Abstract
Keywords
Introduction
The genus Prangos Lindl. from Apiaceae family, consists of about 30 species, mainly distributed in Irano-Turanian phytogeographic region (1). In flora of Iran, this genus is represented by 14 species including Prangos ferulacea (L.) Lindl. (2).
P. ferulacea is a perennial plant with up to 1.5 m height which grows in Eastern Europe, Turkey, Caucasia and southwestern Asia (2). This species is known as "Jâshir" in Iran and its aerial parts are used traditionally as laxative and against ruminant parasites (3, 4). In eastern Anatolia, the aromatic aerial parts of P. ferulacea are used as a flavor in cheese and its stem is also used as digestive, antidiabetic and antihypertensive agent (5, 6). Beside, P. ferulacea is used as animal fodder because of its valuable nutritive properties (7).
Literature review revealed that some biological properties such as antioxidant (5, 8), antibacterial (9, 10), antispasmodic (11), abortifacient (12), analgesic (13) and hepatoprotective (14) activities have been reported for the aerial parts of P. ferulacea. It has also been reported that the roots of this medicinal species improve serum glucose and lipid profile in diabetic rats (15).
So far, several phytochemical studies have been conducted on essential oils and extracts obtained from the different parts of P. ferulacea (16-28). Tawaha et al. (2001) reported the isolation of four coumarins, herniarin, umbelliferone, scopoletin, and osthenol, along with two furanocoumarins, xanthotoxin and imperatorin from the aerial parts of P. ferulacea (23). A number of coumarin and furanocoumarin derivatives have also been reported from the roots of this species (24-28).
In the present study, we report essential oil constituents and isolation of the five phenolic derivatives, isoimperatorin (1), ferudenol (2), caffeic acid glucosyl ester (3), isorhamnetin-3-O-β-D-glucopyranoside (4) and quercetin-3-O-β-D-glucopyranoside (5) from the aerial parts of P. ferulacea growing in Northwest of Iran. To the best of our knowledge, this is the first report on isolation and structure elucidation of these phenolic derivatives from the P. ferulacea aerial parts.
Experimental
General procedures
1H-NMR and 13C-NMR spectra were acquired in CDCl3 and DMSO-d6 on a Bruker Avance DRX 400 spectrometer. EI-MS spectra were obtained on a Hewlett-Packard model 5973 HP system. UV spectra were recorded in methanol (and after the addition of shift reagents) on a CECIL 7250 spectrophotometer.
Silica gel (230-400 mesh, Merck), fully endcappedRP-18 (230-400 mesh, Fluka) and Sephadex LH-20 (Fluka) were applied for column chromatographies. Preparative thin layer chromatography (PTLC) was also performed on handmade silica gel 60 GF254 (Merck) plates. Pre-coated silica gel GF254 aluminum sheets (Merck) were used for TLC and monitoring of the spots were carried out under UV (254 and 366 nm) and by spraying with anisaldehyde-H2SO4 reagent followed by heating at 120°C for 5 min. All of the solvents were also obtained from Merck chemical company.
Plant material
The flowering aerial parts of P. ferulacea were collected from the Razi border, located in Khoy County (West-Azerbaijan Province, Northwest of Iran) in June 2014. The plant was identified by botanist Dr. Yousef Ajani, Institute of Medicinal Plants, Academic Center for Education, Culture and Research (ACECR), Karaj, Iran.
Extraction and fractionation
The air-dried aerial parts of P. ferulacea (1kg) were grinded and extracted exhaustively with MeOH by maceration method at the room temperature (10×12 L and 48 h each time). The combined extracts were concentrated by a rotary evaporator at 45°C. The obtained total extract (274.58 g) was fractionated successively with petroleum ether and chloroform using liquid-liquid extraction, to get three main fractions, petroleum ether, chloroform and methanol (residue) fractions.
Isolation and purification of compounds
The petroleum ether fraction (12 g) was dissolved in petroleum ether (200 ml) and transferred into a flask. Following the addition of ethanol (30 ml) to above solution, a white precipitate was appeared which was then separated by filtration and named fraction E2.
The fraction E2 (7 g) was moved over a silica gel column (4.5×30 cm) and eluted with n-hexane-EtOAc (9:1 to 5:5) to get fourteen fractions (E2A-E2N). Fraction E2B (52 mg) was chromatographed on silica gel PTLC with n-hexane-EtOAc (8:2), to give compound 1 (9 mg). Compound 2 (5 mg) was also obtained from the fraction E2I (18 mg) by chromatography on silica gel PTLC (n-hexane-EtOAc, 6:4). A part of methanol fraction (6 g) was moved in two divided portions over a sephadex LH-20 column (4×25 cm) and eluted with MeOH-H2O (8:2), to get three fractions (M1-M3). Reversed phase C18 column chromatography (3×15 cm) of the fraction M2 (2 g) with ACN (acetonitrile)-H2O (1:9-3:7) yielded eleven fractions (M2A-M2K). Chromatography of the fraction M2A (250 mg) on a RP-18 column (1.5×20 cm) using ACN-H2O (0.5:9.5) resulted in isolation of compound 3 (18 mg). Fraction M2H (38 mg) was rechromatographed on a RP-18 column (1×20 cm) with ACN-H2O (1:9-2:8) to obtain compound 4 (21 mg). Compound 5 (7 mg) was also achieved from the fraction M2I (34 mg) through RP-18 column chromatography (1×20 cm) using ACN-H2O (1.5:8.5) as eluent and its impurities were removed over a sephadex LH-20 column (MeOH-H2O, 8:2).
Isolation of essential oil
Essential oil of the plant aerial parts was extracted using hydro-distillation for 4 h by a Clevenger-type apparatus. The prepared oil was subsequently dried over anhydrous sodium sulfate and stored at 4°C in the dark until analysis.
GC-MS analysis
An Agilent 6890 gas chromatograph (Column: BPX5, 30 m × 0.25 mm (id), 0.25 µm film thickness) equipped with a MS detector (Agilent 5973, EI mode at 70 eV, 220 °C) was applied for the essential oil analysis. The flow rate of carrier gas (Helium) was 0.5 ml min-1. The oven temperature was raised from 50 °C to 240 °C at a rate of 3 °C per minute and then raised to 300 °C at a rate of 15 °C and finally maintained at 300 °C for 3 min. The injection temperature was 290 °C, and the oil sample (1.0 µL) was injected with a split ratio of 1:30. The Kovats retention indices (KI) of the compounds were calculated using a homologous series of n-alkanes (C8-C30) injected in conditions equal to the samples. Identification of the chemical constituents was performed using Wiley7n.l online library, as well as by direct comparison of their MS spectra and KIs with data published in the literature for standard compounds (29).
Results
Phytochemical investigation of the aerial parts of P. ferulacea yielded the isolation of five compounds. The structures of isolated compounds were established as isoimperatorin (1), ferudenol (2), caffeic acid glucosyl ester (3), isorhamnetin-3-O-β-D-glucopyranoside (4) and quercetin-3-O-β-D-glucopyranoside (isoquercetin) (5) (Figure 1) using their 1H- & 13C-NMR, EI-MS and UV spectral analyses, and also by comparison with related data published in the literature (27, 30-33).
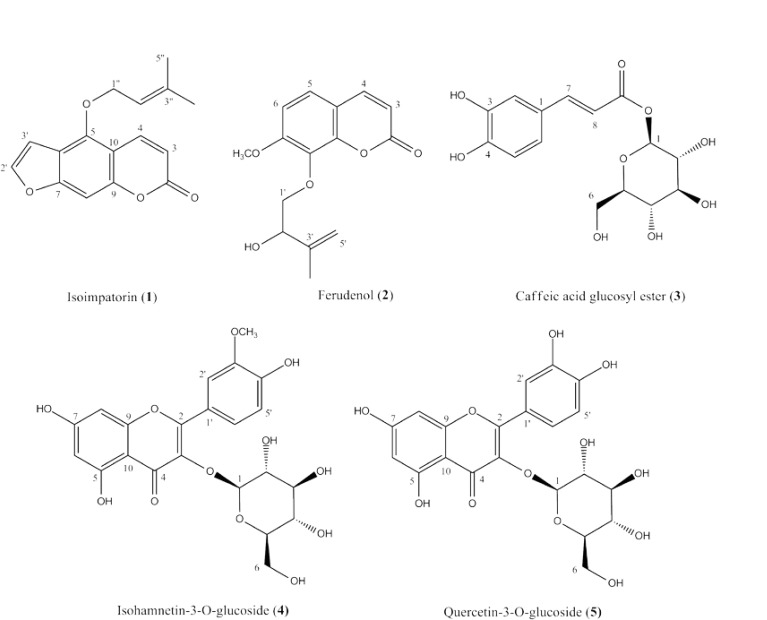
Spectral data of the isolated compounds
Compound 1; Isoimperatorin: White crystals, 1H-NMR (CDCl3, 400 MHz): δ 8.17 (1H, d, J= 9.8 Hz, H4), 7.60 (1H, d, J= 2.2 Hz, H2'), 7.15 (1H, s,H8), 6.97 (1H, d, J= 2.2 Hz, H3'), 6.26 (1H, d, J= 9.8 Hz, H3), 5.55 (1H, t, J= 6.8 Hz, H2''), 4.93 (2H, d, J= 6.8, H1''), 1.81 (3H, s, H5''), 1.71 (3H, s, H4''); 13C-NMR (CDCl3, 100 MHz); 161.1 (C2), 158.2 (C7), 152.6 (C9), 148.8 (C5), 144.8 (C2'), 139.7 (C3''), 139.4 (C4), 119.2 (C2''), 114.9 (C6), 112.3 (C3), 107.3 (C10), 105.1 (C3'), 95.1 (C8), 69.8 (C1''), 25.8 (C5''), 18.3 (C4''); UV (MeOH) λ max: 249, 260, 309 (30).
Compound 2; Ferudenol: White crystals, 1H-NMR (CDCl3, 400 MHz): δ 8.52 (1H, s, OH), 7.65 (1H, d, J= 9.6 Hz, H4), 7.37 (1H, d, J= 8.6 Hz, H5), 6.88 (1H, d, J= 8.6 Hz, H6), 6.27 (1H, d, J= 9.6 Hz, H3), 4.89 (2H, d, J= 21.0 Hz, H5'), 4.62 (1H, dd, J= 8.0, 5.5 Hz, H2'), 3.96 (3H, s, OCH3), 3.28 (1H, dd, J= 11.3, 8.0Hz, H1'a), 3.17 (1H, dd, J= 11.3, 5.5 Hz, H1'b),1.92 (3H, s, H4'); UV (MeOH) λ max: 210, 253 (sh), 322.; EI-MS (m/z): 276 [M+], 258, 219, 203, 189, 175, 131 (27).
Compound 3; Caffeic acid glucosyl ester: Light brown solid, 1H-NMR (DMSO-d6, 400 MHz): δ 7.46 (1H, d, J= 15.3 Hz, H7), 7.04 (1H, brs, H2), 6.95 (1H, brd, J= 8.1, H6), 6.75 (1H, d, J= 8.1, H5), 6.23 (1H, d, J= 15.3 Hz, H8), 4.10 (1H, d, J= 7.6 Hz, H1'), 2.8-3.8 (6H, H2'-6'); 13C-NMR (DMSO-d6, 100 MHz): δ 170.1 (C9), 150.2 (C4), 149.6 (C3), 146.3 (C7), 128.3 (C1), 125.1 (C6), 118.4 (C8), 117.5 (C5), 115.1 (C2), 102.4 (C1'), 79.1 (C3'), 74.6 (C2', C5'), 69.1 (C4'), 60.7 (C6'); UV (MeOH) λ max: 206, 245 (sh), 300 (sh), 328., +AlCl3: 206, 259, 304 (sh), 366., +AlCl3/HCl: 206, 272 (sh), 302 (sh), 342 (31).
Compound 4; Isorhamnetin-3-O-β-D-glucopyranoside: Yellow powder, 1H-NMR (DMSO-d6, 400 MHz): δ 8.06 (1H, brs, H2'), 7.40 (1H, brd, J= 8.5 Hz, H6'), 6.91 (1H, d, J= 8.5 Hz, H5'), 6.29 (1H, brs, H8), 6.09 (1H, brs, H6), 5.50 (1H, d, J= 6.8 Hz, H1''), 3.82 (3H, s, OCH3), 3.0-4.0 (6H, H2''-6''); 13C-NMR (DMSO-d6, 100 MHz): δ 177.0 (C4), 167.3 (C7), 161.1 (C5), 156.6 (C2), 155.2 (C9), 149.8 (C3'), 145.9 (C4'), 133.4 (C3), 122.8 (C6'), 121.2 (C1'), 115.6 (C5'), 111.3 (C2'), 103.6 (C10), 101.0 (C1''), 99.5 (C6), 93.9 (C8), 77.5 (C3''), 76.5 (C5''), 74.1 (C2''), 69.9 (C4''), 60.9 (C6''), 55.6 (OCH3); UV (MeOH) λ max: 253, 335, 360 (sh)., +AlCl3: 365, 266., +AlCl3/HCl: 266, 305 (sh), 355, 404 (sh)., +NaOMe: 272, 329 (sh), 395., +NaOAc: 332, 269; EI-MS (m/z): 299 [Aglycon+], 149, 137 (32).
Compound 5; Quercetin-3-O-β-D-glucopyranoside (Isoquercetin): Yellow powder, 1H-NMR (DMSO-d6, 400 MHz): δ 8.28 (1H, brs, H2'); 7.35 (1H, brd, J= 7.9 Hz, H6'); 6.82 (1H, d, J= 8.3 Hz, H5'); 6.40 (1H, brs, H8); 6.20 (1H, brs, H6); 5.23 (1H, d, J= 5.9 Hz, H1'') 3.0-4.0 (6H, H2''-6''); 13C-NMR (DMSO-d6, 100 MHz): δ 177.3 (C4), 164.5 (C7), 161.2 (C5), 156.3 (C2), 156.1 (C9), 148.5 (C4'), 144.8 (C3'), 133.2 (C3), 121.5 (C6'), 121.1 (C1'), 116.1 (C5'), 115.2 (C2'), 103.8 (C10), 100.8 (C1''), 98.7 (C6), 93.5 (C8), 77.5 (C5''), 76.4 (C3''), 74.0 (C2''), 69.9 (C4''), 60.9 (C6''); UV (MeOH) λmax: 259, 368., +AlCl3: 269, 304 (sh), 421., + NaOMe: 272, 411., +NaOAc: 262, 368 (33).
The hydrodistillation of the plant aerial parts yielded 0.2% (V/W) pale yellowish oils. Twenty seven compounds (99.25% of the total oil) were identified as a result of GC-MS analysis of P. ferulacea essential oil, among them β-pinene (43.1%), α-pinene (22.1%) and δ-3-carene (16.9%) were characterized as main compounds (Table 1). The results also indicated that monoterpene hydrocarbons (94.45%) were the main group of constituents in P. ferulacea essential oil.
Chemical composition of the essential oil of P. ferulacea aerial parts
No. | Compoundsa | KIb | % | No. | Compounds | KI | % |
---|---|---|---|---|---|---|---|
1 | α-thujene | 926 | 0.10 | 18 | trans-verbenol | 1142 | 0.50 |
2 | α-pinene | 938 | 22.1 | 19 | pinocarvone | 1163 | 0.36 |
3 | camphene | 947 | 0.13 | 20 | p-cymene-8-ol | 1183 | 0.67 |
4 | sabinene | 969 | 0.57 | 21 | myrtenal | 1194 | 0.23 |
5 | β-pinene | 975 | 43.13 | 22 | β-elemene | 1388 | 0.61 |
6 | β-myrcene | 989 | 1.92 | 23 | β-caryophyllene | 1415 | 0.29 |
7 | δ-3-carene | 1008 | 16.88 | 24 | elemol | 1548 | 0.14 |
8 | α-terpinene | 1016 | 0.12 | 25 | caryophyllene oxide | 1582 | 0.26 |
9 | p-cymene | 1021 | 0.06 | 26 | α-bisabolol | 1687 | 0.46 |
10 | o-cymene | 1023 | 0.95 | 27 | osthole | 2144 | 1.28 |
11 | limonene | 1024 | 2.06 | ||||
12 | β-phellandrene | 1027 | 1.89 | Monoterpene hydrocarbons | 94.45 | ||
13 | (Z)-β-ocimene | 1032 | 0.07 | Oxygenated monoterpenes | 1.76 | ||
14 | (E)-β-ocimene | 1046 | 0.10 | Sesquiterpene hydrocarbons | 0.90 | ||
15 | γ-terpinene | 1054 | 0.26 | Oxygenated sesquiterpenes | 0.86 | ||
16 | α-terpinolene | 1085 | 3.90 | Non-terpenes | 1.28 | ||
17 | p,α-dimethylstyrene | 1099 | 0.21 | Total identified | 99.25 |
Discussion
Two coumarin derivatives, isoimperatorin (1) and ferudenol (2) were isolated from the petroleum ether fraction of P. ferulacea aerial parts. These compounds (1 and 2) have been previously reported from the roots of P. ferulacea (24, 27, 28). A phytochemical study on P. uloptera aerial parts, however, reported the isolation of five other coumarin derivatives, xanthotoxin, prangenin, scopoletin, deltoin and prangolarin from its n-hexane extract (34). In 2006, Pokharel et al. showed that isoimperatorin possesses a considerable hepatoprotective effects against aflatoxin B1 through induction of glutathione S-transferase α (GSTα) and direct inhibition of CYP1A activity (35). It has also been reported that this compound (1) exerts a potent anti-inflammatory activity via cyclooxygenase-2 and 5-lipoxygenase inhibitory activity, as well as by inhibition of TNF-α-stimulated VCAM-1 (vascular cell adhesion molecule-1) expression (36, 37). Furthermore, antibacterial effects of the isoimperatorin against Mycobacterium tuberculosis (38) and its acetylcholinesterase (AChE) inhibitory activity (39) have been demonstrated during previous biological investigations. Phytochemical study on the methanolic fraction of the plant aerial parts also yielded caffeic acid glucosyl ester (3), together with two flavonol glycosides, isorhamnetin-3-O-β-D-glucopyranoside (4) and quercetin-3-O-β-D-glucopyranoside (Isoquercetin) (5). Caffeic acid, a bioactive phenolic compound, is found in many plant families, alone or in combination with sugars, quinic acid (caffeoylquinic acids), phenyl ethanol (caffeic acid phenethyl esters) and etc. (40). A review of literature on caffeic acid derivatives revealed that the glucose could be connected to caffeic acid through OH-3, OH-4 or COOH groups (31, 41, 42). In the present study, the structure of caffeic acid glucosyl ester was confirmed for compound 3, based on the observed bathochromic sift (+38 nm), followed by addition of AlCl3 to the methanolic solution of compound 3 which was indicated to the presence of a ortho dihydoxy group in compound 3 (Figure 1) (43). Caffeic acid glucosyl ester has also been reported as 2-methyl-3-[3'-O-caffeic acid glucosyl ester]-γ-pyrone from the aerial parts of P. tschimganica (44). Two isolated flavonoids, isorhamnetin-3-O-β-D-glucopyranoside (4) and quercetin-3-O-β-D-glucopyranoside (Isoquercetin) (5), have been documented for their antioxidant (45, 46), α-glucosidase inhibitory (45, 46), anti-inflammatory (47, 48), antimicrobial (49) and antidiabetic (50) activities. Isorhamnetin-3-О-β-D-glucopyranoside has also been reported as an antiobesity agent with antiadipogenic activity on 3T3-L1 adipocytes (51).
Essential oil analysis of the P. ferulacea aerial parts resulted to the identification of twenty seven compounds, mainly β-pinene (43.1%), α-pinene (22.1%), δ-3-carene (16.9%) (Table 1). Osthole, a pernylated coumarin was identified in the plant essential oil with the relative percentage of 1.28%. This compound has been previously reported from the roots of P. ferulacea with antispasmodic effects on ileum contraction (11). Previous reports on essential oil composition of the different parts of P. ferulacea have been summarized in Table 2.
The results of essential oil analysis of P. ferulacea from the previous and present studies
Location of plant collection | Date | Method | Part(s) | Main compounds (%) | Reference |
---|---|---|---|---|---|
Khoy | June 2012 | HDa | aerial part | β-pinene (43.1%) | Present |
Isfahan | July 1996 | SDb | aerial part | β-pinene (22.9%) | (16) |
Myaneh | July 2005 | HD | aerial part | (E)-anethole (95.0%) | (17) |
Myaneh | May 2005 | HD | leaves & | α-pinene (57.0%) | (17) |
Sirjan | June 2010 | HD | leaves | α-pinene (28.2%) | (21) |
Myaneh | - | HD | flowers | α-pinene (42.2%) | (18) |
Tosya | Sept. 1994 | HD | fruits | γ-terpinene (30.2%) | (20) |
Sanandaj | July 2001 | SD | fruits | β-pinene (33.0%) | (16) |
Shemshak | June 2006 | HD | fruits | chrysanthenyl acetate (26.5%) | (19) |
Sirjan | June 2010 | HD | fruits | α-pinene (25.4%) | (21) |
Myaneh | - | HD | fruits | α-pinene (61.3%) | (18) |
Yasouj | June 2010 | HD | roots | δ-3-carene (22.5%) | (22) |
Sefidkon et al. (1998) reported β-pinene (22.9%), δ-3-carene (16.0%) and α-pinene (12.6%) as the main compounds of the essential oil of P. ferulacea aerial parts from Isfahan (center of Iran) (16). The comparison of the results represented in this paper with the former study on P. ferulacea essential oil from Isfahan province (Iran) revealed that α-thujene, α-pinene, camphene, β-pinene, β-myrcene, δ-3-carene, α-terpinene, p-cymene, (Z)-β-ocimene, (E)-β-ocimene, γ-terpinene, β-caryophyllene and α-bisabolol are the compounds present in both essential oil samples (16). Furthermore, in another study on essential oil of the plant flowering aerial parts from East-Azerbaijan (northwest of Iran), (E)-anethole (95.0%) and α-pinene (1.2%) were characterized as its main compounds (17). (E)-anethole, an aromatic major compound of the last mentioned study, however, was not identified in our analysed oil sample (17). The present study reports the monoterpene hydrocarbons (94.5%) as main group of constituents in P. ferulacea essential oil, whereas two previous reports from Isfahan and East-Azerbaijan provinces (Iran) reported the level of monoterpene hydrocarbons at 64.0 and 2.7%, respectively (16, 17). Genetic variations and climatic conditions could be considered as main factors involved in the chemical differences in composition of the essential oils obtained from P. ferulacea aerial parts (52).
Conclusion
The results of present study on isolation of bioactive phenolic derivatives (1-5) from the aerial parts of P. ferulacea emphasize the therapeutic potentials of this medicinal species and suggest it as an appropriate candidate for further biological and pharmacological research. This study also introduces P. ferulacea as a plant with monoterpene rich oil and report β-pinene (43.1%) as the main compound of its aerial parts oil.
Acknowledgements
References
-
1.
Şenol SG, Yildirim H, Seçmen O. Prangos hulusii sp nov (Apiaceae) from West Anatolia, Turkey. Nord. J. Bot. 2011;29:402-407.
-
2.
Mozaffarian V. Flora of Iran. No.54: Umbelliferae. Tehran: Publication of Research Institute of Forests and Rangelands; 2007. p. 220-222.
-
3.
Ghasemi PA, Momeni M, Bahmani M. Ethnobotanical study of medicinal plants used by Kurd tribe in Dehloran and Abdanan districts, Ilam Province, Iran. Afr. J. Tradit. Complement. Altern. Med. 2013;10:368-385. [PubMed ID: 24146463].
-
4.
Bahmani M, Rafieian-Kopaei M, Avijgan M, Hosseini S, Golshahi H, Eftekhari Z, Gholizadeh GH. Ethnobotanical studies of medicinal plants used by Kurdish owner's in south range of Ilam Province, West of Iran. Am-Euras. J. Agri. Environ. Sci. 2012;12:1128-1133.
-
5.
Coruh N, Celep AS, Özgökçe F. Antioxidant properties of Prangos ferulacea (L) Lindl Chaerophyllum macropodum Boiss and Heracleum persicum Desf from Apiaceae family used as food in Eastern Anatolia and their inhibitory effects on glutathione-S-transferase. Food Chem. 2007;100:1237-1242.
-
6.
Özgen U, Kaya Y, Houghton P. Folk medicines in the villages of Ilica District (Erzurum, Turkey). Turk. J. Biol. 2012;36:93-106.
-
7.
Coşkun B, Gülşen N, Umucalilar H. The nutritive value of Prangos ferulacea. Grass Forage Sci. 2004;59:15-19.
-
8.
Mavi A, Terzi Z, Ozgen U, Yildirim A, Coşkun M. Antioxidant properties of some medicinal plants: Prangos ferulacea (Apiaceae) Sedum sempervivoides (Crassulaceae) Malva neglecta (Malvaceae) Cruciata taurica (Rubiaceae), Rosa pimpinellifolia (Rosaceae) Galium verum subsp verum (Rubiaceae) Urtica dioica (Urticaceae). Biol.Pharm.Bull. 2004;27:702-705. [PubMed ID: 15133249].
-
9.
Durmaz H, Sagun E, Tarakci Z, Ozgokce F. Antibacterial activities of Allium vineale, Chaerophyllum macropodum and Prangos ferulacea. Afr. J. Biotech. 2006;5:1795-1798.
-
10.
Sagun E, Durmaz H, Tarakci Z, Sagdic O. Antibacterial activities of the extracts of some herbs used in Turkish herby cheese against Listeria monocytogenes serovars. Int.J.Food Prop. 2006;9:255-260.
-
11.
Sadraei H, Shokoohinia Y, Sajjadi S, Mozafari M. Antispasmodic effects of Prangos ferulacea acetone extract and its main component osthole on ileum contraction. Res. Pharm. Sci. 2013;8:137-144. [PubMed ID: 24019823].
-
12.
Kazerooni T, Mousavizadeh K, Abdollahee A, Sarkarian M, Sattar A. Abortifacient effect of Prangos ferulacea on pregnant rats. Contraception. 2006;73:554-556. [PubMed ID: 16627045].
-
13.
Emamghoreishi M, Taghavi A, Javidnia K. The effect of aqueous and methanolic extracts of Prangos ferulacea on formalin-induced pain in mice. J. Jahrom Uni. Med. Sci. 2012;9:1-6.
-
14.
Farokhi F, Farkhad NK, Togmechi A. Preventive effects of Prangos ferulacea (L) Lindle on liver damage of diabetic rats induced by alloxan. Avicenna J. Phytomed. 2012;2:63-71. [PubMed ID: 25050232].
-
15.
Farkhad NK, Farokhi F, Tukmacki A. Hydro-alcoholic extract of the root of Prangos ferulacea (L) Lindl can improve serum glucose and lipids in alloxan-induced diabetic rats. Avicenna J. Phytomed. 2012;2:179-187. [PubMed ID: 25050248].
-
16.
Sefidkon F, Khajavi M, Malackpour B. Analysis of the oil of Prangos ferulacea (L) Lindl. J. Essent. Oil Res. 1998;10:81-82.
-
17.
Razavi S. Chemical composition and some allelopathic aspects of essential oils of (Prangos ferulacea L) Lindl at different stages of growth. J. Agri. Sci. Tech. 2011;14:349-356.
-
18.
Razavi SM, Nazemiyeh H, Zarrini G, Asna-Asharii S, Dehghan G. Chemical composition and antimicrobial activity of essential oil of Prangos ferulacea (L) Lindl from Iran. Nat. Prod. Res. 2010;24:530-533. [PubMed ID: 19452346].
-
19.
Massumi M, Fazeli M, Alavi S, Ajani Y. Chemical constituents and antibacterial activity of essential oil of Prangos ferulacea (L) Lindl fruits. Iran. J. Pharm. Sci. 2007;3:171-176.
-
20.
Baser K, Ermin N, Adigüzel N, Aytac Z. Composition of the essential oil of Prangos ferulacea (L) Lindl. J. Essenti. Oil Res. 1996;8:297-298.
-
21.
Akhgar M. Composition of essential oils of fruits and leaves of Prangos ferulacea (L) Lindl growing wild in Iran. Trends Mod. Chem. 2011;1:1-4.
-
22.
Sajjadi SE, Shokoohinia Y, Gholamzadeh S. Chemical composition of essential oil of Prangos ferulacea (L) Lindl roots. Chemija. 2011;22:178-180.
-
23.
Tawaha K, Al-Muhtaseb S, Al-Khalil S. Coumarins from the aerial parts of Prangos ferulacea. Alexandria J. Pharm. Sci. 2001;15:89-90.
-
24.
Shokoohinia Y, Sajjadi SE, Gholamzadeh S, Fattahi A, Behbahani M. Antiviral and cytotoxic evaluation of coumarins from Prangos ferulacea. Pharm. Biol. 2014;52:1543-1549. [PubMed ID: 25026335].
-
25.
Abyshev A, Denisenko P, Kostyuchenko N, Anisimova O, Ermakov E, Sheinker YN. Pranferin-A new coumarin from the roots of Prangos ferulacea. Chem. Nat. Compd. 1970;6:689-692.
-
26.
Abyshev A, Denisenko P, Kostyuchenko N, Ermakov A, Sheinker YN. Gosferol-A new furocoumarin from the roots of Prangos ferulacea. Chem. Nat. Compd. 1972;8:43-47.
-
27.
Abyshev A. Structure of some components of the roots of Prangos ferulacea. Chem. Nat. Compd. 1974;10:581-585.
-
28.
Eshbakova K, Saidkhodzhaev A, Baser KH, Duman H, Vdovin A, Abdullaev N. Furocoumarins from Prangos ferulacea. Chem. Nat. Compd. 2006;42:102-103.
-
29.
Adams RP. Identification of Essential Oil Components by Gas Chromatography/Mass Spectrometry. Carol Stream: Allured Publishing Corporation; 2007.
-
30.
Yang F, Zhang T, Liu Q, Xu G, Zhang Y, Zhang S, Ito Y. Preparative isolation and purification of notopterol and isoimperatorin from Notopterygium forbessi Boiss (Chinese traditional medicinal herb) by high-speed counter-current chromatography. J. Chromatogr. A. 2000;883:67-73. [PubMed ID: 10910201].
-
31.
Yassa N, Saeidnia S, Pirouzi R, Akbaripour M, Shafiee A. Three phenolic glycosides and immunological properties of Achillea millefolium from Iran, population of Golestan. DARU J. Pharm. Sci. 2007;15:49-52.
-
32.
Yeskaliyeva B, Mesaik MA, Abbaskhan A, Kulsoom A, Burasheva GS, Abilov ZA, Choudhary MI, Atta-ur-Rahman. Bioactive flavonoids and saponins from Climacoptera obtusifolia. Phytochemistry. 2006;67:2392-2397. [PubMed ID: 16956629].
-
33.
Eldahshan OA. Isolation and structure elucidation of phenolic compounds of carob leaves grown in Egypt. Curr. Res. J. Biol. Sci. 2011;3:52-55.
-
34.
Razavi SM, Nazemiyeh H, Hajiboland R, Kumarasamy Y, Delazar A, Nahar L, Sarker SD. Coumarins from the aerial parts of Prangos uloptera (Apiaceae). Braz. J. Pharmacog. 2008;18:1-5.
-
35.
Pokharel YR, Han EH, Kim JY, Oh SJ, Kim SK, Woo ER, Jeong HG, Kang KW. Potent protective effect of isoimperatorin against aflatoxin B1-inducible cytotoxicity in H4IIE cells: bifunctional effects on glutathione S-transferase and CYP1A. Carcinogenesis. 2006;27:2483-2490. [PubMed ID: 16829687].
-
36.
Moon TC, Jin M, Son JK, Chang HW. The effects of isoimperatorin isolated from Agelicae dahuricae on cyclooxygenase-2 and 5-lipoxygenase in mouse bone marrow-derived mast cells. Arch. Pharm. Res. 2008;31:210-215. [PubMed ID: 18365692].
-
37.
Moon L, Ha YM, Jang HJ, Kim HS, Jun MS, Kim YM, Lee YS, Lee DH, Son KH, Kim HJ, Seo HG, Lee JH, Kim YS, Chang KC. Isoimperatorin, cimiside E and 23-O-acetylshengmanol-3-xyloside from cimicifugae rhizome inhibit TNF-α-induced VCAM-1 expression in human endothelial cells: involvement of PPAR-γ upregulation and PI3K, ERK1/2, and PKC signal pathways. J. Ethnopharmacol. 2011;133:336-344. [PubMed ID: 20937376].
-
38.
Guo N, Wu J, Fan J, Yuan P, Shi Q, Jin K, Cheng W, Zhao X, Zhang Y, Li W, Tang X, Yu L. In-vitro activity of isoimperatorin, alone and in combination, against Mycobacterium tuberculosis. Lett .Appl. Microbiol. 2014;58:344-349. [PubMed ID: 24330002].
-
39.
Kim DK, Lim JP, Yang JH, Eom DO, Eun JS, Leem KH. Acetylcholinesterase inhibitors from the roots of Angelica dahurica. Arch. Pharm. Res. 2002;25:856-859. [PubMed ID: 12510838].
-
40.
Touaibia M, Jean-François J, Doiron J. Caffeic acid, a versatile pharmacophore: an overview. Mini Rev. Med. Chem. 2011;11:695-713. [PubMed ID: 21679136].
-
41.
Ha TJ, Lee JH, Lee MH, Lee BW, Kwon HS, Park CH, Shim KB, Kim HT, Baek Y, Jang DS. Isolation and identification of phenolic compounds from the seeds of Perilla frutescens (L) and their inhibitory activities against α-glucosidase and aldose reductase. Food Chem. 2012;135:1397-1403. [PubMed ID: 22953872].
-
42.
Regos I, Urbanella A, Treutter D. Identification and quantification of phenolic compounds from the forage legume sainfoin (Onobrychis viciifolia). J.Agri. Food Chem. 2009;57:5843-5852.
-
43.
Mabry TJ, Markham KR, Thomas MB. The Systematic Identification Of Flavonoids. New York: Springer-Verlag; 1970. p. 35-40.
-
44.
Shikishima Y, Takaishi Y, Honda G, Ito M, Takeda Y, Kodzhimatov OK, Ashurmetov O. Terpenoids and γ-pyrone derivatives from Prangos tschimganica. Phytochemistry. 2001;57:135-141. [PubMed ID: 11336255].
-
45.
Shibano M, Kakutani K, Taniguchi M, Yasuda M, Baba K. Antioxidant constituents in the dayflower (Commelina communis L) and their α-glucosidase-inhibitory activity. J. Nat. Med. 2008;62:349-53. [PubMed ID: 18409066].
-
46.
Jo S, Ka E, Lee H, Apostolidis E, Jang H, Kwon Y. Comparison of antioxidant potential and rat intestinal α-glucosidases inhibitory activities of quercetin, rutin, and isoquercetin. Int. J. Appl. Res. Nat. Prod. 2009;2:52-60.
-
47.
Ahmed M, El Tanbouly N, Islam W, Sleem A, El Senousy A. Antiinflammatory flavonoids from Opuntia dillenii (Ker‐Gawl) Haw flowers growing in Egypt. Phytother. Res. 2005;19:807-809. [PubMed ID: 16220577].
-
48.
Toker G, Küpeli E, Memisoğlu M, Yesilada E. Flavonoids with antinociceptive and anti-inflammatory activities from the leaves of Tilia argentea (silver linden). J. Ethnopharmacol. 2004;95:393-397. [PubMed ID: 15507365].
-
49.
Rigano D, Formisano C, Basile A, Lavitola A, Senatore F, Rosselli S, Bruno M. Antibacterial activity of flavonoids and phenylpropanoids from Marrubium globosum ssp Libanoticum. Phytother. Res. 2007;21:395-397. [PubMed ID: 17186490].
-
50.
Jung M, Park M, Lee HC, Kang YH, Kang ES, Kim SK. Antidiabetic agents from medicinal plants. Curr. Med. Chem. 2006;13:1203-18. [PubMed ID: 16719780].
-
51.
Kong CS, Seo Y. Antiadipogenic activity of isohamnetin 3-O-β-D-glucopyranoside from Salicornia herbacea. Immunopharm. Immunot. 2012;34:907-911.
-
52.
Baser KHC, Buchbauer G. Handbook of Essential Oils: Science, Technology, and Applications. Florida: CRC Press; 2009. p. 60-70.