Abstract
Keywords
Introduction
Cancer is unusual cell growth and division which can spread to different parts of body. Chemotherapy is a main approach for cancer treatment and limited by low selectivity of chemotherapy drugs for normal tissues, especially tissues with rapidly cells division and growth.
Angiogenesis is crucial and essential factor in the invasive growing and proliferation of cancer cells. It is the process of formation of new blood vessel from pre-exciting vessel which includes the migration, growth, and differentiation of endothelial cells, inside the walls of blood vessel (1). Integrin as a cell surface and adhesion receptor interacts to ECM proteins. They are heterodimer glycoprotein receptors and have 24 pairs α and 8 β subunits and have key roles in cell growing, proliferation, migration, differentiation and cell survival signaling (2). αv integrin is highly overexpressed on activated endothelial of cancer cells and tumor cells, but it is not expressed in resting endothelial cells and most normal organ systems, making it a potential target for antiangiogenic strategy in cancer therapy (3).
Integrins are ideal pharmacological targets based on their key role in angiogenesis and tumor development and on their easy accessibility as cell surface receptors interacting with extracellular ligands. αvβ3 was the first vascular integrin, targeted to suppress tumor and ischemia-induced angiogenesis. Antagonists of integrin αvβ3 are currently tested in clinical trials as antiangiogenic gents (4). On the other hand NSAIDs have shown significant anti-proliferative effects through the inhibition of COX-2 (5, 6). It is also proven that the anti-proliferative activity of NSAIDs is via anti-angiogenesis activity of these drugs (7, 8) and inhibition of COX-2 by NSAIDs have suppressed tumor growth in animal models and reduced the risk of developing cancer in humans (6, 9 and 10). Moreover NSAIDs exert some of their anti-thrombotic and anti-inflammatory effects by interfering with integrin function. For example, indomethacin and aspirin inhibit platelet aggregation by suppressing activation of integrin αvβ3, the main fibrinogen receptor in platelets (11). Addition, several other reports indicate that NSIADs inhibit αvβ3 integrin, which affects the endothelial-cell spreading, migration and angiogenesis processes (12). In light of the evidences which support the role of NSAIDs in cancer cells suppression and considering the overexpression of COX-2 enzyme in cancer cells (6, 9 and 10), targeted delivery of these therapeutic agents to the cancerous cells could maximize their efficiency and minimize their side effects. Nemours studies in tumor targeting have been implemented by using certain peptide sequences which are conjugated to the drug of interest and this strategy has been recently introduced as one of the useful strategies in medicinal chemistry of chemotherapeutic agents.
The two tripeptidic sequences, RGD (Arginine-Glycine-Aspartic acid) and NGR (Asparagine-Glycine-Arginine) motifs have been recognized as targeting tools based on phage display studies (13). RGD is a well-known peptide sequence for targeting integrin receptors and can bind to avb3 and avb5 integrin receptors subunits which are overexpressed during the angiogenesis process of cancer cells (14). Since αv integrins are overexpressed on surface of cancer cells (15-19), RGD as an integrin ligand can be used as a targeting system for delivering chemotherapeutic agents to the cancer cells (3). For example, RGD has receptor on activated platelet; Shaoming Jin has used aspirin conjugated with RGDV for delivery of Aspirin to thrombus (20). NGR peptide has also been used as targeting tool for aminopeptidase N (CD13) which is overexpressed in cancer and inflammatory diseases,
Ketoprofen is a nonsteroidal anti-inflammatory drug (NSAID) which inhibits COX-1 and COX-2 enzymes. Numerous studies demonstrate that NSAIDs have anticancer properties and reduced the risk of cancers like colon and liver (21) Several structural modifications have been made in NSAIDs to change their properties such as lipophilicity, solubility in water and side effects (22-24). Cheng et al. have demonstrated that ketoprofen inhibits human colon tumor cell N-acetyltransferase (NAT) activity, gene expression and DNA adduct formation for the first time in 2006 (25). Marjanovic and his coworkers have also reported potential antitumor activity for the amide derivatives of ketoprofen and fenoprofen later in 2007 (26). Based on the above mentioned fact, we decided to prepare RGD and NGR conjugates of ketoprofen and evaluate their anti-cancer activity on a selected group of cancer cell lines. The conjugated forms are expected to be delivered rather selectively to tumor cell lines due to the overexpression of RGD and NGR receptors on the surface of these cells. In order to investigate the impact of possible steric hindrance due to the attachment of the drug to the peptide, a linear six carbon (hexanoic acid) linker was also used as a spacer.
Experimental
Materials and methods
All chemicals and solvents were purchased from Merck (Darmstadt, Germany). Na-9-Fluorenylmethoxycarbonyl (Fmoc)-protected amino acids, Rink amid resin, 1-[Bis(dimethylamino)methylene]-1H- 1,2,3-triazolo[4,5-b] pyridinium 3-oxid hexafluorophosphate (HATU) were purchased from GL Biochem Company (Shanghi) Ltd. 1HNMR spectra were recorded on a 500 MHz Bruker spectrometer. ESI-MS spectra were obtained by Agilent 6410 Triple Quad LC/MS. IR spectra were recorded on a PerkinElmer IR spectrophotometer as potassium bromide discs. The purity of compounds was confirmed by thin layer chromatography using Whatman Sil
G/UV254 silica gel plates as the stationary phase and with suitable mobile phase with fluorescent indicator, and the spots were visualized under 254 and 366 nm illumination. All products was purified by reverse phase HPLC on a Knaur chromatography system equipped with a Waters Prep Nova-Pak® HR ODS column (250 × 4.6 mm; porosity 5 μm).
General procedure for synthesis RGD conjugated with ketoprofen
Rink amide resin (0.5 g) was placed in a reactor and suspended in DMF under nitrogen atmosphere for 15 min. The fmoc group was removed with 20% piperidine in DMF (3 × 5 mL). After washing with DMF, coupling reaction was conducted. For each amino acid coupling: 2eq AA, 2eq HATU, 4eq N,N-diisopropylethylamine (DIEA), 15 mL DMF was mixed and added to the resin. The mixture was purged with N2 gas for 20 min. The completion of each coupling reaction and fmoc deprotection was tested by Kaiser Test which is the reaction of ninhydrin with amines.
After the last amino acid coupling reaction and removal of fmoc protecting group, the ketoprofen was coupled to the free amino group of the peptide, using the same standard procedure which is used for the coupling of amino acids. Finally resin washed and dried then linear peptide cleaved with trifluoroacetic acid (TFA) cocktail: TFA: Thioanisol: p-cresol: triisopropylsilane (TIS) (84%: 12%: 2%: 2%), during 2 h at room temperature. The reaction mixture was filtered and cold Diethyl Ether (50 mL) was added to the filtrate. The precipitate thus obtained was separated using centrifugation.
RGD
The title compound (50 mg) was obtained as a white solid with 63% yield at 97% purity (based on chromatography, mobile phase 30% CH3CN in H2O+ 0.1% TFA); 1H NMR (500 MHz, DMSO-d6) δ: 1.54 (s, 2 H, CH2), 1.70 (s, 2H, CH2), 2.63-2.69 (m, 2H, CH2), 3.11 (s, 2H, CH2), 3.86 (s, 3H, CH2, CH), 4.50-4.53 (quartet, J = 0.01, 1H, CH), 7.15-8.68 ppm (10H, NH, NH2); IR (KBr): υ (cm-1) = 1656, 3330; LC-MS (ESI) m/z (M + H)+ calcd for C12H23N7O5: 346.0, found: 346.1.
NGR
The title compound (57 mg) was obtained as a white solid with 64% yield at 93% purity (based on chromatography, mobile phase 30% CH3CN in H2O+ 0.1% TFA); 1H NMR (500 MHz, CDCl3) δ: 1.50 (s, 2 H, CH2), 1.99 (s, 2H, CH2), 2.5 (s, 1H, NH), 2.58-2.69 (m, 2H, CH2), 3.23 (s, 2H, CH2), 4.03 (s, 2H, CH2), 7.15-8.3 ppm (10H, NH, NH2); IR (KBr): υ (cm-1) = 1656, 3330; LC-MS (ESI) m/z (M + H)+ calcd for C12H23N7O5: 346.0, found: 346.1.
Keto-RGD
The title compound (83 mg) was obtained as a white solid with yield 65%, 98% purity (based on chromatography, mobile phase 30% CH3CN in H2O+ 0.1% TFA); 1H NMR (500 MHz, DMSO-d6) δ: 1.32-1.33(d, J = 0.01, 3H, CH3), 1.45-1.46 (m, 2H, CH2), 1.64-1.82 (m, 2H, CH2), 2.5 (s, 1H, NH), 2.96,3.07 (m, 2H, CH2), 3.64-3.69 (m, 2H,CH2), 3.82-3.85 (m, 1H, CH), 4.15-4.16 (m, 2H, CH2), 4.26-4.27 (m, 1H, CH), 4.38-4.43 (m, 1H,CH1), 7.05-8.31(15H, NH, NH2 and aromatic hydrogen), 11.8 (br s, 1H, OH) ppm; IR (KBr): υ (cm-1) 1656, 3330 ; LC-MS (ESI) m/z (M + H)+ calcd for C28H35N7O7: 581, found: 582.2.
Keto-NH-(CH2)4-CO-RGD
The title compound (103 mg) was obtained as a white solid with yield 65%, 98% purity (based on chromatography, mobile phase 30% CH3CN in H2O+ 0.1% TFA); 1H NMR (500 MHz, CDCl3) δ: 1.05 (s, 3H, CH3), 1.20-1.23 (m, 2H, CH2), 1.28 (s, 2H, CH2), 1.48 (m, 4H, CH2), 1.7 (m, 2H, CH2), 2.07 (m ,2H, CH2), 2.8 (br s, 2H, CH2), 3.17 (m, 2H, CH2), 3.38 (m, 2H, CH2), 3.46-3.52 (q, J = 0.02, 1H, CH), 4.16 (s, 2H, CH2), 4.55 (s, H, CH), 5.88-5.93 (m, H, CH), 7.00-7.8 ppm (18H, NH, NH2 and aromatic hydrogen), IR (KBr): υ (cm-1) 1654, 3356 ; LC-MS (ESI) m/z (M + H)+ calcd for C34H47N9O7: 693, found: 694.2.
Keto-NGR
The title compound (73 mg) was obtained as a white solid with yield 57%, 98% purity (based on chromatography, mobile phase 30% CH3CN in H2O+ 0.1% TFA); 1H NMR (500 MHz, CDCl3) δ: 1.05 (s, 3H, CH3), 1.25 (m, 2H, CH2), 1.45 (m, 2H, CH2), 2.5 (s, 1H, NH), 2. 6 (m, 2H, CH2), 3.47 (m, 2H,CH2), 3.6 (m, 1H, CH), 3.9 (m, 2H, CH2), 4.16 (m, 1H, CH), 4.43 (m, 1H,CH), 7.05-8.29 (19H, NH, NH2 and aromatic hydrogen), 11.8 (br s, 1H, OH) ppm; IR (KBr): υ (cm-1) 1656, 3330; LC-MS (ESI) m/z (M + H)+ calcd for C28H36N8O7: 581, found: 582.2.
Keto-NH-(CH2)4-CO-NGR
The title compound (110 mg) was obtained as a white solid with yield 69%, 97% purity (based on chromatography, mobile phase 30% CH3CN in H2O+ 0.1% TFA); 1H NMR (500 MHz, CDCl3) δ: 1.05 (d, 3H, CH3), 1.19-1.25 (m, 6H, 3CH2), 1.46-1.48 (m, 2H, CH2), 1.64 (m, 2H, CH2), 2.28 (br s, 2H, CH2), 2.49 (s, 1H , NH), 2.90 (br s, 2H, CH2), 2.97 (s, 2H, CH2), 3.40 (br s, 2H, CH2), 3.46-3.51(q, H, CH), 4.12 (s, 2H, CH2), 4.8 (m, 1H, CH) 5.88-5.93 (m, 1H, CH), 7.00-8.03 ppm (20, H NH, NH2, aromatic ring); IR (KBr): υ (cm-1); IR (KBr): υ (cm-1) 1659, 1647, 3331; LC-MS (ESI) m/z (M + H)+ calcd for C34H47N9O7: 693, found 694.1.
Cell cytotoxicity
In order to evaluate the cytotoxic activity of the synthesis compounds, five cell lines: A2780 (low αVβ3), OVCAR-3 (high αVβ3), SKOV-3 (low CD13), HT-1080 (high CD13) and MCF-7 and fibroblast cell were selected. A2780 and MCF-7 are both cancer cells, but A2780 is αv β3 integrin overexpressing cell line. Three concentrations of products were prepared and were evaluated after 48 h by using MTT method. Therefore cells cultured in culture media (RPMI1640) at 37 °C under 5% CO2 supplemented with 10% fetal bovine serum (FBS), 100 U/mL penicillin and 100 μg/mL streptomycin. Nearly 104 cells were seeded into 96-well plates and were grown for 24 h in incubator. Compounds and doxorubicin added to wells, cells were incubated at 37 °C for 48 h. Cells without any adding of compound were used as negative control, Doxorubicin was used as positive control and DMSO as the solvent of the test compounds. After 48 h, 10 μL of MTT (5 mg/mL in PBS) was added at 37 °C for 4 h. The medium with MTT was removed and 100 μL DMSO was added to each well, cover the plate from light, shake it for 5 min, then optical densities were read at 570 nm with a reference wavelength of 630 nm as background using a spectrophotometer plate reader (Infinite® M200, TECAN).
Molecular modeling (docking) studies
Docking studies were performed using AutoDock Vina software for all the synthesized compounds to study their interactions with extracellular domain of the αvβ3 integrin receptor in the presence of the Mn2+ ion and with active sites of APN. The crystal structure of the extracellular domain of the αvβ3 integrin receptor in the presence of the Mn2+ ion (PDB entry code: 1L5G) (27) was obtained from the RCSB Protein Data Bank. Since in the X-ray structure the head group of αvβ3 integrin, which comprises the β propeller domain of αv and the β3 domain of β3, has been identified as the ligand binding region, only the globular head is considered for docking study. The X-ray crystal structure of APN was obtained from the RCSB Protein Data Bank (PDB code: 2DQM) (28).
All the compounds were built using hyperchem version 8 and subsequently minimized. The protein structure was prepared for docking using AutoDock Tool. Polar hydrogen was added and non-polar hydrogen was merged and finally Kollman united atom charge and atom type parameters were added to 1L5G for RGD products and 2DQM for NGR products. Grid map dimensions for 1L5G (18.6378 × 41.9200 × 43.1259) were set surrounding active site. The energy minimized ligands were docked in binding site of αvβ3 integrin receptor. The quality of the docked structures was assessed by measuring the intermolecular energy of the ligand-enzyme assembly. Grid map dimensions for 2DQM (71.9186 × 47.8031 × 7.3077) were set surrounding active site. The energy minimized ligands were docked in binding site of APN.
Results and Discussion
Chemistry
Ketoprofen were selected as NSAIDs and fmoc peptide synthesis strategy was used to make both the peptide-drug conjugates and peptide-spacer-drug conjugates. Therefore, fmoc-6-amino hexanoic acid was needed to make the peptide-spacer-drug conjugates (Scheme 1). The synthesis process is summarized in Scheme 2. The structures of all products are showed in Scheme 3.
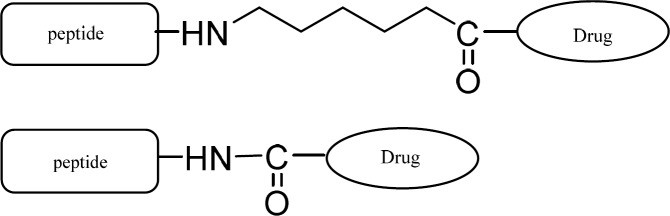
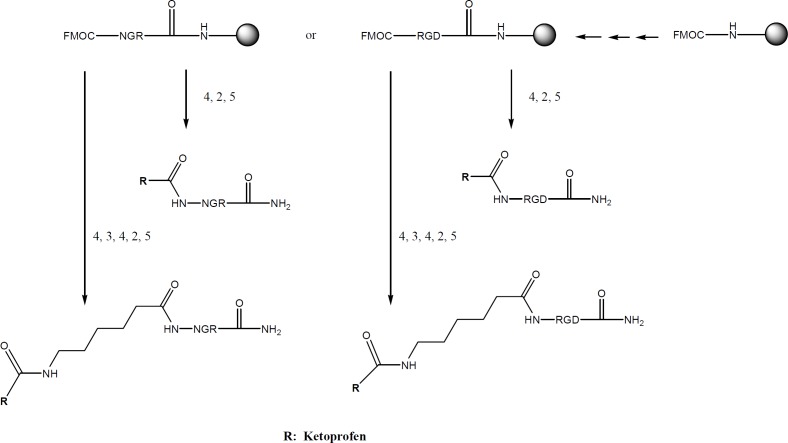
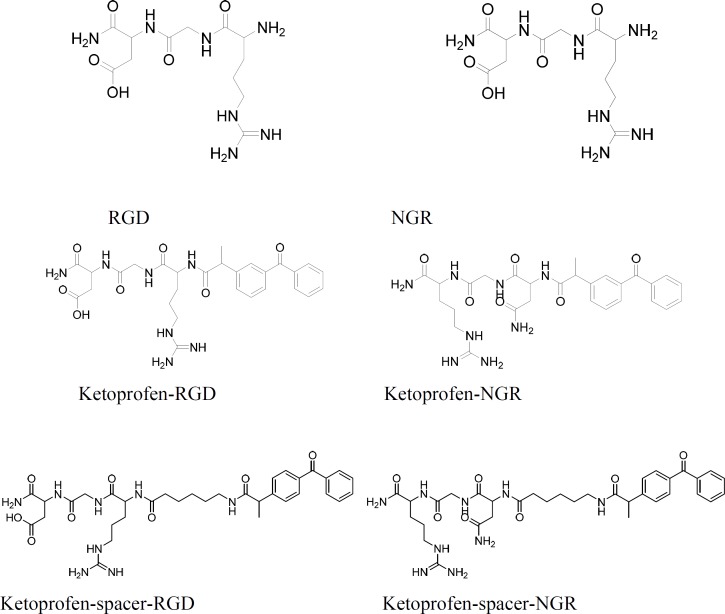
Biological activity
RGD and NGR are promising peptide sequences for targeted drug delivery to tumor cells. They can carry their drug conjugated to their target tumor. Ketoprofen was attached to N-terminus of the tripeptides RGD and NGR through its carboxylic acid group and thus an amide bond is responsible for the conjugation. In order to avoid the possible steric hindrance which is caused by the closeness of ketoprofen to the tripeptide, another from of the conjugate was also synthesized in which ketoprofen was attached to the tripeptide through a linear six carbon linker. Anti-proliferation activities of the synthesized compound were evaluated on a selected group of cancer cell lines. The results are summarized in Table 1: comparing the results reveals the following points:
a. No inhibitory activity was observed on MCF-7 (as a tumor cell without over-expressed RGD- or NGR-binding protein on its surface) or Fibroblasts (as normal non-tumor cells).
b. ketoprofen caused no inhibition at 100 µm concentration either on A2780 or on OVCAR-3. However the conjugated forms of ketoprofen with RGD either with or without spacer, showed >50% inhibition on both cell lines. The two cell lines are well recognized for the overexpression of RGD receptors (A2780 with low and OVCAR-3 with high expression of αvβ3) on their surface. In case of A2780, keto-spacer-RGD showed higher inhibitory activity than keto-RGD (63 vs. 55% inhibition) which indicates possible role of spacer either in better binding to the receptor or in mitigating the steric hindrance for better binding of RGD to the cell surface. Surprisingly, the opposite pattern is observed in case of OVCAR-3 which shows the possible impediment of linker group for the ligand-receptor binding.
c. NGR conjugates of ketoprofen caused 3-7 times higher inhibition compared to ketoprofen on HT-1080 and SKOV-3. These are the cancer cell lines with over expression of NGR receptor (SKOV-3 with low and HT-1080 with high expression of CD13) on their surface. Therefore it could be speculated that the NGR companionship with ketoprofen has improved its cytotoxic activity on these cell lines. In both cases, the conjugated form which contains the spacer between ketoprofen and NGR showed higher activity which could be assigned to the less hindrance for ligand- receptor binding.
Inhibitory % values of compounds at 100 µM concentration
A2780 | OVCAR3 | HT-1080 | SKOV-3 | MCF-7* | Fibroblast | |
---|---|---|---|---|---|---|
Ketoprofen | No inhibition | No inhibition | 9.25 ± 1.3 | 14 ± 4.7 | - | No inhibition |
Keto-RGD | 55 ± 0.5 | 77 ± 0.47 | - | - | 13 ± 0.2 | No inhibition |
Keto-NH-(CH2)4-CO-RGD | 63 ± 0.6 | 65 ± 2.7 | - | - | 36 ± 0.5 | No inhibition |
Keto-NGR | 28 ± 2.6 | - | 54 ± 0.5 | 50 ± 0.6 | - | No inhibition |
Keto-NH-(CH2)4-CO-NGR | 41 ± 0 | - | 76 ± 1 | 60 ± 0.2 | - | No inhibition |
RGD | 10 ± 2.2 | No inhibition | No inhibition | 9.5 ± 0.5 | No inhibition | No inhibition |
NGR | 6 ± 1.5 | No inhibition | 6.5 ± 0.5 | No inhibition | No inhibition | No inhibition |
Doxorubicin** | 65 ± 1.2 | 45.25 ± 1.25 | 26.7 ± 4.8 | 29.5 ± 2.5 | 38 ± 0.53** | - |
Molecular modeling (docking) studies
Aminopeptidase N (APN/CD13) which is assumed to be the target of ketoprofen-NGR conjugate is a zinc-dependent metalloprotease which is overexpressed in many disease such as cancer and inflammation. Bestatin is the first marketed APN inhibitor which has been introduced in 1976 (29). Therefor a docking study was conducted to investigate the possible binding mode of ketoprofen-spacer-NGR which has highest activity against HT-1080 cells. The result is presented in Figure 1. The carbonyl oxygen of ketoprofen can interact with the zinc ion with the distance of ca 6.6A˚. The distance of the guanidine residue of Arg 832 with guanidine residue of keto-spacer-RGD was 3.62A˚ and they form hyrogen bond. Tyrosine 376 is in proximity of the ketoprofen ring, suggesting a π-π stacking interaction between the two rings with the distance of 3.66A˚. Leucin 378 residue has come into contact with the 6 membered spacer which was used to prevent the possible steric interference between ketoprofen and NGR and thus causing the more efficient binding of ketoprofen-spacer-NGR to the receptor.
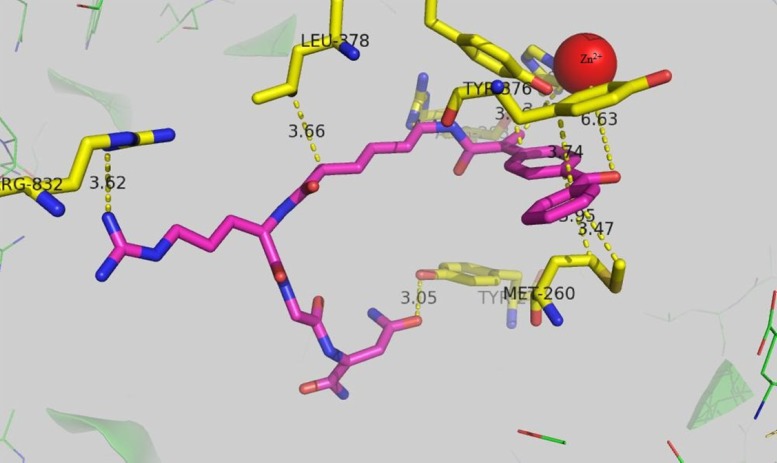
RGD target αvβ3 integrin subunit that is overexpressed in cancer. In order to explore the possible mode of interaction for keto-RGD conjugate, docking study was carried out using 1L5G crystallography of αvβ3 integrin and the results are presented in Figure 2. Arg 216 in β chain forms hydrogen bond with gunidine residue with the distance of 3.29 A˚and also (β)-Arg 214 forms hydrogen bon with 3.38A˚ from NH2 of asparagin moiety. The distance of Mn2+ ion in active site of enzyme from the carbonyl group of ketoprofen is 6.6A˚. Another Mn2+ ion in active site has 5.45A˚ distance from the carbonyl group of RGD peptide chain. Hydrophobic residue of (β)-Ala 218 with the distance of 3.9A˚ and 3.7A˚ has come into contact with ketoprofen rings. Hydroxyl group of (α)-Tyr178 forms hydrogen bond with NH of guanidine in keto-RGD with the distance of 3.88A˚ and also tyrosin ring has 3.49A˚ distance from CH2 side chain of Arg in keto-RGD.
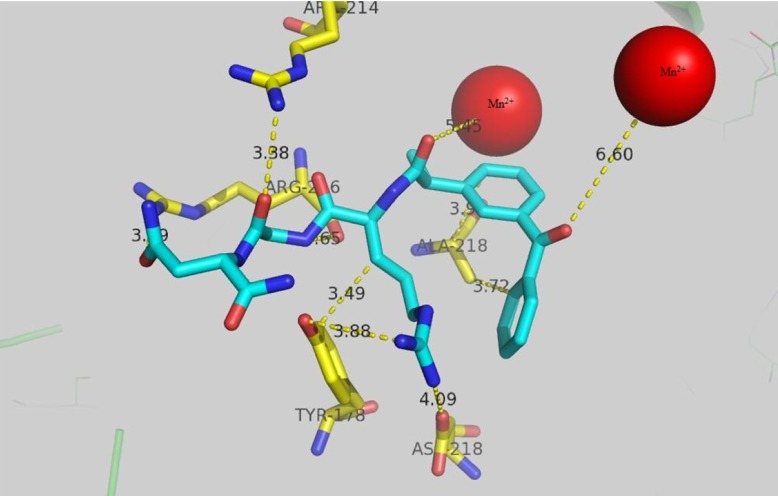
Conclusion
RGD and NGR peptide sequences are capable of guiding a chemotherapeutic agent to its target. This strategy could be used for maximizing the efficiency of chemotherapy based on the fact that in many cancer cells, specific membrane receptors are overexpressed on the cell surface. The result of present study show that ketoprofen conjugated to RGD and NGR have higher cytotoxic activity compared to ketoprofen itself which strongly supports the hypothesis of targeting by peptide-drug conjugates.
References
-
1.
Campbell SC, Volpert OV, Ivanovich M, Bouck NP. Molecular mediators of angiogenesis in bladder cancer. Cancer Res. 1998;58:1298-304. [PubMed ID: 9515819].
-
2.
Barczyk M, Carracedo S, Gullberg D. Integrins. Cell Tissue Res. 2010;339:269-80. [PubMed ID: 19693543].
-
3.
Brooks PC, Clark R, Cheresh DA. Requirement of vascular integrin alpha v beta 3 for angiogenesis. Science. 1994;264:569-71. [PubMed ID: 7512751].
-
4.
Raguse JD, Gath HJ, Bier J, Riess H, Oettle H. Cilengitide (EMD 121974) arrests the growth of a heavily pretreated highly vascularised head and neck tumour. Oral Oncol. 2004;40:228-30. [PubMed ID: 14693249].
-
5.
Taketo MM. Cyclooxygenase-2 inhibitors in tumorigenesis (Part II). J. Natl. Cancer Inst. 1998;90:1609-20. [PubMed ID: 9811310].
-
6.
Williams CS, Tsujii M, Reese J, Dey SK, DuBois RN. Host cyclooxygenase-2 modulates carcinoma growth. Eur. J. Clin. Invest. 2000;105:1589-94.
-
7.
Tsujii M, Kawano S, Tsuji S, Sawaoka H, Hori M, DuBois RN. Cyclooxygenase regulates angiogenesis induced by colon cancer cells. Cell. 1998;93:705-16. [PubMed ID: 9630216].
-
8.
Masferrer JL, Leahy KM, Koki AT, Zweifel BS, Settle SL, Woerner BM, Edwards DA, Flickinger AG, Moore RJ, Seibert K. Antiangiogenic and antitumor activities of cyclooxygenase-2 inhibitors. Cancer Res. 2000;60:1306-11. [PubMed ID: 10728691].
-
9.
Taketo MM. Cyclooxygenase-2 inhibitors in tumorigenesis (Part II). J. Natl. Cancer Inst. 1998;90:1609-20. [PubMed ID: 9811310].
-
10.
Steinbach G, Lynch PM, Phillips RK, Wallace MH, Hawk E, Gordon GB, Wakabayashi N, Saunders B, Shen Y, Fujimura T. The effect of celecoxib, a cyclooxygenase-2 inhibitor, in familial adenomatous polyposis. N. C. Med. J. 2000;342:1946-52.
-
11.
Domínguez Jiménez C, Díaz González F, González Álvaro I, Cesar JM, Sánchez Madrid F. Prevention of αIIbβ3 activation by non-steroidal antiinflammatory drugs. FEBS Lett. 1999;446:318-22. [PubMed ID: 10100866].
-
12.
Brooks PC, Montgomery AM, Rosenfeld M, Reisfeld RA, Hu T, Klier G, Cheresh DA. Integrin αvβ3 antagonists promote tumor regression by inducing apoptosis of angiogenic blood vessels. Cell. 1994;79:1157-64. [PubMed ID: 7528107].
-
13.
Pierschbacher MD, Ruoslahti E. Cell attachment activity of fibronectin can be duplicated by small synthetic fragments of the molecule. Nature. 1984;309:30. [PubMed ID: 6325925].
-
14.
Plow EF, Haas TA, Zhang L, Loftus J, Smith JW. Ligand binding to integrins. J. Biol. Chem. 2000;275:21785-8. [PubMed ID: 10801897].
-
15.
Hynes RO. Cell adhesion: Old and new questions. Trends Biochem. Sci. 1999;24:M33-M7.
-
16.
Hynes RO. A reevaluation of integrins as regulators of angiogenesis. Nat. Med. 2002;8:918-21. [PubMed ID: 12205444].
-
17.
Stupack DG, Cheresh DA. Get a ligand, get a life: Integrins, signaling and cell survival. J. Cell Sci. 2002;115:3729-38. [PubMed ID: 12235283].
-
18.
Varner JA, Cheresh DA. Integrins and cancer. Curr. Opin. Cell Biol. 1996;8:724-30. [PubMed ID: 8939661].
-
19.
Brooks PC, Montgomery AM, Rosenfeld M, Reisfeld RA, Hu T, Klier G, Cheresh DA. Integrin α v β 3 antagonists promote tumor regression by inducing apoptosis of angiogenic blood vessels. Cell. 1994;79:1157-64. [PubMed ID: 7528107].
-
20.
Jin S, Wang Y, Zhu H, Wang Y, Zhao S, Zhao M, Liu J, Wu J, Gao W, Peng S. Nanosized aspirin-Arg-Gly-Asp-Val: Delivery of aspirin to thrombus by the target carrier Arg-Gly-Asp-Val tetrapeptide. ACS Nano. 2013;7:7664-73. [PubMed ID: 23931063].
-
21.
Thun MJ, Henley SJ, Patrono C. Nonsteroidal anti-inflammatory drugs as anticancer agents: Mechanistic, pharmacologic, and clinical issues. J. Natl. Cancer Inst. 2002;94:252-66. [PubMed ID: 11854387].
-
22.
Zovko M, Zorc B, Jadrijević-Mladar Takač M, Zorc D. The novel fenoprofenamides: Synthesis and spectroscopic characterisation. Acta Pharm. 2001;51:107-15.
-
23.
Zovko M, Zorc B, Jadrijević Mladar Takač M, Metelko B, Novak P. The novel ketoprofenamides: synthesis and spectroscopic characterization. Croat. Chem. Acta. 2003;76:335-41.
-
24.
Kourounakis PN, Tsiakitzis K, Kourounakis AP, Galanakis D. Reduction of gastrointestinal toxicity of NSAIDs via molecular modifications leading to antioxidant anti-inflammatory drugs. Toxicology. 2000;144:205-10. [PubMed ID: 10781889].
-
25.
Cheng KC, Li YC, Yu CS, Yu FS, Lee JH, Lin ML, Yang JS, Chung JG. Ketoprofen-inhibited N-acetyltransferase activity and gene expression in human colon tumor cells. Anticancer Res. 2006;26:1105-11. [PubMed ID: 16619513].
-
26.
Marjanović M, Zorc B, Pejnović L, Zovko M, Kralj M. Fenoprofen and ketoprofen amides as potential antitumor agents. Chem. Biol. Drug Des. 2007;69:222-6. [PubMed ID: 17441909].
-
27.
Arnaout MA, Goodman SL, Xiong JP. Coming to grips with integrin binding to ligands. Curr. Opin. Cell Biol. 2002;14:641-52. [PubMed ID: 12231361].
-
28.
Ito K, Nakajima Y, Onohara Y, Takeo M, Nakashima K, Matsubara F, Ito T, Yoshimoto T. Crystal structure of aminopeptidase N (proteobacteria alanyl aminopeptidase) from Escherichia coli and conformational change of methionine 260 involved in substrate recognition. J. Biol. Chem. 2006;281:33664-76. [PubMed ID: 16885166].
-
29.
Umezawa H, Aoyagi T, Suda H, Hamada M, Takeuchi T. Bestatin, an inhibitor of aminopeptidase B, produced by actinomycetes. J. Antibiot. 1976;29:97-9. [PubMed ID: 931798].