Abstract
Keywords
Naja Naja Immunodominant protein Antibacterial effect Electroelution
Introduction
Snake venom is a complex mixture of peptides, proteins, different enzymes including phospholipases, oxidases, nucleosidase, ribonuclease, carbohydrate, and mineral that contain a variety of chemicals namely pharmacological and toxicological proteins. Antibacterial and anticancer peptides and proteins produced by snake venom have recently attracted attention for the purpose of identifying new therapeutic agents. The first reports about antibacterial effects of snake venoms were documented (1948 and 1968) for Elapidae and Viperidae (1, 2). Snake venoms of the Viperidae family have antibacterial effects against Sarcina species, while a lytic factor or cytotoxin found in Naja species, was composed of a basic low-molecular-weight protein. Also Hemachatus haemachatus venom has been shown to have antibacterial activity; these venoms kill Escherichia coli (3). Several antibacterial studies on Crotalidae (4, 5), Elapidae and Viperidae (6, 7) snake venoms have also been mentioned in the literature to have antibacterial activity against Gram-negative and Gram-positive bacteria. In addition, several studies have reported the antibacterial effects of proteins extracted from Pseudechis australis (Australian King Brown) (7).
Accordingly, previous studies have demonstrated that L-amino acid oxidase (8, 9), lectin (10) and phospholipase A2 (11) have antibacterial components. Antimicrobial agents inhibit the growth of microorganisms at low concentrations. These compounds include antibiotics which are naturally occurring substances from venoms and microorganisms, as well as chemically-synthesized substances. Therefore, antibiotics that are produced from venoms and microorganisms can be used to treat bacterial diseases instead of chemotherapeutics (12). Antibacterial proteins are widely expressed in snake venoms (13, 14). A variety of snake venom proteins and peptides have been investigated in previous studies and they have demonstrated that these antibacterial proteins and peptides are highly active in killing a wide range of bacteria (14-16). In the present study, the antibacterial effect of isolated immunodominant proteins (14, 22, 32, and 65kDa) from the venom of Naja Naja Oxiana snake was tested against several strains of clinical isolates including S.aureus, B.subtilis (Gram-positive bacteria) P.aeruginosa, and E.coli (Gram-negative bacteria) using broth microdilution and disc-diffusion assays.
Materials and Methods
Lyophilized crude venom of Naja Naja (Oxiana) was a kind gift from poisonous animal Dept.Razi Vaccine and serum research Institute, Karaj –Iran.
The serum of hyper-immunized horses was provided by Razi Institute, Karaj-Iran
Rabbit Anti-horse – IgG (whole molecule) – peroxidase conjugate, was prepared from sigma.
Micro-organisms
The Gram-positive bacteria including Bacillus subtilis (ATCC 6633) and Staphylococcus aureus (ATCC 6538) and Gram-negative bacteria including Escherichia coli (ATCC 25922) and Pseudomonas aeruginosa (ATCC 97853) were obtained from RTCC (Razi Type Culture Collection)
Standard Antibiotics
Ampicillin (10 μg), Gentamicin (10 μg), Penicillin (10 μg), Ciprofloxacin (5 μg), and Amoxiclav (25 μg)
Chemical Reagents
Ammonium persulfate (APS), Tween 20, Acrylamide, SDS and TEMED were obtained from Bio-Rad (USA); phosphate buffered salt (PBS) was acquired from Merck, Bovine serum albumin (BSA), chloro-1-naphthol from Fluka, and hydrogen peroxidas were obtained from Sigma.
Determination of protein concentration
Protein concentration was determined by the method of Lowry using BSA as standard (17).
SDS-PAGE
Electrophoresis was carried out on a 15% polyacrylamide gel by the method of laemmli (18). Crude venom (20 μg) was mixed with an equal volume of sample buffer and molecular weight marker separately loaded into the wells. The wells were filled with running gel buffer. The output voltage was 110V for 75 min. After the run, the gel was stained with Coomassie Brilliant Blue R-250 (sigma) and then it was distained. Molecular weights of proteins of crude venom were estimated according to the bands pattern of obtained marker proteins.
Western Blotting
The proteins of venom were denatured, separated on 15% SDS-PAGE gel, and transferred onto nitrocellulose membrane (sigma) by semi-dry transfer cell (Bio-Rad, Hercules, CA). The membrane was blocked with 3% bovine serum albumin (BSA) and incubated at room temperature for 90 min. The membrane was washed with several changes of PBST (phosphate buffered salt tween) buffer and incubated with diluted horse antivenom solution (1:20 diluted with PBST buffer) at 4 °C overnight. Then the membrane was washed with several changes of PBST buffer and incubated with appropriate HRP-conjugated rabbit anti-horse secondary antibody (1:10,000 dilution with PBST buffer) for 90 min at room temperature. After washing off unbound secondary antibody with PBST buffer, the bonds were visualized with substrate buffer (18 mg 4-chloro-1-naphthol (Fluka)), 24 mL PBS and 3 μL hydrogen peroxidase (4).
Isolation of immunodominant proteins identified by Western Blot Electro-elution
In the present study, Bio-Rad model 422 electro-eluter was used to recover 4 sharp bond immunodominant proteins that were identified by western blot and separated by SDS-PAGE electrophoresis.
Briefly, after running a standard 15% preparative gel electrophoresis, the gel was stained with Coomassie Blue to visualize the protein bands. The expected (14, 22, 32, and 65kDa) protein bands were cut out and diced into smaller slices and placed into 6 tubes. The tubes were filled with elution buffer (Tris/Glycin buffer was also used for gel electrophoresis), then, silicone adaptors were filled with elution buffer and checked for leakage and air bubbles; then, the lead was attached and the elution conditions were set (8 to 10 mA/glass tube) for 5h, on a magnetic stirrer.
The per chamber was removed and the eluted proteins were pipetted from the membrane. Next, they were dialyzed in elution buffer without SDS (overnight at 4 °C) in order to remove SDS from isolated proteins; then it was concentrated with PEG.
Disc Diffusion
Disc-diffusion assay was performed by the published method (Bauer et al., 1966). Pure cultures were prepared by sub culturing the test strain into 10 mL of Brain Heart Infusion broth (BHI broth) (Merck), following incubation at 37 °C for 24 h. The culture media was diluted and adjusted to 0.5 McFarland standard, containing 1.5x108 cfu ml -1, in order to inoculate the same dose of bacteria when repeating the experiment. The absorbance of the cultured media was also determined at 600 nm, using a spectrophotometer apparatus (Jenway 6105, Essex, England).
Sterile blank paper discs (7 mm in diameter) were then placed on MH agar surface and 40 µL of isolated immunodominant proteins (14, 22, 32, and 65 kDa) were added per disc in four replicates. Antibiogram discs including, Ampicillin (10 μg), Gentamicin (10 μg), Penicillin (10 μg), Ciprofloxacin (5 μg), and Amoxiclav (25 μg) were used as positive controls. The plates were incubated at 37 °C for 16-18h and the zones of inhibition were measured. The experiments were performed at least in four replicates.
Broth Microdilution Assay
Broth microdilution assay was determined according to the protocol explained by the NCCLS, National Committee for Clinical Laboratory Standards (2000).
Bacteria suspensions were cultured in Muller-Hinton broth at 37 °C. The inoculum suspension was adjusted to the density of 0.5 McFarland (1.5× 108 cfu/mL). Bacterial suspensions (50 μL) were incubated in a 96-well plate in the presence of 50 μL of immunodominant proteins (14kDa ranging from 600 to 4.68 μg/mL), 22 kDa ranging from 380 to 2.96 μg/mL, 32kDa ranging from 350 to 2.68 μg/mL and 65 kDa from 500 to 3.87 μg/mL, using serial dilutions. The plates were incubated at 37 °C for16 -18 h. The MIC was defined as the lowest concentration of protein that visibly inhibited bacterial growth (two assays for each). The plates were read at 620 nm in a microplate reader (Epoch-Bio TeK) after being incubated overnight (~ 16 h) at 37 °C.
Results
Determination of Protein Concentration
Protein concentration of crude Naja Naja venom was determined 16 mg/mL by BSA standard protein using the Lowry method.
SDS-PAGE
Figure 1. shows the results of SDS-PAGE (15%), which determined the molecular weight of proteins of Naja Naja venom using the protein ladder (14, 20, 21.2, 22, 25, 32, 34, 37, 50, 65, 75, and 150 kDa).
Western Blot Analysis
Western blot analysis on antigen at the dilution 1:20 with serum hyper- immunized- horse, Figure (2) shows 4 sharp bands as (14, 22, 32, and 65 kDa) molecular weights.
Evaluation of Isolation of 14, 22, 32, and 65 kDa immunodominant proteins After Electro-Elution
The Electro- elution method was used for isolation of 14, 22, 32, and 65 kDa immunodominant proteins. Electro-elution of proteins from gel is the method used to recover proteins resolved by electrophoresis in polyacrylamide gel by transferring the protein molecules out of gel by means of an electric field (18, 19). Only 4 protein bonds with 14, 22, 32, and 65 kDa molecular weights were manifested in the coomassie staining gel of isolated proteins achieved from Electro-elution. Then, the concentrations of isolated proteins (14, 22, 32, and 65 kDa) were determined respectively as 1.3, 0.7, 0.7, and 1 mg/mL by the Lowry assay.
Immunodominant proteins were homogeneous as judged by SDS-PAGE, and respectively, with molecular mass of Acidic phospholipase A2 4 (14,015 Da with AC.no:Q6T179 and 16,013 kDa , AC.no: P00598), Cytochrome C oxidase subunit 1(COI) (22,915 Da ,AC.no: A0A0A7PA27), Snake venom serine protease (Nasp) (31,137 Da , AC.no A8QL53), Zinc metalloproteinase-disintegrin-like (K-like and atragin) (66,292 Da with AC.no D3TTC1 and 69,180Da ,AC.no D3TTC2) in Uniprot protein database (NCBI research) in agreement with previous reports (20, 21) .
Disc Diffusion
Table 1. shows antibacterial activity of four crude isolated immunodominant proteins of N. najaOxiana (14, 22, 32 and 65 kDa) and also different antibiotics, against Gram-negative bacteria including E.coli and Pseudomonas aeruginosa and Gram-positive bacteria including Bacillus subtilis and Staphylococcus aureus on MH agar plate which produced inhibitory zones. These are important for the defensive mechanism of innate immunity system.
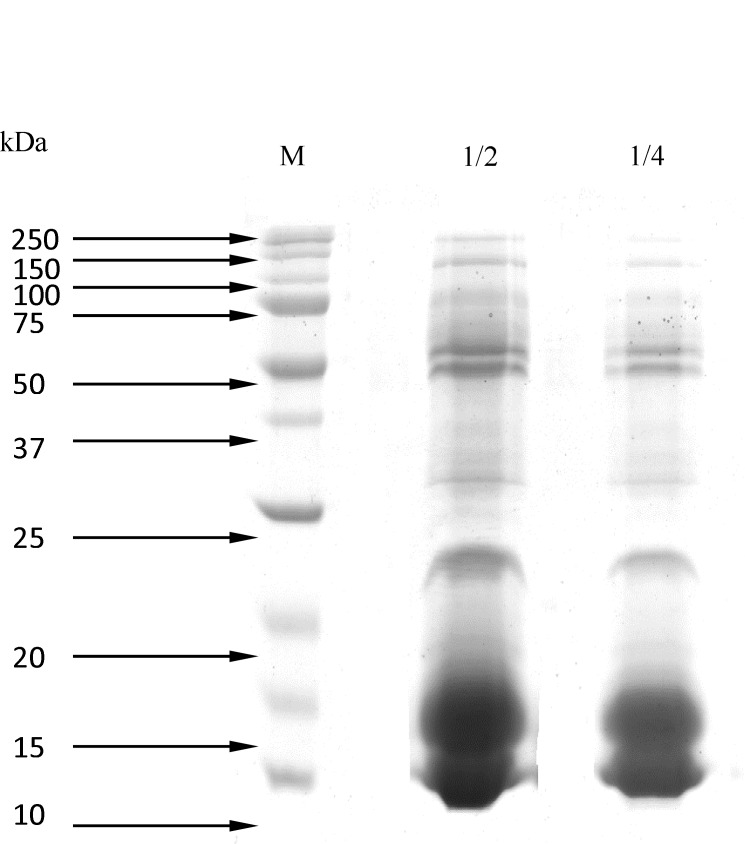
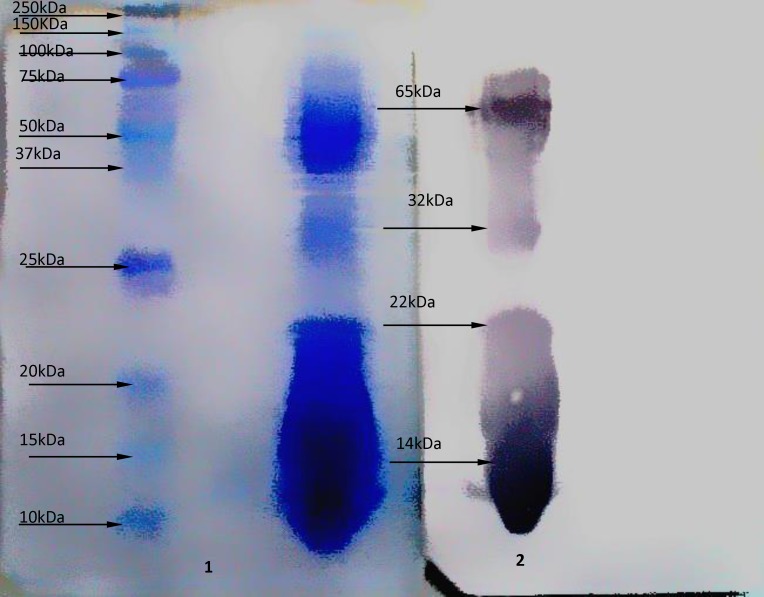
Antibacterial activity of immunodominant proteins crude isolated of N.naja oxiana venom tested by disc-diffusion and compared to some standard antibiotics. Each number is presented as mean + SD of inhibition zone in mm,(n = 4
Microorganism | Pseudomonas aeruginosa | E.coli | B. subtilis | S. aureuss |
---|---|---|---|---|
Immunodominant protein 0f 14 kDa | 10/83 ± 0/28 | 10/66 ± 0/28 | 16/5 ± 0/5 | 13/16 ± 0/28 |
Immunoominant protein of 22 kDa | 6.7 + 0.64 | 7/1 ± 0/36 | 7/1 ± 0/36 | 10/13 ± 0/32 |
Immunodominant protein of 32 kDa | 6/93 ± 0/11 | 7/6 ± 0/52 | 8/1 ± 0/36 | 12/73 ± 0/25 |
Immunodominant protein of 65 kDa | 7/6 ± 0/52 | 7/43 ± 0/40 | 15/76 ± 0/25 | 14/5 ± 0/5 |
Ampicillin | 0.0 + 0.0 | 8/26 ± 0/55 | 19/26 ± 0/64 | 24/13 ± 0/41 |
Gentamicin | 18 ± 0/2 | 19/04 ± 0/16 | 35/88 ± 0/34 | 16/13 ± 0/20 |
Penicillin | 0.0 + 0.0 | 0.0 + 0.0 | 9/13 ± 0/32 | 0.0 + 0.0 |
Ciprofloxacin | 0.0 + 0.0 | 35/66 ± 0/57 | 30/88 ± 0/34 | 24/02 ± 0/13 |
Amoxiclav | 0.0 + 0.0 | 14/27 ± 0/26 | 18/46 ± /57 | 20 ± 0/8 |
Antibacterial activities of isolated immunodominant proteins of Naja Naja Oxiana
Bacteria | Immunodominant Protein name/Standard Antibiotics | MIC(μg/mL) |
---|---|---|
Gram-negative | ||
Escherichia Coli | Immunodominant protein 0f 14 kDa | 75 |
Immunoominant protein of 22 kDa | >190 | |
Immunodominant protein of 32 kDa | >175 | |
Immunodominant protein of 65 kDa | ≥125 | |
Ampicillin | 120 | |
Gentamicin | <10 | |
Penicillin | >200 | |
Ciprofloxacin | <5 | |
Amoxiclav | 25 | |
Pseudomonas aeruginosa | Immunodominant protein 0f 14 kDa | 75 |
Immunoominant protein of 22 kDa | >190 | |
Immunodominant protein of 32 kDa | >175 | |
Immunodominant protein of 65 kDa | ≥125 | |
Ampicillin | >200 | |
Gentamicin | <10 | |
Penicillin | >200 | |
Ciprofloxacin | >200 | |
Amoxiclav | >200 | |
Staphylococcus aureus | Immunodominant protein 0f 14 kDa | 37.5 |
Immunoominant protein of 22 kDa | >190 | |
Immunodominant protein of 32 kDa | 87 | |
Immunodominant protein of 65 kDa | 26 | |
Ampicillin | 25 | |
Gentamicin | 10 | |
Penicillin | >200 | |
Ciprofloxacin | <5 | |
Amoxiclav | 10 | |
Bacillus subtilis | Immunodominant protein 0f 14kDa | 37.5 |
Immunoominant protein of 22 kDa | >190 | |
Immunodominant protein of 32kDa | >175 | |
Immunodominant protein of 65 kDa | 31 | |
Ampicillin | 25 | |
Gentamicin | 10 | |
Penicillin | 120 | |
Ciprofloxacin | <5 | |
Amoxiclav | 25 |
Broth Microdilution Assay of Four Identified Immunodominant Proteins
The MIC values of these immunodominant proteins, also of standard antibiotics, were calculated against four bacteria. Table 2. shows these results.
Discussion
Previous studies have demonstrated that a variety of snake venoms have antibacterial effects and are highly active in killing a wide range of bacteria (14-16). Generally, antibacterial proteins are cationic in nature (22) and it is believed that they exert their bactericidal effect through permeabilization of bacterial membranes via a thinning process (23, 24) that involves the destabilization of the membrane bilayer (25). On the other hand, bactericidal mechanism used by anionic antimicrobial proteins against Gram-positive bacteria such as S. aureus obviously involves interactions with the membrane lipid head group region (24). In addition to membrane permeabilization, antibacterial proteins, and peptides kill bacteria by inhibition of macromolecular biosynthesis (26) and interacting with specific basic components inside the bacteria (27). Some studies have suggested that induction of autolytic enzymes by peptides and proteins may be associated with bacterial cell death (28). The mechanisms of bactericidal and membrane damaging effects were confirmed ultrastructrually (29). Previous studies have reported a proteinase from Agkistrodon halys venom of viper family that inhibits the growth of S.aureus (30) and other drug-resistant human pathogens (31). Reported the MICs of basic myotoxic PLA2 (EcTx-I) from Echis carinatus venom was (15g/mL) against Burkholderia pseudomallei (KHW) and 30 g/mL against Enterobacter aerogenes. Also, MIC of Daboia russelli russelli (DRR-PLA2) against S. aureus was reported to be 62.3 g/mL (21) and for a viper metalloprotein at comparatively lower concentrations against S. aureus (MIC, 7.5 μM ) (31). Also antibacterial effects of L-amino acid oxidase in the venom of Cobra and Viper have been investigated against Gram-positive and Gram-negative bacteria. This enzyme with high molecular weight has been proved to have a strong antibacterial effect (4). Mui Li Lee et.al , 2011 (32) isolated LAAO (L-amino acid oxidase ) 65 kDa from the venom of King Cobra (Ophiophagus hannah) and have demonstrated that LAAO has higher antibacterial effect against Gram-positive bacteria compared to Gram-negative bacteria. In contrast, galactose binding lectin (33), phospholipase A2 (4, 21, 34), acuthrobin-C (35), onwaprin, and 50-amino acid cationic protein (35)that were extracted from snake venoms were low molecular weight proteins (10-17 KDa) with strong antibacterial activities. In addition, Hsuan-Wei Hung et al., 2015 (20), have reported the molecular weight of CTXs (8980-9323 Da), acidic phospholipase A2 (PLA2s 16,013 Da) and Zinc metalloproteinase-disintegrin-like (K-like) and atragin (SVMP 66,292 Da and 69,180 Da), respectively, extracted from Naja atra with high immunogenicity and also high immunoreactivity to immunize mice against snakebite of individual Naja atra snake venom. But they did not investigate the antibacterial effects of these proteins. Most authors have not compared the antibacterial activity of the immunodominant proteins with common antibiotics, as well as their MICs against Gram-positive and Gram-negative bacteria. Hence, in the present study, cobra (Naja Naja Oxiana), the only major species of snakes found in the North-east of Iran, was chosen and immunogenicity and antibacterial effects of its immunodominant proteins were investigated. The MICs of immunodominant protein of 14kDa against S. aureus and B. subtilis were 37.5 µg/mL. The MICs against E.coli and P.aeruginosa were 75µg/mL. The MICs of immunodominant protein of 22kDa against S. aureus, B. subtilis, E.coli, and P. aeruginosa were >190 µg/mL. The MICs of immunodominant protein of 32 kDa against E.coli, P. aeruginosa and B. subtilis were >175µg/Ml and against S. aureus it was 85µg/mL. The MICs of immunodominant protein of 65 kDa against E.coli and P.aeruginosa were ≥125 µg/mL and against S. aureus and B. subtilis the MICs were 26 µg/mL and 31 µg/mL, respectively. The MICs of immunodominant proteins of 14 kDa and 65 kDa against the tow Gram-positive bacteria were comparable to the antibiotics tested, except for Ciprofloxacin, which was far more potent than the immunodominant proteins. Also the results of disc diffusion showed that the antibacterial effect of immunodominant protein of 14 kDa against P. aeruginosa was more than Ampicillin, Penicillin, Ciprofloxacin, and Amoxiclav, and against E.coli it was more than Ampicillin and penicillin, and almost the same as Amoxiclav. Antibacterial effect of immunodominant protein of 14 kDa against B. subtilis was more than penicillin and almost the same as Ampicillin and Amoxiclav. Also antibacterial effect against S. aureus was almost the same as Gentamicin. Antibacterial effects of immunodominant proteins of 22 kDa and 32 kDa against S. aureus was more than penicillin. Antibacterial effect of immunodominant protein of 65 kDa against B. subtilis was almost the same as Ampicillin and Amoxiclav; the effect was almost the same as Gentamicin and more effective than Penicillin against S. aureus. Our results on antibacterial activities of these proteins with small molecular weights ≤ 65 kDa, firmly confirmed the findings of previous studies that immunodominant proteins of 14, and 65 kDa, with high immunogenicity and also high immunoreactivity were very effective in inhibiting the two Gram-positive bacteria (S.aureus, B.sub). However these proteins were only slightly effective against the two Gram-negative bacteria of P.aeruginosa and E.coli. Immunodominant proteins of 22 kDa and 32 kDa with high immunogenicity showed a slight antibacterial activity against the Gram-positive and Gram-negative bacteria in comparison with the other immunodominant proteins. Therefore, the proteins 22 kDa and 32 kDa that has high immunogenicity, cannot have high antibacterial activity. In addition, the results (Tables 1 & 2) showed that immunodominant proteins have more potent antimicrobial effects against the Gram-positive bacteria (S.aureus, B.subtilis) than the Gram-negative bacteria (E.coli, P.aeruginosa). We hope that this study will be useful in finding some alternative agents for drug-resistant microorganisms.
Conclusion
In the present study four proteins of high immunogenicity with molecular weights of 14, 22, 32, and 65 kDa were identified. Two novel antibacterial immunodominant proteins of 14 kDa and 65 kDa with high immunogenicity and high antibacterial activity against Gram-positive bacteria and with slightly antibacterial effect against Gram-negative bacteria (see Table 1 and 2) and two novel immunodominant proteins of 22 kDa and 32 kDa, with high immunogenicity but slight antibacterial activity against the Gram-positive bacteria and Gram-negative bacteria were identified.
It was shown that the antibacterial activities of immunodominant proteins of 14 kDa and 65 kDa were comparable to Penicillin and Gentamicin against S.aureus. The effects were comparable to penicillin, Ampicillin, and Amoxiclav against B.subtilis.
This is a simple and economic method for preparing suitable antibacterial immundominant proteins from individual venom components.
Acknowledgements
References
-
1.
Aloof-Hirsch S, De Vries A, Berger A. The direct lytic factor of cobra venom: purification and chemical characterization. Biochimica et Biophysica Acta (BBA)-Protein Structure. 1968;154:53-60.
-
2.
Glaser HR. Bactericidal activity of Crotalus venom in-vitro. Copeia. 1948:245-7.
-
3.
Stocker JF, Traynor J. The action of various venoms on Escherichia coli. Journal of applied bacteriology. 1986;61:383-8. [PubMed ID: 3542922].
-
4.
Samy RP, Pachiappan A, Gopalakrishnakone P, Thwin MM, Hian YE, Chow VT, Bow H, Weng JT. In-vitro antimicrobial activity of natural toxins and animal venoms tested against Burkholderia pseudomallei. BMC Infectious Diseases. 2006;6:1. [PubMed ID: 16390553].
-
5.
Talan DA, Citron DM, Overturf GD, Singer B, Froman P, Goldstein EJ. Antibacterial activity of crotalid venoms against oral snake flora and other clinical bacteria. Journal of Infectious Diseases. 1991;164:195-8. [PubMed ID: 2056205].
-
6.
Blaylock RS. Antibacterial properties of KwaZulu Natal snake venoms. Toxicon. 2000;38:1529-34. [PubMed ID: 10775753].
-
7.
Stiles BG, Sexton FW, Weinstein SA. Antibacterial effects of different snake venoms: purification and characterization of antibacterial proteins from Pseudechis australis (Australian king brown or mulga snake) venom. Toxicon. 1991;29:1129-41. [PubMed ID: 1796476].
-
8.
Chippaux JP. Snake-bites: appraisal of the global situation. Bulletin of the World Health organization. 1998;76:515. [PubMed ID: 9868843].
-
9.
Kini RM, Evans HJ. Effects of snake venom proteins on blood platelets. Toxicon. 1990;28:1387-422. [PubMed ID: 2089737].
-
10.
Warrell DA. Guidelines for the management of snake-bites.Guidelines for the management of snake-bites. 2010.
-
11.
Barker RA, Ratcliffe E, Mclaughlin M, Richards A, Dunnett SB. A role for complement in the rejection of porcine ventral mesencephalic xenografts in a rat model of Parkinson›s disease. The Journal of Neuroscience. 2000;20:3415-24. [PubMed ID: 10777804].
-
12.
Cromwell G. Antimicrobial and promicrobial agents. Swine nutrition. 2001;2:401-26.
-
13.
Perumal Samy R, Gopalakrishnakone P, Thwin M, Chow T, Bow H, Yap E, Thong T. Antibacterial activity of snake, scorpion and bee venoms: a comparison with purified venom phospholipase A2 enzymes. Journal of applied microbiology. 2007;102:650-9. [PubMed ID: 17309613].
-
14.
Toyama MH, de Oliveira DG, Beriam LO, Novello JC, Rodrigues-Simioni L, Marangoni S. Structural, enzymatic and biological properties of new PLA 2 isoform from Crotalus durissus terrificus venom. Toxicon. 2003;41:1033-8. [PubMed ID: 12875878].
-
15.
Samy RP, Gopalakrishnakone P, Ho B, Chow VT. Purification, characterization and bactericidal activities of basic phospholipase A 2 from the venom of Agkistrodon halys (Chinese pallas). Biochimie. 2008;90:1372-88. [PubMed ID: 18472013].
-
16.
Nair DG, Fry BG, Alewood P, Kumar PP, Kini RM. Antimicrobial activity of omwaprin, a new member of the waprin family of snake venom proteins. Biochemical Journal. 2007;402:93-104. [PubMed ID: 17044815].
-
17.
Lowry OH, Rosebrough NJ, Farr AL, Randall RJ. Protein measurement with the Folin phenol reagent. J biol Chem. 1951;193:265-75. [PubMed ID: 14907713].
-
18.
Cutler P. Protein purification protocols. Vol. 244. Springer Science & Business Media; 2004.
-
19.
Walker JM. The protein protocols handbook. Vol. 1996. Springer Science & Business Media; 1996.
-
20.
Huang H-W, Liu B-S, Chien K-Y, Chiang L-C, Huang S-Y, Sung W-C, Wu W-G. Cobra venom proteome and glycome determined from individual snakes of Naja atra reveal medically important dynamic range and systematic geographic variation. Journal of proteomics. 2015;128:92-104. [PubMed ID: 26196238].
-
21.
Oliveira D, Toyama M, Novello J, Beriam L, Marangoni S. Structural and functional characterization of basic PLA2 isolated from Crotalus durissus terrificus venom. Journal of protein chemistry. 2002;21:161-8. [PubMed ID: 12018617].
-
22.
Zasloff M. Antimicrobial peptides of multicellular organisms. nature. 2002;415:389-95. [PubMed ID: 11807545].
-
23.
Heller WT, Waring AJ, Lehrer RI, Harroun TA, Weiss TM, Yang L, Huang HW. Membrane thinning effect of the β-sheet antimicrobial protegrin. Biochemistry. 2000;39:139-45. [PubMed ID: 10625488].
-
24.
Gazit E, Boman A, Boman HG, Shai Y. Interaction of the mammalian antibacterial peptide cecropin P1 with phospholipid vesicles. Biochemistry. 1995;34:11479-88. [PubMed ID: 7547876].
-
25.
Dennison SR, Howe J, Morton LH, Brandenburg K, Harris F, Phoenix DA. Interactions of an anionic antimicrobial peptide with Staphylococcus aureus membranes. Biochemical and biophysical research communications. 2006;347:1006-10. [PubMed ID: 16857163].
-
26.
Yenugu S, Hamil KG, French FS, Hall SH. Antimicrobial actions of the human epididymis 2 (HE2) protein isoforms, HE2alpha, HE2beta1 and HE2beta2. Reproductive Biology and Endocrinology. 2004;2:1. [PubMed ID: 14711378].
-
27.
Otvos L, O I, Rogers ME, Consolvo PJ, Condie BA, Lovas S, Bulet P, Blaszczyk-Thurin M. Interaction between heat shock proteins and antimicrobial peptides. Biochemistry. 2000;39:14150-9. [PubMed ID: 11087363].
-
28.
Sahl H-G, Pag U, Bonness S, Wagner S, Antcheva N, Tossi A. Mammalian defensins: structures and mechanism of antibiotic activity. Journal of leukocyte biology. 2005;77:466-75. [PubMed ID: 15582982].
-
29.
Samy RP, Gopalakrishnakone P, Bow H, Puspharaj PN, Chow VT. Identification and characterization of a phospholipase A 2 from the venom of the Saw-scaled viper: Novel bactericidal and membrane damaging activities. Biochimie. 2010;92:1854-66. [PubMed ID: 20723574].
-
30.
Samy RP, Gopalakrishnakone P, Chow VT, Ho B. Viper metalloproteinase (Agkistrodon halys pallas) with antimicrobial activity against multi-drug resistant human pathogens. Journal of cellular physiology. 2008;216:54-68. [PubMed ID: 18297685].
-
31.
Van den Bosch H. Intracellular phospholipases A. Intracellular phospholipases A. Biochimica et Biophysica Acta (BBA)-Biomembranes. 1980;604:191-246. [PubMed ID: 6252969].
-
32.
Lee ML, Tan NH, Fung SY, Sekaran SD. Antibacterial action of a heat-stable form of L-amino acid oxidase isolated from king cobra (Ophiophagus hannah) venom. Comparative Biochemistry and Physiology Part C: Toxicology & Pharmacology. 2011;153:237-42. [PubMed ID: 21059402].
-
33.
Lomonte B, Rojas G, Gutiérrez J, RAMiREZ G. Isolation of a galactose-binding lectin from the venom of the snake Bothrops godmani (Godmann›s pit viper). Toxicon. 1990;28:75-81. [PubMed ID: 2109909].
-
34.
Gao W, Starkov VG, Tsetlin VI, Utkin YN, Lin Z-j, Bi R-c. Isolation and preliminary crystallographic studies of two new phospholipases A2 from Vipera nikolskii venom. Acta Crystallographica Section F: Structural Biology and Crystallization Communications. 2005;61:189-92. [PubMed ID: 16510990].
-
35.
Huang Q-q, Teng M-k, Niu L-w. Purification and characterization of two fibrinogen-clotting enzymes from five-pace snake (Agkistrodon acutus) venom. Toxicon. 1999;37:999-1013. [PubMed ID: 10484747].