
Statistical procedures were performed using the SPSS software (version 18). ANOVA followed by Tukey’s post-hoc analysis was used to compare the differences between groups. The PA learning and probe data of MWM were analyzed by one-way ANOVA. Repeated measures ANOVA was used to analyze the data of MWM task in the learning phase.
The band density values were expressed as BDNF/β-actin ratio for each sample. The averages for different groups were compared using one-way ANOVA. All data were expressed as mean ± SEM. P <0.05 was considered statistically significant.
Results
The effect of BRI and EPO administration on plasma variables
As demonstrated in Table 1A, 24 h after reperfusion, plasma concentrations of creatinine and urea nitrogen were significantly increased in BRI +V group compared to the other groups (P < 0.001). Administration of EPO counteracted these effects of BRI and reduced these plasma concentrations (P < 0.001).
Also in table 1B plasma concentrations of creatinine and urea nitrogen has been showed 1w after operation. Urea nitrogen was significantly increased in BRI +V group (P < 0.05 versus sham +V) and administration of EPO reduced that (P < 0.01), but there are no significant difference in creatinine level between the groups (P > 0.05).
The effect of BRI and EPO administration on PA learning 24 h after reperfusion
There was no significant difference in the number of shocks received among the four groups (P > 0.05) (Figure 1a).
RI +V rats had impairment in memory retrieval compared to the other three groups (P < 0.01, ANOVA); administration of EPO reversed this impairment (Figure.1b).
The time in the dark compartment (TDC) was altered in the BRI +V group compared to the BRI + EPO group (P< 0.05, ANOVA). EPO counteracted this effect of BRI on PA learning (Figure 1c).
The effect of BRI and EPO administration on plasma variables 24 h after surgery.
Variables | Plasma creatinine | Plasma urea nitrogen |
---|---|---|
Groups | ||
Sham + V | 0.68 ± 0.03 | 37.8 ± 3.5 |
Sham + EPO | 0.71 ± 0.04 | 38 ± 3.3 |
BRI + V | 1.5 ± 0.19*** ††† | 192.1 ± 22.06*** ††† |
BRI + EPO | 0.67 ± 0.04 | 42.4 ± 1.7 |
The effect of BRI and EPO administration on plasma variables 1 week after surgery.
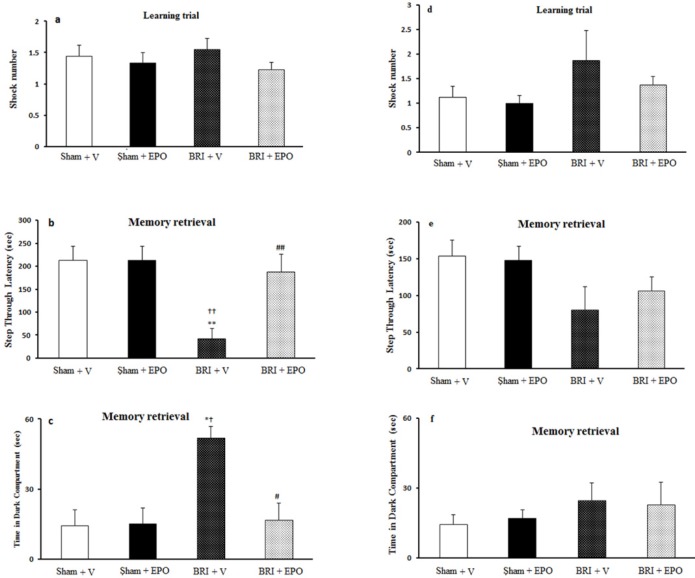
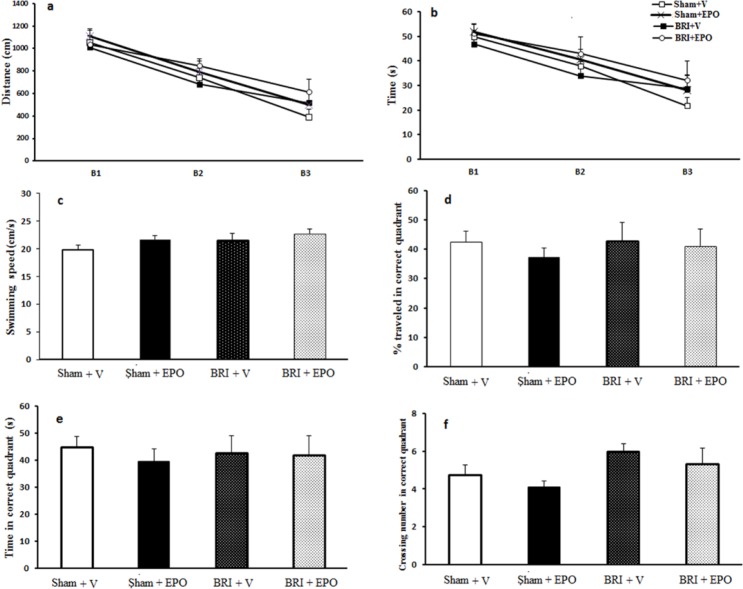
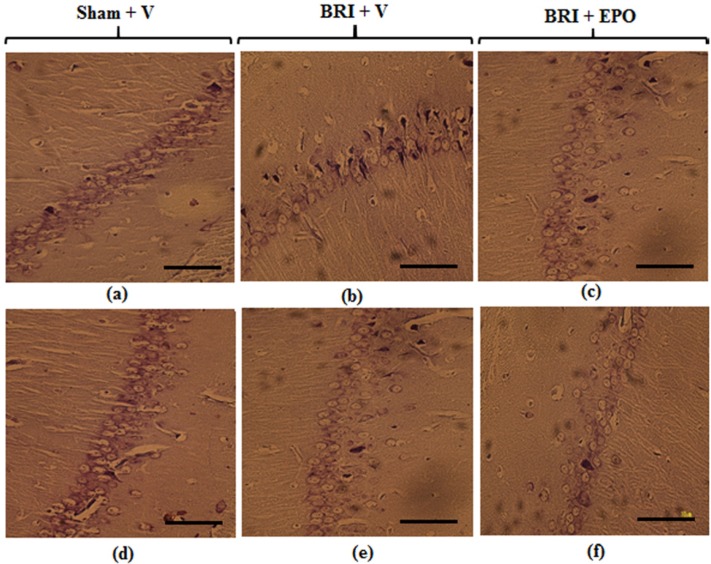
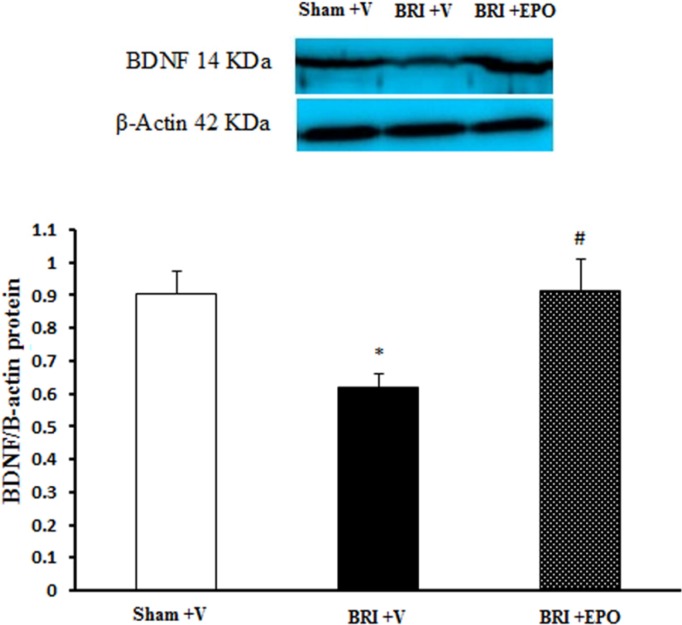
The Effect of BRI + V and EPO administration on PA learning 1 week after reperfusion
No significant alteration in the parameters measured was observed amongst the four groups of study 1 week after reperfusion (P > 0.05) (Figure 1d_f).
The effect of BRI and EPO administration on spatial learning
Figure 2a and b shows the results of the learning trial. Repeated measures of analysis of variance showed a reduction in escape latencies at the block effect in all rats and the swimming distance to locate the platform across blocks of trials, indicating spatial acquisition (block 3 compared to block 1 for all groups, at least P < 0.05). All treated rats, sham + V, and the BRI + V groups progressively took less time to locate the hidden platform over the course of the 12 trials during the training ----period. Spatial learning in the MWM task was not altered in BRI + V rats compared to sham +V, sham + EPO or BRI + EPO rats (P > 0.05, (Figure 2a and b). There were no significant differences in the swimming speeds (Figure 2c) between groups in all periods, indicating that the swimming speed did not have influence in the latencies.
There was no significant difference observed in the probe trial parameters (Figure 2d_f) measured among the four groups of study.
Histological evaluation
As Figure 3 (a-c) demonstrates 24 h after reperfusion: Light microscopic study of CA1 region of hippocampus sections showed normal morphology in pyramidal cells of sham + V group. Histologic samples of sham + EPO group also did not show any morphologic changes compared to sham (data have not been shown).
The CA1 of hippocampus sections in BRI +V group showed degenerative changes including shrunken nuclei and dark cytoplasm. Meanwhile, EPO could protect neurons from morphological alterations 24 h after induction of BRI and attenuated degenerative changes in pyramidal neurons of hippocampus region.
One week after reperfusion: Normal morphology of pyramidal neurons was observed in CA1 region of hippocampus in sham + V group. No significant degenerative changes were detected in BRI + V and BRI + EPO groups (Figure 3 d-f).
The effect of BRI and EPO administration on BDNF protein expression
Then, we examined whether there is a potential molecular mechanism that underlies the memory impairments. We evaluated the expression of BDNF 24 h after reperfusion, because the BRI -induced memory dysfunction was observed just in this time point. Since there was no significant difference between sham and sham + EPO groups in the behavioral data, the sham + EPO group did not assess in the molecular experiment.
As shown in Figure 4 the lowest level of BDNF protein (0.61 ± 0.03) was detected in the hippocampus of BRI + V rats and statistically significant difference was observed as compared to sham + V (0.9 ± 0.06; P < 0.05) and BRI + EPO rats (0.91 ± 0.09; P < 0.05) groups (Figure. 4). The current data showed that significant decrease in BDNF protein expression in BRI + V group was inhibited by administration of EPO.
Discussion
The goals of this study were to assess 1) the possible learning and memory disabilities associating with BRI (as an animal model of AKI) and 2) the possible neuroprotection effect of EPO on brain dysfunction induced by BRI in two different time points: short-term (24 h after reperfusion) and long-term (1w after reperfusion).
Our results demonstrated that BRI leads to learning and memory impairments 24 h after reperfusion. Also, we observed no significant memory disabilities in BRI + V group compared to the other groups 1w after operation.
Most of the mortality caused by AKI is due to extrarenal organ dysfunction. Experimental evidence has established that AKI leads to brain inflammation (10, 31). Liu et al observed that AKI led to both soluble and cellular inflammation in the brain, with the hippocampus being the main target (10). Also, they found that mice with AKI showed striking cellular abnormalities, microgliosis and increased neuronal pyknosis in the hippocampus (10). On account of the high sensitivity of hippocampus in response to AKI, cognitive dysfunctions can be proposed following AKI.
Additionally, researchers demonstrated a graded relation between estimated glomerular filtration rate level (24) and cognitive function (32-34). In patients undergoing dialysis, cognitive impairments are seen more frequently (7, 8). When the disease progresses, disorientation, defects in attention span and memory becomes manifest (35).
Pharmacological correction of brain dysfunction associated with AKI is clinically important, which is a reason for studying ischemia and the identifying new treatment strategies affording neuroprotection. In the current study, EPO showed a neuroprotective function against learning and memory deficits in ischemic rats 24 h after reperfusion.
EPO is a glycopeptide that not only plays a key role in erythrocyte production stimulation in the bone marrow, but also known as a neuroprotective agent offers potential (36). EPO has multiple protective effects, such as antioxidant, anti-inflammatory, angiogenic and antiapoptotic effects (37). Since the significant effects of AKI on brain pathologies has become increasingly clear which involves inflammation; therefore, it seems that EPO may exert neuroprotective effects against BRI -induced impairments.
The data of the present study clearly demonstrate that BRI (as an animal model of AKI) leads to PA learning impairment 24 after reperfusion. EPO treated rats had STL and also TDC similar to the sham +V group. Therefore, EPO showed a promising effect against learning and memory impairments induced by BRI 24 h after reperfusion. Passive avoidance test also was performed 1 week after reperfusion and data obtained with this test indicated no significant differences between measured parameters between the groups. Our findings are consistent with previous studies reporting cognitive impairments in renal diseases (as mentioned above).
In the current study, the spatial memory was evaluated using a Morris water maze 1 week after reperfusion and data indicated no significant differences in memory amongst the groups.
As mentioned above, most notably, we discovered that 1 week after BRI, there are no significant cognitive deficits. There are some potential explanations for these findings. First, we could argue through the comparison of plasma variables 24 h and 1w after reperfusion. Our data clearly demonstrate that 60 min bilateral renal ischemia reperfusion caused significant increase in the plasma concentrations of both BUN and Cr 24 h after ischemia, indicating a significant level of renal dysfunction. One week after ischemia, although BUN level is significantly more than the other groups but there is no significant difference in plasma concentration of creatinine between experimental groups. Since the change in creatinine is clinically and pathologically an important indicator of AKI, it can be suggested that the renal function recovered to some extent 1 week after BRI and it is likely the factors involved in the pathogenesis of the kidney tissue injury during I/R reduced.
Second, in this study, histological results also demonstrated that 24 h after reperfusion CA1 of hippocampus sections in BRI + V group showed severe injury and EPO could protect against renal ischemia and decreased degeneration compared to BRI + V group. CA1 region is related with memory and deficits of these neurons leads to behavioral dysfunctions assessed by laboratory tests.
In contrast to this, one week after reperfusion no significant degenerative changes were observed in CA1 region of hippocampus sections in all experimental groups.
Shid Moosavi et al have previously indicated that 24 h of unilateral ureteral obstruction leads to oxidative stress in the obstructed kidney through not only enhanced production of reactive oxygen species (ROS) but also decreased ability of antioxidant defense system (38). Third, in the light of these findings, since oxidative stress observed at early hours after release of obstruction, we can explain severe damages at 24 h compared to 1w. Our results showed that EPO as an antioxidant agent could prevent impairments has been evented at first 24 h after BRI.
The protective effect of EPO on renal and cerebral ischemia, separately has been shown in various studies (39-42). For instance, Tazangi et al reported that the treatment with EPO can improve the memory loss and synaptic plasticity defect in the rat model of Alzheimer’s disease (43). Also the recovery of spatial memory in brain-lesioned animals following EPO administration has been observed (44). EPO can improve nerve function by the modulation of transient neural plasticity mechanisms (44).
In the current study molecular assay was performed at 24 h after reperfusion (because we did not observe learning and memory impairment 1w after reperfusion, therefore did not examine in this time point). The results of our molecular assay indicated that EPO administration improved decrease in BDNF protein level in hippocampus region which was induced by BRI 24 h after reperfusion. Several lines of evidences demonstrated contributions of BDNF in cognitive functions, particularly in memory acquisition and consolidation (24, 45, 46). The present study for the first time provides clear evidences that a single dose of EPO administration 30 min before renal ischemia can confer marked behavioral, histological and molecular protection 24 h after reperfusion. Protection induced by EPO may be mediated by several mechanisms: 1) EPO treatment can increase generation of neurotrophic factors and induce long term changes in cognition (39). 2) EPO can reduce lipid peroxidation and support defense systems which are important insults after renal ischemia (47, 48). 3) It has been previously shown that impairments in the cholinergic pathways of the brain may be responsible for the cognitive deficits (49).
EPO leads to increased cholinergic function and as its result, improvement of cognitive deficits (50, 51). We just summarize some possible mechanisms of EPO but further studies are needed to clarify the exact mechanisms of EPO neuroprotection in a BRI model of cognitive impairments.
In conclusions, results from our experiments showed that EPO when administered as a single dose 30 min before BRI, improved deficits 24 h after reperfusion. One of the potential mechanisms involved in this protection may be through increase in BDNF protein expression. Also, we observed that there are no significant behavioral abnormalities in BRI group 1w after ischemia.
Acknowledgements
References
-
1.
Seifi B, Kadkhodaee M, Najafi A, Mahmoudi A. Protection of liver as a remote organ after renal ischemia-reperfusion injury by renal ischemic postconditioning. Int. J. Nephrol. 2014;2014:120391. [PubMed ID: 24744919].
-
2.
Legrand M, Mik EG, Johannes T, Payen D Ince C. Renal hypoxia and dysoxia after reperfusion of the ischemic kidney. Mol. Med. 2008;4:502-16.
-
3.
Hashemi M. The Study of Petoxifylline Drug Effects on Renal Apoptosis and Bcl2 Gene Expression Changes Following Ischemic Reperfusion Injury in Rat. Iran. J. Pharma. Res. 2014;13:181-9.
-
4.
Grams ME, Rabb H. The distant organ effects of acute kidney injury. Kidney Int. 2012;81:942-8. [PubMed ID: 21814177].
-
5.
Di-Pietro PB, Dias ML, Scaini G, Burigo M, Constantino L, Machado RA, Dal-Pizzol F, Streck EL. Inhibition of brain creatine kinase activity after renal ischemia is attenuated by N-acetylcysteine and deferoxamine administration. Neurosci. Lett. 2008;434:139-43. [PubMed ID: 18304734].
-
6.
Nongnuch A, Panorchan K, Davenport A. Brain–kidney crosstalk. Crit. Care. 2014;18:1.
-
7.
Murray AM. Cognitive impairment in the aging dialysis and chronic kidney disease populations: an occult burden. Adv. Chronic Kidney Dis. 2008;15:123-32. [PubMed ID: 18334236].
-
8.
Murray A, Tupper D, Knopman DS, Gilbertson D, Pederson S, Li S, Smith GE, Hochhalter AK, Collins AJ, Kane RL. Cognitive impairment in hemodialysis patients is common. Neurology. 2006;67:216-23. [PubMed ID: 16864811].
-
9.
Adachi N, Lei B, Deshpande G, Seyfried FJ, Shimizu I, Nagaro T, Arai T. Uraemia suppresses central dopaminergic metabolism and impairs motor activity in rats. Intensive Care Med. 2001;27:1655-60. [PubMed ID: 11685308].
-
10.
Liu M, Liang Y, Chigurupati S, Lathia JD, Pletnikov M, Sun Z, Crow M, Ross C, Mattson MP, Rabb H. Acute kidney injury leads to inflammation and functional changes in the brain. J. Am. Soc. Nephrol. 2008;19:1360-70. [PubMed ID: 18385426].
-
11.
Belayev L, Khoutorova L, Zhao W, Vigdorchik A, Belayev A, Busto R, Magal E, Ginsberg MD. Neuroprotective effect of darbepoetin alfa, a novel recombinant erythropoietic protein, in focal cerebral ischemia in rats. Stroke. 2005;36:1071-6. [PubMed ID: 15790949].
-
12.
Johnson DW, Pat B, Vesey DA, Guan Z, Endre Z, Gobe GC. Delayed administration of darbepoetin or erythropoietin protects against ischemic acute renal injury and failure. Kidney Int. 2006;69:1806-13. [PubMed ID: 16598197].
-
13.
Rezaee MA, Moallem SA, Imenshahidi M, Farzadnia M, Mohammadpour AH. Effects of erythropoietin on electrocardiogram changes in carbon monoxide poisoning: an experimental study in rats. Iran. J. Pharm. Res. 2012;11:1191-9. [PubMed ID: 24250553].
-
14.
Li Y, Juul SE, Morris-Wiman JA, Calhoun DA, Christensen RD. Erythropoietin receptors are expressed in the central nervous system of mid-trimester human fetuses. Pediatr. Res. 1996;40:376-80. [PubMed ID: 8865271].
-
15.
Marti HH, Wenger RH, Rivas LA, Straumann U, Digicaylioglu M, Henn V, Yonekawa Y, Bauer C, Gassmann M. Erythropoietin gene expression in human, monkey and murine brain. Eur. J. Neurosci. 1996;8:666-76. [PubMed ID: 9081618].
-
16.
Celik M, Gökmen N, Erbayraktar S, Akhisaroglu M, Konakç S, Ulukus C, Genc S, Genc K, Sagiroglu E, Cerami A, Brines M. Erythropoietin prevents motor neuron apoptosis and neurologic disability in experimental spinal cord ischemic injury. Proc. Natl. Acad. Sci. 2002;99:2258-63. [PubMed ID: 11854521].
-
17.
Grasso G, Sfacteria A, Erbayraktar S, Passalacqua M, Meli F, Gokmen N, Yilmaz O, Torre DL, Buemi M, Iacopino DG, Coleman T, Cerami A, Brines M, Tomasello F. Amelioration of spinal cord compressive injury by pharmacological preconditioning with erythropoietin and a nonerythropoietic erythropoietin derivative. J. Neurosurg. Spine. 2006;4:310-8. [PubMed ID: 16619678].
-
18.
Grasso G, Sfacteria A, Passalacqua M, Morabito A, Buemi M, Macrì B, Brines ML, Tomasello F. Erythropoietin and erythropoietin receptor expression after experimental spinal cord injury encourages therapy by exogenous erythropoietin. Neurosurgery. 2005;56:821-7. [PubMed ID: 15792521].
-
19.
Wang L, Zhang Z, Wang Y, Zhang R, Chopp M. Treatment of stroke with erythropoietin enhances neurogenesis and angiogenesis and improves neurological function in rats. Stroke. 2004;35:1732-7. [PubMed ID: 15178821].
-
20.
Gonzalez FF, McQuillen P, Mu D, Chang Y, Wendland M, Vexler Z, Ferriero DM. Erythropoietin enhances long-term neuroprotection and neurogenesis in neonatal stroke. Dev. Neurosci. 2007;29:321-30. [PubMed ID: 17762200].
-
21.
Sakanaka M, Wen T-C, Matsuda S, Masuda S, Morishita E, Nagao M, Sasaki R. In-vivo evidence that erythropoietin protects neurons from ischemic damage. Proc. Nat. Acad. Sci. 1998;95:4635-40. [PubMed ID: 9539790].
-
22.
Cerami A. Beyond erythropoiesis: novel applications for recombinant human erythropoietin. Seminars in hematology. Elsevier; 2001.
-
23.
Nagahara AH, Merrill DA, Coppola G, Tsukada S, Schroeder BE, Shaked GM, Wang L, Blesch A, Kim A, Conner JM, Rockenstein E, Chao MV, Koo EH, Geschwind D, Masliah E, Chiba AA, Tuszynski MH. Neuroprotective effects of brain-derived neurotrophic factor in rodent and primate models of Alzheimer’s disease. Nat. Med. 2009;15:331-7. [PubMed ID: 19198615].
-
24.
Kiprianova I, Sandkühler J, Schwab S, Hoyer S, Spranger M. Brain-derived neurotrophic factor improves long-term potentiation and cognitive functions after transient forebrain ischemia in the rat. Exp. Neurol. 1999;159:511-9. [PubMed ID: 10506522].
-
25.
Griesbach GS, Hovda DA, Gomez-Pinilla F. Exercise-induced improvement in cognitive performance after traumatic brain injury in rats is dependent on BDNF activation. Brain Res. 2009;1288:105-15. [PubMed ID: 19555673].
-
26.
Tahamtan M, Moosavi S, Sheibani V, Nayebpour M, Esmaeili-Mahani S, Shabani M. Erythropoietin attenuates motor impairments induced by bilateral renal ischemia/reperfusion in rats. Fundam. Clin. Pharmacol. 2016.
-
27.
Tahamtan M, Allahtavakoli M, Abbasnejad M, Roohbakhsh A, Taghipour Z, Taghavi M, Khodadadi H, Shamsizadeh A. Exercise preconditioning improves behavioral functions following transient cerebral ischemia induced by 4-vessel occlusion (4-VO) in rats. Arch. Iran. Med. (AIM). 2013;16:697-704.
-
28.
Aghaei I, Shabani M, Doustar N, Nazeri M, Dehpour A. Peroxisome proliferator-activated receptor-γ activation attenuates motor and cognition impairments induced by bile duct ligation in a rat model of hepatic cirrhosis. Pharmacol. Biochem. Behav. 2014;120:133-9. [PubMed ID: 24631483].
-
29.
Gittins R, Harrison PJ. Neuronal density, size and shape in the human anterior cingulate cortex: a comparison of Nissl and NeuN staining. Brain Res. Bulletin. 2004;63:155-60.
-
30.
Hajali V, Sheibani V, Ghazvini H, Ghadiri T, Valizadeh T, Saadati H, Shabani M. Effect of castration on the susceptibility of male rats to the sleep deprivation-induced impairment of behavioral and synaptic plasticity. Neurobiol. Learn Mem. 2015;123:140-8. [PubMed ID: 26079215].
-
31.
Yap SC, Lee HT. Acute kidney injury and extrarenal organ dysfunction: new concepts and experimental evidence. Anesthesiology. 2012;116:1139-48. [PubMed ID: 22415388].
-
32.
Kurella M, Chertow GM, Luan J, Yaffe K. Cognitive impairment in chronic kidney disease. J. Am. Geriat. Soc. 2004;52:1863-9. [PubMed ID: 15507063].
-
33.
Kurella M, Yaffe K, Shlipak MG, Wenger NK, Chertow GM. Chronic kidney disease and cognitive impairment in menopausal women. Am. J. Kidney Diseases. 2005;45:66-76. [PubMed ID: 15696445].
-
34.
Hailpern SM, Melamed ML, Cohen HW, Hostetter TH. Moderate chronic kidney disease and cognitive function in adults 20 to 59 years of age: Third National Health and Nutrition Examination Survey (NHANES III). J. Am. Soc. Nephrol. 2007;18:2205-13. [PubMed ID: 17554148].
-
35.
Tyler HR. Neurologic disorders in renal failure. Am. J. Med. 1968;44:734-48. [PubMed ID: 4296458].
-
36.
Felipo V. Hepatic encephalopathy: effects of liver failure on brain function. Nature Rev. Neurosci. 2013;14:851-8. [PubMed ID: 24149188].
-
37.
Ebert BL, Bunn HF. Regulation of the erythropoietin gene. Blood. 1999;94:1864-77. [PubMed ID: 10477715].
-
38.
Moosavi SMS, Ashtiyani SC, Hosseinkhani S, Shirazi M. Comparison of the effects of l-carnitine and α-tocopherol on acute ureteral obstruction-induced renal oxidative imbalance and altered energy metabolism in rats. Urol. Res. 2010;38:187-94. [PubMed ID: 19940986].
-
39.
Kumral A, Uysal N, Tugyan K, Sonmez A, Yilmaz O, Gokmen N, Kiray M, Gencf S, Duman N, FKoroglu T, Ozkan H, Genc K. Erythropoietin improves long-term spatial memory deficits and brain injury following neonatal hypoxia–ischemia in rats. Behav. Brain Res. 2004;153:77-86. [PubMed ID: 15219709].
-
40.
Catania MA, Marciano MC, Parisi A, Sturiale A, Buemi M, Grasso G, Squadrito F, Caputi AP, Calapai G. Erythropoietin prevents cognition impairment induced by transient brain ischemia in gerbils. Eur. J. Pharmacoy. 2002;437:147-50.
-
41.
Patel NS, Sharples EJ, Cuzzocrea S, Chatterjee PK, Britti D, Yaqoob MM, Thiemermann C. Pretreatment with EPO reduces the injury and dysfunction caused by ischemia/reperfusion in the mouse kidney in-vivo. Kidney Int. 2004;66:983-9. [PubMed ID: 15327391].
-
42.
Johnson D, Pat B, Vesey D, Guan Z, Endre Z, Gobe G. Delayed administration of darbepoetin or erythropoietin protects against ischemic acute renal injury and failure. Kidney Int. 2006;69:1806-13. [PubMed ID: 16598197].
-
43.
Tazangi PE, Moosavi SMS, Shabani M, Haghani M. Erythropoietin improves synaptic plasticity and memory deficits by decrease of the neurotransmitter release probability in the rat model of Alzheimerʹs disease. Pharmacol. Biochem. Behav. 2015;130:15-21. [PubMed ID: 25553822].
-
44.
Almaguer-Melian W, Mercerón-Martínez D, Delgado-Ocaña S, Pavón-Fuentes N, Ledón N, Bergado JA. EPO induces changes in synaptic transmission and plasticity in the dentate gyrus of rats. Synapse. 2016;70:240-52. [PubMed ID: 26860222].
-
45.
Hariri AR, Goldberg TE, Mattay VS, Kolachana BS, Callicott JH, Egan MF, Weinberger DR. Brain-derived neurotrophic factor val66met polymorphism affects human memory-related hippocampal activity and predicts memory performance. J. Neurosci. 2003;23:6690-4. [PubMed ID: 12890761].
-
46.
Bechara RG, Lyne R, Kelly ÁM. BDNF-stimulated intracellular signalling mechanisms underlie exercise-induced improvement in spatial memory in the male Wistar rat. Behav. Brain Res. 2014;275:297-306. [PubMed ID: 24269499].
-
47.
Vannucci RC. Hypoxic-ischemic encephalopathy. Am.J. Perinatol. 1999;17:113-20.
-
48.
Berger R, Garnier Y. Pathophysiology of perinatal brain damage. Brain Res. Rev. 1999;30:107-34. [PubMed ID: 10525170].
-
49.
Wang LS, Zhou J, Shao XM, Tang XC. Huperzine A attenuates cognitive deficits and brain injury in neonatal rats after hypoxia–ischemia. Brain Resh. 2002;949:162-70.
-
50.
Tabira T, Konishi Y, Gallyas F. Neurotrophic effect of hematopoietic cytokines on cholinergic and other neurons in-vitro. Int. J. Dev. Neurosci. 1995;13:241-52. [PubMed ID: 7572278].
-
51.
Konishi Y, Chui D-H, Hirose H, Kunishita T, Tabira T. Trophic effect of erythropoietin and other hematopoietic factors on central cholinergic neurons in-vitro and in -vivo. Brain Res. 1993;609:29-35. [PubMed ID: 7685231].