Abstract
Keywords
Introduction
Insulin resistance (IR) is a state that body tissues have less sensitivity to insulin and as a result, downstream metabolic pathways that are regulated by insulin are impaired and blood glucose rises (1). It is a prevalent metabolic disorder in industrialized countries and mainly associated with glucose intolerance, overt hyperglycemia, hyperinsulinemia and hypertension, a complex state that is referred to as metabolic syndrome (1, 2).
Numerous factors have been identified to be associated with insulin resistance and type 2 diabetes mellitus. Obesity has a strong association with type 2 diabetes mellitus and this link is mediated by induction of insulin resistance (3). In obesity, adipocyte stress and hypertrophy and the resulting increase in proinflammatory adipocytokines (IL.6 and TNFα) contribute to the development of insulin resistance (4, 5).
Adiponectin is the most abundant adipocytokine that is secreted from adipose tissue and has an inverse correlation with body fat. Plasma adiponectin is decreased in insulin resistance and type 2 diabetes mellitus (6). Adiponectin increases insulin sensitivity by decreasing hepatic gluconeogenesis and lipid storage (7).
Increased plasma free fatty acid is another key factor in the induction of insulin resistance. Free fatty acids affect numerous mechanisms such as activation of inflammatory pathways, alteration in phosphorylation of signaling molecules and synthesis of lipid intermediate (eg. diacylglycerol) (8-10).
Thiazolidinedione’s (e.g. pioglitazone) are agonists of PPAR.γ and are used to sensitize the body to respond to insulin (11). Upon binding its ligand, PPAR.γ sits on Peroxisome Proliferator Response Elements on the DNA and changes the expression of target genes which ultimately result in increased insulin sensitivity (12).
Discovering new agents for treatment of type 2 diabetes mellitus is highly demanded and plants are a potential useful source for this purpose. In our previous study we reported that rosa- damascena-flower-extract has an inhibitory effect on Alpha glucosidase and therefore decreases postprandial blood glucose level in normal and diabetic rats after administration of maltose (1 g/kg body weight( (13). However, in further studies (data not published) we also found that this extract inhibited the elevation of postprandial blood glucose after administration of Glucose, a sugar which is not a substrate for Alpha glucosidase We also found that the extract diminished the fasting blood glucose after administration for 15 days in diabetic rats (results not published). For this reason we designed this study to investigate the mechanism (s). Through which rosa damascena extract affects blood glucose level
Experimental
Animals
Insulin resistant rats were used to study insulin secretion, insulin resistance, adiponectin and free fatty acid levels and Glucose Transporter 4 (GLUT.4) and Peroxisome proliferator activated receptor γ (PPAR.γ) gene expression.
Plant extract
Flowers of Rosa damascena were collected from Lalehzar, Keman province, Iran. Air dried flowers (300 g) were milled and extracted by maceration method in 1000 mL of methanol at room temperature for 48 h. After filtration, the extract was concentrated by a rotary evaporator at 40 °C and dried in a 40 °C oven. Dried extract was stored at -20 °C.
Animal treatment
Male Wistar rats weighing 270–280 g were obtained from the animal house of Kerman University of Medical Sciences. Rats were housed in cages (four animals per cage) at 22 ± 3 ◦C with 12 h of light. Rats were fed with standard chow and fresh tap water for 2 weeks. After 2 weeks, rats were divided randomly into 4 groups (n=8). Three groups were fed a 60% fructose diet and the fourth group as the healthy control group (HCG) was fed with standard diet (14). After 6 weeks, insulin resistance in fructose fed rats was confirmed by oral glucose tolerance test (OGTT) (results not shown). Animals were treated by Rosa damascena extract (100 mg/Kg) (13) and Pioglitazone (10 mg/Kg) (15). and were compared with two control groups: healthy control (fed on standard chow) and insulin resistant control (were fed a high fructose diet)
Animals were treated by intragastric injection and treatment was continued for 2 weeks. Water and food intake were measured daily during this period. Body weight was also measured weekly throughout the 8 weeks of treatment.
primers used in this study for real time PCR
Accession number | PCR product | primers | Gene |
---|---|---|---|
NM_012751 | 106 | F: ACTGGCGCTTTCACTGAACT | GLUT.4 |
R: CGAGGCCAAGGCTAGATTTTG | |||
NM_001145367 | 131 | F: CATGCTTGTGAAGGATGCAAG | PPAR.γ |
R: TTCTGAAACCGACAGTACTGACAT | |||
NM_017008 | 138 | F: TGGAGTCTACTGGCGTCTT | GAPDH |
R: TGTCATATTTCTCGTGGTTCA |
Effect of rosadamascenaon on body weight, water, food intake and other insulin resistance related parameters.
parameter | groups | P-value¥ | |||
---|---|---|---|---|---|
HCG | Con | Pio | RDE | ||
weight in 6 week (g) | 283±9 | 285±8 | 292±13 | 286±12 | 0.986 |
weight in 8 week (g) | 285±9 | 307±8 | 294±16 | 275±9 | 0.042 |
Weight gain (g) | 2±1.54* | 22.25±11.49# | 2.17±0.52# | -10.75±5.81* | <0.0001 |
Water intake (mL) | 35±0.8* | 47±2# | 60±2# * | 56±1.2# | 0.151 |
Food intake (g) | 21.6±0.7* | 13.4±0.4# | 13.5±0.3# | 12.8±0.1# | 0.978 |
Insulin (pmol/L) | 50±4.8* | 137±34# | 40±2.7* | 42±2.7* | <0.0001 |
Adiponectin (μg/mL) | 2.9±0.16 | 3.9±0.15 | 5.6±0.4# * | 5.6±0.17 * # | <0.0001 |
Glucose (mg/dL) | 132±4* | 187±15# | 129±5.8* | 129±4.7* | 0.002 |
HOMA.IR | 2.7±0.37* | 9.7±2.1# | 2.1±0.12* | 2.2±0.18* | <0.0001 |
Cholesterol (mg/dL) | 71±12.1 | 59±3.2 | 63±4.2 | 55±3.18 | 0.986 |
Triglyceride (mg/dL) | 85±13* | 217±18# | 200±51# | 75±9 * | <0.0001 |
Effect of rosa damascenaon on plasma free fatty acids profiles
parameter | groups | *P-value | |||
---|---|---|---|---|---|
HCG | Con | Pio | RDE | ||
myristic acid (μmol/L) | 1.07±0.01 | 1.12±0.06 | 1.2±0.06 | 1.33±0.1 | 0.983 |
palmitic acid (μmol/L) | 5.9±0.57 | 11.1±2.6 | 5.1±0.18 | 9.39±0.82 | 0.034 |
palmitoleic acid (μmol/L) | 1.06±0.04 | 1.4±0.12 | 1.1±0.01 | 1.59±0.49 | 0.936 |
stearic acid (μmol/L) | 1.3±0.12 | 2.7±0.12 | 2.06±0.11 | 2.2±0.23 | 0.837 |
oleic acid (μmol/L) | 2.2±0.21 | 2.9±0.22 | 1.7±0.08 | 2.82±0.69 | 0.996 |
total free fatty acids (μmol/L) | 12.53±1.95 | 22±2.7 | 15±2.3 | 21.82±0.94 | 0.999 |
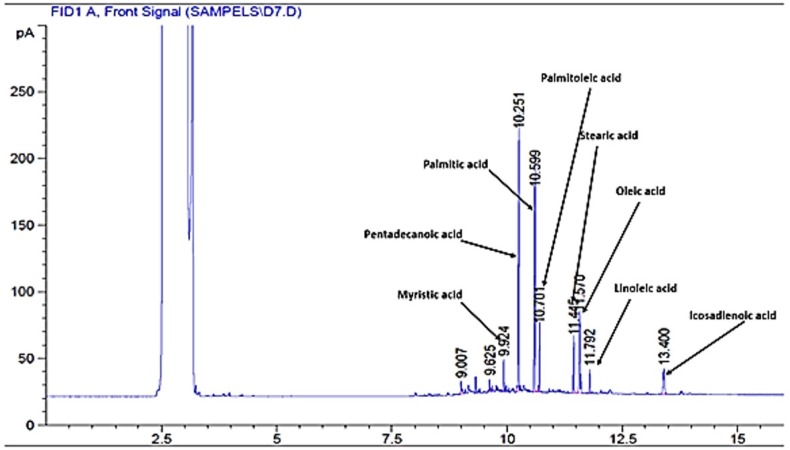
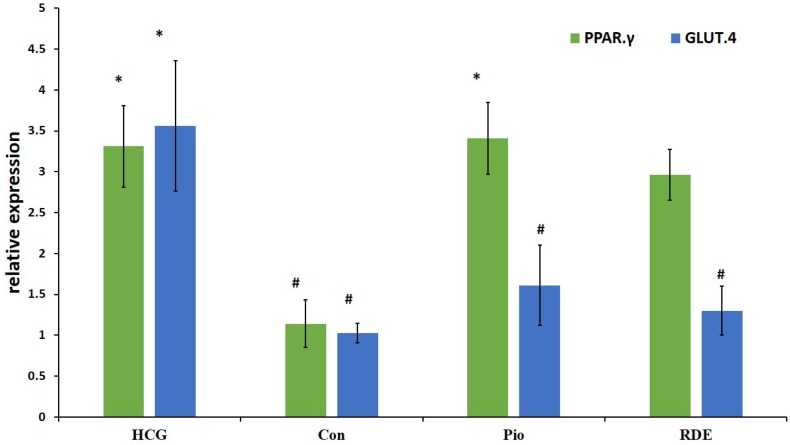
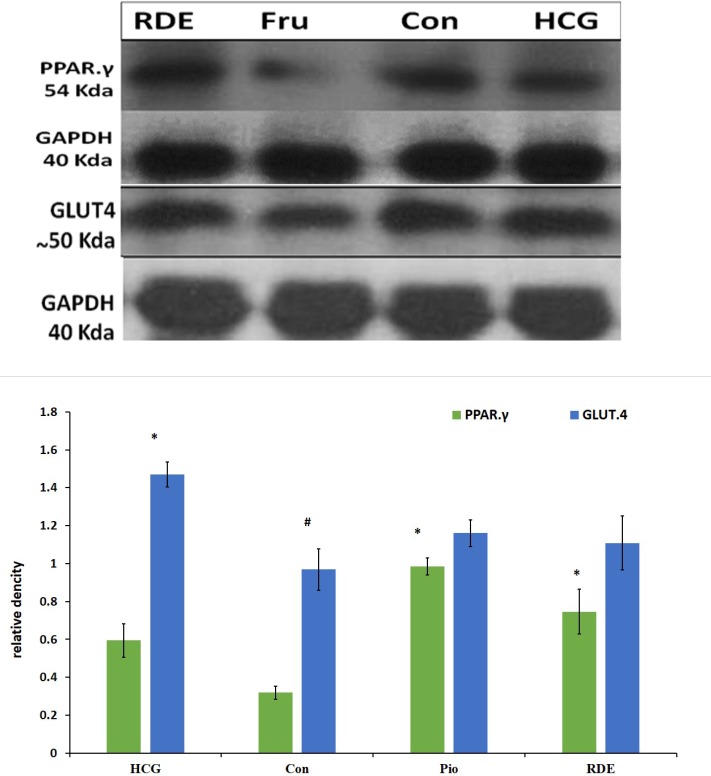
Blood and tissue collection
Animals were fasted for 12 h. overnight, blood samples were collected from heart under ether anesthesia. Blood sample was divided into two vials with or without EDTA. Vials were centrifuged at 3000 rpm for 10 min at 4 °C and plasma (for FFA analysis) or serum (for other biochemical analyses) were separated immediately.
Hind limb skeletal muscle and liver were excised. Tissue samples were immediately frozen in liquid nitrogen, and subsequently stored at −75 °C until required.
Measurement of serum parameters
Serum glucose, triglyceride, cholesterol and HDL-c concentrations were measured in an RA-1000 autoanalyser. Blood insulin and adiponectin levels were measured by ELISA method using a commercial assay kits (Mouse/Rat Adiponectin or insulin ELISA kit, USCN. China). Homeostasis Model Assessment of Insulin Resistance (HOMA-IR) was calculated using the equation [(insulin (µU/mL)×glucose (mmol/L))/22.5].
Analysis of plasma free fatty acid profiles
Plasma FFAs were extracted and analyzed by the method explained by Kangani et al. with slight modifications (16). Five hundred μL of plasma was mixed with 20 μL of pentadecanoic acid (1 mg/mL) as an internal standard. Lipids were extracted from plasma by a solvent which contained isopropanol–heptane–hydrochloric acid (1M) (40:10:1, v/v/v). FFAs were separated by Teen Layer Chromatography (TLC) on silica gel plates using a heptane–ether–acetic acid [60:40:3] solvent system. Lipids were visualized by iodine vapor on TLC plates. FFA bands were scrapped and free fatty acid methyl esters (FAME) were prepared by a reaction with BF3 in methanol (Sigma). FFA methyl esters were separated using an Agilent GC-7890A system equipped with a flame ionization detector and DB-225 capillary column (20m×0.1 mm I.D., 0.1μm film thickness, J&W GC columns, USA). The injection volume was 1μL in the split 30:1 injection mode.
Real time PCR:
Total RNA from the skeletal muscle (for GLUT.4 assay) and liver (for PPAR.γ assay) tissues was extracted with RNeasy mini kit (Qiagen) according to manufacturerʹs guidelines. The RNA concentration was determined by the ultraviolet (UV) light absorbance at 260 nm and 280 nm (ND-1000 Nanodrop). The quality of the RNA was confirmed by ethidium bromide staining of 18S and 28S ribosomal RNA bands after electrophoresis in a 2% agarose gel.
Then cDNA synthesis was performed by Quanti Tect Reverse Transcription Kit (Qiagen) according to the manufacturerʹs procedure. Relative Quantitative real time PCR was performed on a Qiagen Thermal Cycler (Rotor-Gene Q 5plex HRM System, Qiagen) using the corresponding QuantiFast SYBR Green PCR kit (Qiagen) according to manufacturerʹs protocol. The primers that were used in this study are shown in table 1.
Western blotting
Total protein was extracted from muscle or liver by homogenization of the tissue in the RIPA (Radio Immuno Precipitation Assay) buffer (Sigma, cat number: R0278). The homogenate was centrifuged at 14,000 rpm for 20 min and the supernatant which contain proteins was removed. Protein concentration was measured by Bradford method. Proteins were separated using SDS-PAGE by loading 120 μg/lane. Proteins were then transferred on a PVDF membrane. Non-specific binding sites were blocked by overnight incubating of membrane with 5% (usually a 5% solution is used) skim milk in TBST buffer at 4 °C. The membrane was then washed with TBST buffer 3 times (20 min each).
The membrane was incubated with appropriate polyclonal primary antibodies for PPAR.γ (ab27649, Rabbit polyclonal to PPAR gamma, Abcam) or GLUT.4 (ab33780, Rabbit polyclonal to GLUT4, Abcam) antibody in TBST buffer for 1 h. The membrane was then washed with TBST as described above, followed by incubation with anti-rabbit secondary antibody (Goat polyclonal HRP conjugated antibody to rabbit IgG, ab 6112, Abcam). For 1 h. at room temperature. After washing, membrane was incubated with substrate (western lightening plus ECL, Perkin-Elmer) for 1 min. Then in a dark room PVDF membrane was exposed to Hyblot film (Denvill) for 30 sec. Band densities were analyzed by image-J software.
Statistical analysis
All data are presented as mean±SEM. Statistical analysis was performed by analysis of variance (ANOVA) and Post-Hoc Tukey test; p-values of less than 0.05 were considered to be significant.
Results
Weight gain and water and food intake
Weight gain that was calculated by subtraction of primary body weight from body weight at week 6 (before drug or extract administration) showed a significant difference between Rosa damascena Extract (RDE) and control groups (P<0.0001). There was no significant difference in water or food intake between RDE group and pioglitazone or control groups (results are shown in Table 2). Results show that Rosa damascena can induce weight loss in animals in spite of intake of high fructose diet.
Serum biochemical analysis
Roasa damascena significantly decreased the levels of glucose (129±4.7 mg/dL), triglyceride (75 ± 9 mg/dL). Insulin (42 ± 2.7 pmol/L). HOMA.IR (2.2±0.18) and increased adiponectin in comparison with the control group with glucose (187±15 mg/dL), triglyceride (217 ± 18 mg/dL), insulin (137 ± 34 pmol/L). Adiponectin and HOMA.IR (9.7 ± 2.1).
Serum adiponectin was significantly increased by Rosa damascena extract (5.6 ± 0.17 µg/mL) compared to the control (3.19 ± 0.15 µg/mL) and healthy control (2.9 ± 0.16 µg/mL) groups (results are shown in Table 2).
Plasma free fatty acids
Rosa damascena showed no significant effect on total free fatty acid level in comparison with the control or healthy control groups. However, pioglitazone significantly decreased the level of total free fatty acids, oleic acid, palmitic acid and palmitoleic acid as compared with the control and RDE groups (P<0.05) (results are presented in Figure 1 and Table 3).
GLUT.4 and PPAR.γ gene expression
Our results demonstrate that rosa damascena as well as pioglitazone significantly increase the mRNA level of PPAR.γ in liver. PPAR.γ protein also was significantly increased by rosa damascena and pioglitazone. However, neither rosa damascena nor pioglitazone didn’t have any effect on mRNA or protein levels of muscle GLUT.4 (figure 2).
This study showed that there is a significant difference in PPAR.γ protein levels between RDE and control groups. Also, pioglitazone significantly increased the level of PPAR.γ protein as compared with the control group. There was no significant difference in GLUT.4 protein level between RDE and control or pioglitazone groups (Figurer 3).
Discussion
The goal of this study was to investigate the role of rosa damascena extract on insulin secretion and insulin action in animal model of insulin resistant. Our results showed that rosa damascena decreased blood glucose and insulin levels and thereupon HOMA-IR in insulin resistant rats. These results suggest that rosa damascena increases the insulin action and insulin sensitivity without increasing insulin secretion (17). Another possible mechanism is that rosa damascena has insulin like effect and through this decreases blood glucose level (18).
Gholamhoseinian et al. reported that rosa damascena has an inhibitory effect on pancreatic lipase (19). however the lowering effect of this extract on TG in hryperlipidemic rabbits was not significant (20). However, in our study, rosa damascena extract, unlike pioglitazone, significantly decreased the level of blood TG in insulin resistant rats. ATPIII guideline recommends that in treatment of metabolic syndrome, lowering triglycerides should be considered (21). Therefore, RDE seems to be more suitable than pioglitazone for treatment of metabolic syndrome.
The concentration of adiponectin was increased by administration of rosa damascena extract. Several factors, including insulin and Thiazolidinediones are associated with regulation of adiponectin secretion (22, 23). Adiponectin increases insulin sensitivity by suppression of hepatic gluconeogenesis and stimulation of fatty acid oxidation in muscles (24). These findings are sufficient to conclude that antihyperglycemic effect of RDE may be due to stimulation of adiponectin production.
At the molecular level, RDE increased the mRNA and protein of PPAR.γ in the liver, however RDE had no effect on GLUT.4 protein and mRNA in the striated muscle. PPAR-γ agonists exert their antidiabetic effect by PPAR-γ activation to increase the sensitivity of insulin receptors (25). Thiazolidinediones significantly increase insulin sensitivity and adiponectin concentration in diabetic patients (25, 26). Yadav et al. showed that PPAR.γ mRNA increased in liver of insulin resistant rats that were treated with rosiglitazone (27). PPAR.γ protein decreased in fructose fed rats and increased after treatment with pioglitazone and RDE. Similar to the role of PPAR.γ in improvement of insulin resistance, it is likely that increased PPAR-γ protein is another mechanism through which RDE applies its antihyperglycemic effect.
Agonists of PPAR.γ increase the expression and translocation of GLUT4 in adipose tissue, and increase the catabolism of glucose in the liver along with decreasing hepatic glucose output (28). We don’t sow study any difference between RDE and control group in GLUT.4 levels. Also pioglitazone didn’t have any effect on GLUT.4 protein and mRNA. In this study we evaluated total GLUT.4 in striated muscle cells. It is possible that translocation of GLUT.4 to the cell membrane after treatment with RDE and pioglitazone is increased despite the unchanged total GLUT.4 protein between groups (29).
In diabetes, the levels of free fatty acids are increased and there is a reversed correlation between plasma FFAs and insulin sensitivity in muscles (30). Although in our study there was a significant difference in serum total free fatty acids between healthy control and control groups which supports the relationship between free fatty acids and insulin resistance, total FFA or FFA fractions did not significantly change with RDE in insulin resistant rats despite increase in insulin sensitivity by RDE. Our results showed that pioglitazone (a PPAR.γ agonist) decreased the level of free fatty acids. PPAR.γ agonists enhance insulin sensitivity in adipose tissue and reduce serum FFA levels by increasing their uptake and storage in adipose tissues (31, 32). However, in our study, FFA level was not significantly changed by rosa damascena while PPAR.γ protein was increased. We only evaluated PPAR.γ gene expression in the liver to examine its effect on hepatic insulin resistance and it is possible that PPAR.γ gene expression in adipocytes does not increase.
Conclusion
The findings of this study showed that rosa damascene water extract can decrease blood glucose and triglyceride levels in insulin resistant rats. This antihyperglycemic effect was probably exerted at least by three mechanisms, including an increase in insulin action or a direct insulin-like effect, increase in adiponectin and increased PPAR.γ protein expression. Therefore, rosa damascene, because of its antihyperglycemic and antihyperlipidemic effects, can be a good candidate to control diabetes
Acknowledgements
References
-
1.
Chen L, Chen R, Wang H, Liang F. Mechanisms Linking Inflammation to Insulin Resistance. Int. J. Endocrinol. 2015;2015:508409. [PubMed ID: 26136779].
-
2.
Fuentes L, Roszer T, Ricote M. Inflammatory mediators and insulin resistance in obesity: role of nuclear receptor signaling in macrophages. Mediators Inflamm. 2010;2010:1-10.
-
3.
Jung UJ, Choi MS. Obesity and its metabolic complications: the role of adipokines and the relationship between obesity, inflammation, insulin resistance, dyslipidemia and nonalcoholic fatty liver disease. IJMCFK. 2014;15:6184-223. [PubMed ID: 24733068].
-
4.
Van Beek M, Oravecz-Wilson KI, Delekta PC, Gu S, Li X, Jin X, Apel IJ, Konkle KS, Feng Y, Teitelbaum DH, Ruland J, McAllister-Lucas LM, Lucas P. C, Bcl10 links saturated fat overnutrition with hepatocellular NF-kB activation and insulin resistance. Cell Rep. 2012;1:444-52. [PubMed ID: 22708078].
-
5.
Belkina AC, Denis GV. Obesity genes and insulin resistance. Curr. Opin. Endocrinol. Diabetes Obes. 2010;17:472-7. [PubMed ID: 20585247].
-
6.
Aleidi S, Issa A, Bustanji H, Khalil M, Bustanji Y. Adiponectin serum levels correlate with insulin resistance in type 2 diabetic patients. Saudi. Pharm. J. 2015;23:250-6. [PubMed ID: 26106273].
-
7.
Esteve E, Ricart W, Fernandez-Real JM. Adipocytokines and insulin resistance: the possible role of lipocalin-2, retinol binding protein-4, and adiponectin. Diabetes Care. 2009;32:362-7.
-
8.
Boden G. Obesity, insulin resistance and free fatty acids. Curr. Opin. Endocrinol. Diabetes Obes. 2011;18:139-43. [PubMed ID: 21297467].
-
9.
Martins AR, Nachbar RT, Gorjao R, Vinolo MA, Festuccia WT, Lambertucci RH, Cury-Boaventura MF, Silveira LR, Curi R, Hirabara SM. Mechanisms underlying skeletal muscle insulin resistance induced by fatty acids: importance of the mitochondrial function. Lipids Health Dis. 2012;11:1-11. [PubMed ID: 22221411].
-
10.
Hoeks J, Mensink M, Hesselink MK, Ekroos K, Schrauwen P. Long- and medium-chain fatty acids induce insulin resistance to a similar extent in humans despite marked differences in muscle fat accumulation. J. Clin. Endocrinol. Metab. 2012;97:208-16. [PubMed ID: 22031508].
-
11.
He J, Xu C, Kuang J, Liu Q, Jiang H, Mo L, Geng B, Xu G. Thiazolidinediones attenuate lipolysis and ameliorate dexamethasone-induced insulin resistance. Metabolism. 2015;64:826-36. [PubMed ID: 25825274].
-
12.
Ravnskjaer K, Frigerio F, Boergesen M, Nielsen T, Maechler P, Mandrup S. PPAR delta is a fatty acid sensor that enhances mitochondrial oxidation in insulin-secreting cells and protects against fatty acid-induced dysfunction. J. Lipid Res. 2010;51:1370-9. [PubMed ID: 19965574].
-
13.
Gholamhoseinian A, Fallah H, Far FS. Inhibitory effect of methanol extract of Rosa damascena Mill flowers on alpha-glucosidase activity and postprandial hyperglycemia in normal and diabetic rats. Phytomedicine. 2009;16:935-41. [PubMed ID: 19380218].
-
14.
Shih C-C, Lin C-H, Lin W-L, Jin-BinWu. Momordica charantia extract on insulin resistance and the skeletal muscle GLUT4 protein in fructose-fed rats. J. Ethnopharmacol. 2009;123:82-90. [PubMed ID: 19429344].
-
15.
Elrashidy RA, Asker ME, Mohamed HE. Pioglitazone attenuates cardiac fibrosis and hypertrophy in a rat model of diabetic nephropathy. J. Cardiovasc. Pharmacol. Ther. 2012;17:324-33. [PubMed ID: 22261714].
-
16.
Kangani CO, Kelley DE, Delany JP. New method for GC/FID and GC-C-IRMS analysis of plasma free fatty acid concentration and isotopic enrichment. J. Chromatogr. B Analyt Technol. Biomed. Life. Sci. 2008;873:95-101.
-
17.
Abdin AA, Baalash AA, Hamooda HE. Effects of rosiglitazone and aspirin on experimental model of induced type 2 diabetes in rats: focus on insulin resistance and inflammatory markers. J. Diabetes Complications. 2010;24:168-78. [PubMed ID: 19328014].
-
18.
Ohnogi H, Hayami S, Kudo Y, Deguchi S, Mizutani S, Enoki T, Tanimura Y, Aoi W, Naito Y, Kato L, Yoshikawa T. Angelica keiskei Extract Improves Insulin Resistance and Hypertriglyceridemia in Rats Fed a High-Fructose Drink Bioscience. Biosci. Biotechnol. Biochem. 2012;76:928-32. [PubMed ID: 22738961].
-
19.
Gholamhoseinian A, Shahozehi B, sharififar F. Inhibitory effect of some iranian plant extract on pancreatic lipase. Int. J. Pharm. 2010;6:18-24.
-
20.
Gholamhoseinian A, Shahuzehi B, Sharififar F. Effect of quercus infectoria and rosa damascena on lipid profile and athrosclerotic plaque formation in rabbit model of hyperlipidemia. Pak J. Biol. Sci. 2012;15:27-33. [PubMed ID: 22530439].
-
21.
Naples M, Federico LM, Xu E, Nelken J, Adeli K. Effect of rosuvastatin on insulin sensitivity in an animal model of insulin resistance: evidence for statin-induced hepatic insulin sensitization. Atherosclerosis. 2008;198:94-103. [PubMed ID: 18093597].
-
22.
Han SH, Sakuma I, Shin EK, Koh KK. Antiatherosclerotic and anti-insulin resistance effects of adiponectin: basic and clinical studies. Prog. Cardiovasc. Dis. 2009;52:126-40. [PubMed ID: 19732605].
-
23.
Ziemke F, Mantzoros CS. Adiponectin in insulin resistance: lessons from translational research. Am. J. Clin. Nutr. 2010;91:258-61.
-
24.
Lee CY, Lee CH, Tsai S, Huang CT, Wu MT, Tai SY, Lin FF, Chao NC, Chang CJ. Association between serum leptin and adiponectin levels with risk of insulin resistance and impaired glucose tolerance in non-diabetic women. J. Med. Sci. 2009;25:116-25.
-
25.
Monte SMdl, Pang M, Chaudhry R, Duan K, Lisa Longato, Carter J, Ouh J and Wands JR. Peroxisome proliferator-activated receptor agonist treatment of alcohol-induced hepatic insulin resistance. Hepatol. Res. 2011;41:386-98. [PubMed ID: 21426453].
-
26.
Park JH, Lee SH, Chung M, Park1§ Y. Sorghum extract exerts an anti-diabetic effect by improving insulin sensitivity via PPAR-γ in mice fed a high-fat diet. Nutr. Res. Pract. 2012;6:322-7. [PubMed ID: 22977686].
-
27.
Yadav H, Jain S, Yadavb M, Sinha PR, Prasad GBKS, Marotta F. Epigenomic derangement of hepatic glucose metabolism by feeding of high fructose diet and its prevention by Rosiglitazone in rats. Dig. Liver. Dis. 2009;41:500-8. [PubMed ID: 19196556].
-
28.
Elmazar MM, El-Abhar HS, Schaalan MF, Farag NA. Phytol/Phytanic Acid and insulin resistance: potential role of phytanic Acid proven by docking simulation and modulation of biochemical alterations. PLoS. One. 2013;8:4563-8.
-
29.
Kim S, Jwa H, Yanagawa Y, Park T. Extract from Dioscorea batatas ameliorates insulin resistance in mice fed a high-fat diet. J. Med. Food. 2012;15:527-34. [PubMed ID: 22424459].
-
30.
Chen Y, Li Y, Wang Y, Wen Y, Sun C. Berberine improves free-fatty-acid-induced insulin resistance in L6 myotubes through inhibiting peroxisome proliferator-activated receptor gamma and fatty acid transferase expressions. Metabolism. 2009;58:1694-702. [PubMed ID: 19767038].
-
31.
Sharma AK, Bharti S, Kumar R, Krishnamurthy B, Bhatia J, Kumari S, Arya DS. Syzygium cumini ameliorates insulin resistance and beta-cell dysfunction via modulation of PPAR, dyslipidemia, oxidative stress, and TNF-alpha in type 2 diabetic rats. J. Pharmacol. Sci. 2012;119:205-13. [PubMed ID: 22786584].
-
32.
Li RW, Theriault AG, Au K, Douglas TD, Casaschi A, Kurowska EM, Mukherjee R. Citrus polymethoxylated flavones improve lipid and glucose homeostasis and modulate adipocytokines in fructose-induced insulin resistant hamsters. Life Sci. 2006;79:365-73. [PubMed ID: 16497336].