Abstract
Keywords
Penicillamine Multiwall carbon nanotubes Modified electrode Sensor Electrocatalysis
Introduction
Sulfhydryl based compounds (thiols, R-SH) are known to play many important roles in physiological systems (1). PA is a pharmaceutically significant thiol compound, frequently used as a therapeutic agent in several diseases such as rheumatoid artluitis and Wilson’s disease, and as an antidote in heavy metal poising (2). In Wilsonʹs disease, a rare genetic disorder of copper metabolism, PA treatment relies on its binding to accumulated copper and elimination through urine (3). Various analytical methods have been used for the determination of PA in biological and pharmaceutical samples (4-7). Among the mentioned techniques, the electrochemical methods have been very successful for determination of electroactive pharmaceutical and biological compounds (8-11). Chemically modified electrodes based on carbon nanotubes have been widely used for the electrocatalytic determination of pharmaceutical and biological compounds (12-15). The low signal using modified electrodes and high over-voltage voltammetric responses of the target analyte at unmodified electrodes are often serious problems in detection with these kinds of electrodes. A number of strategies for resolving such problems have been investigated, which are typically based on application of conductive compounds at a surface of electrode such as carbon nanotubes, nanoparticles, electroactive mediators and ionic liquids (16-20).
Electrochemical sensing and especially carbon nanotubes modified sensors have been proven as an inexpensive and simple analytical method with remarkable detection sensitivity, reproducibility, and simple preparation (22). Numerous advantages of CNTs as electrode materials have been attested for analysis of diversified chemicals of pharmaceutical, food quality, clinical and environmental interest. Carbon nanotubes modified electrode-based sensors exhibit low limit of detection, good dynamic range and fast response thanks to the signal enhancement provided by high surface area, low overvoltage, and rapid electrode kinetics (23-25).In this work, the application of MDOP as a suitable homogeneous mediator in the electrocatalysis determination of PA in an aqueous buffer solution was described initially. Following, in order to demonstrate the catalytic ability of the propose sensor in the electrooxidation of PA in real samples, the method was employed for the voltammetric determination of PA in tablet, urine samples from both patients in PA treatment and healthy subjects and on medicines.
Experimental
Reagents and apparatus
All used chemicals were of analytical reagent grade purchased from Merck (Darmstadt, Germany) unless otherwise stated. Doubly distilled water was used throughout PA, methyldopa, NaOH and phosphoric acid were used from Sigma-Aldrich.
1.0 × 10-3 M PA solution was prepared daily by dissolving 0.015 g D-PA in water, and the solution was diluted to 100 mL with water in a 100-mL volumetric flask. The solution was kept in a refrigerator at 4 °C in dark. More dilute solutions were prepared by serial dilution with water.
Spectrally pure graphite powder (particle size < 50 µm) from Merck and multiwall carbon nanotubes (> 95%, MWCNTs, d × l = (100 – 70 nm) × (5 – 9 μm)) from Fluka were used as the substrate for the preparation of the carbon paste electrode. High viscosity paraffin (d = 0.88 kg L–1) from Merck was used as the pasting liquid for the preparation of the paste electrodes.
Britton-Robinson buffer or Universal buffer (boric acid, phosphoric acid, acetic acid plus sodium hydroxide, 0.04 M) solutions with different pH values were used.
All voltammetric measurements were performed using an Autolab PGSTAT 302N, potentiostat/galvanostat (Utrecht, The Netherlands) connected to a three-electrode cell, Metrohm (Herisau, Switzerland) Model 663 VA stand, linked with a computer (Pentium IV, 1,200 MHz) and with Autolab software. A platinum wire was used as the auxiliary electrode. MWCNTs/MCPE and Ag/AgCl/KClsat was used as the working and reference electrodes, respectively. The electrodes were characterized by scanning electron microscopy (SEM) (Seron Tech. AIS 2100).
Preparation of the electrode
In the first step and for eliminating any metal oxide catalysts within the nanotubes, multiwall carbon nanotubes were refluxed in 2.0 M HNO3 for 18 h, and then washed twice with distilled water and dried at room temperature. 0.9 g graphite powder was dissolved in diethyl ether and hand mixed with 0.100 g CNTs with a mortar and pestle. The solvent was evaporated by stirring. A syringe was used to add paraffin to the mixture, which was mixed well for 50 min until a uniformly wetted paste was obtained. The paste was then packed into a glass tube (diameter 0.09 cm2). Electrical contact was made by pushing a copper wire down the glass tube into the back of the mixture. When necessary, a new surface was obtained by pushing an excess of the paste out of the tube and polishing it on a weighing paper.
Preparation of real samples
For the determination of PA in pharmaceuticals (capsule), seven capsules of PA labeled with 250 mg per capsule were completely grounded and homogenized. Then, 150 mg of the powder was accurately weighed and dissolved in 5 mL of water, and then the mixture was filtered on a 0.45 mm filter. The resulted solution was diluted 5 times with the buffer (pH 5.0) and used for the determination of PA contents.
Urine samples were stored in a refrigerator immediately after collection. Ten milliliters of the sample was centrifuged for 30 min at 1500 rpm. The supernatant was filtered out using a 0.45 µm filter and then 5.0 mL of the result solution mixed with 5.0 mL of the buffer (pH 5.0). The solution was transferred into the voltammetric cell to be analyzed without any further pretreatment. The standard addition method was used for the determination of PA in real samples.
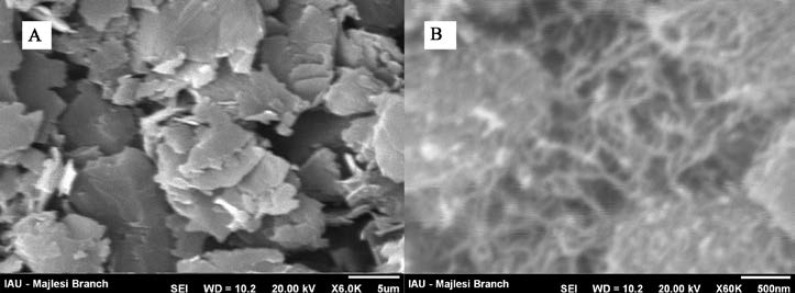
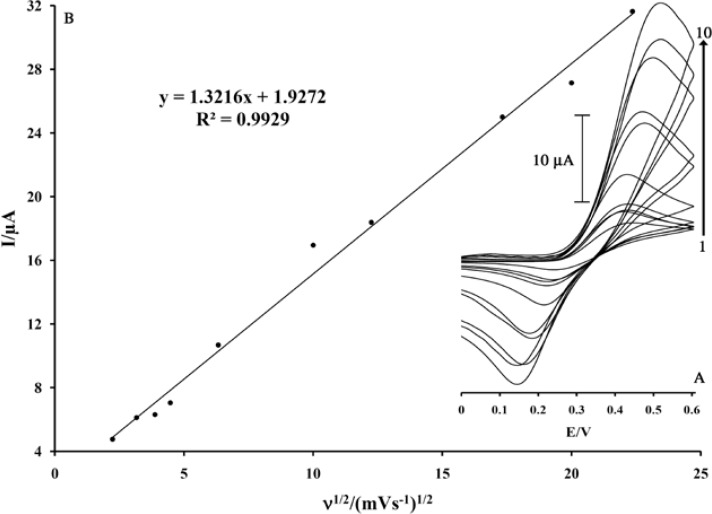
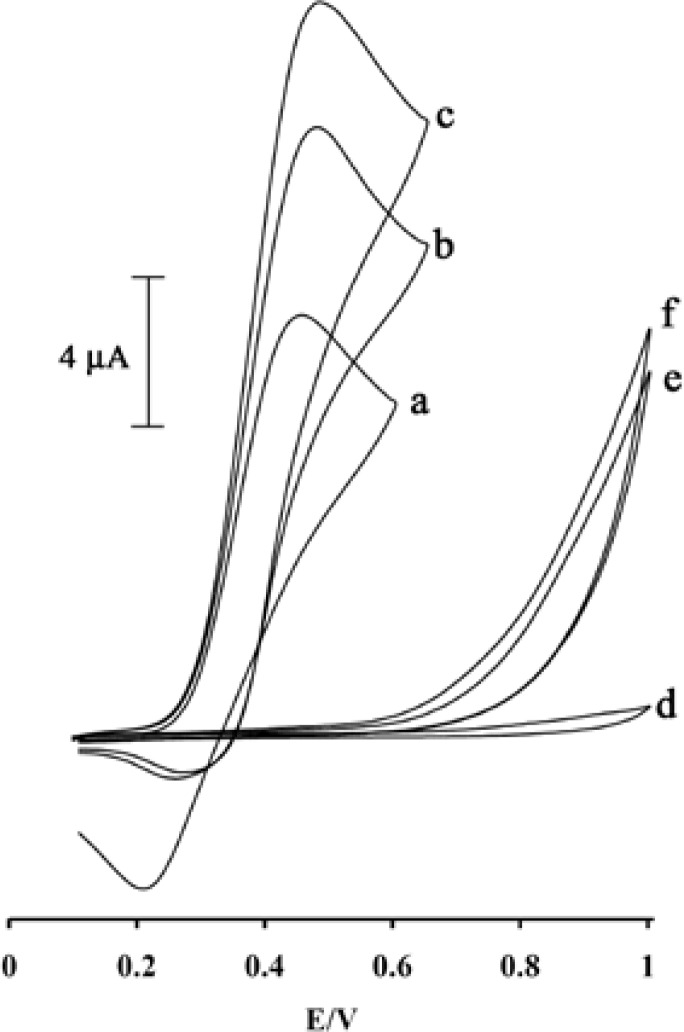
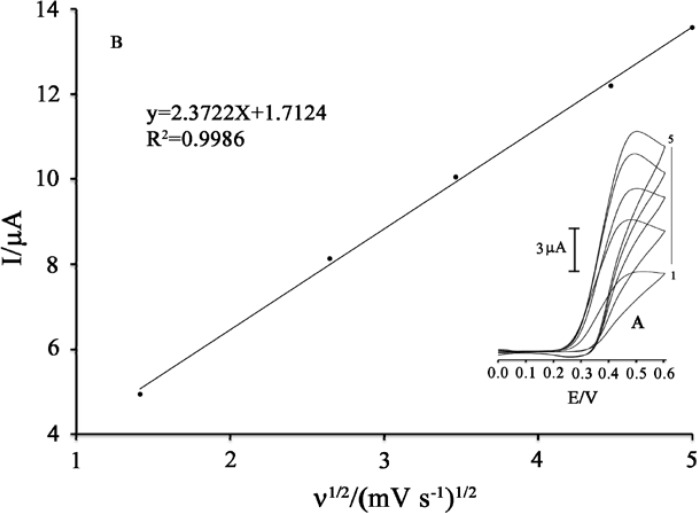
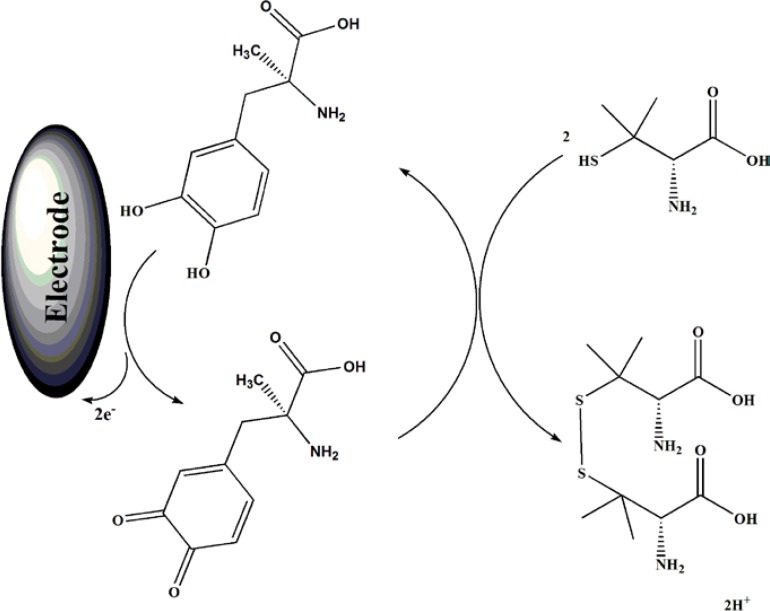
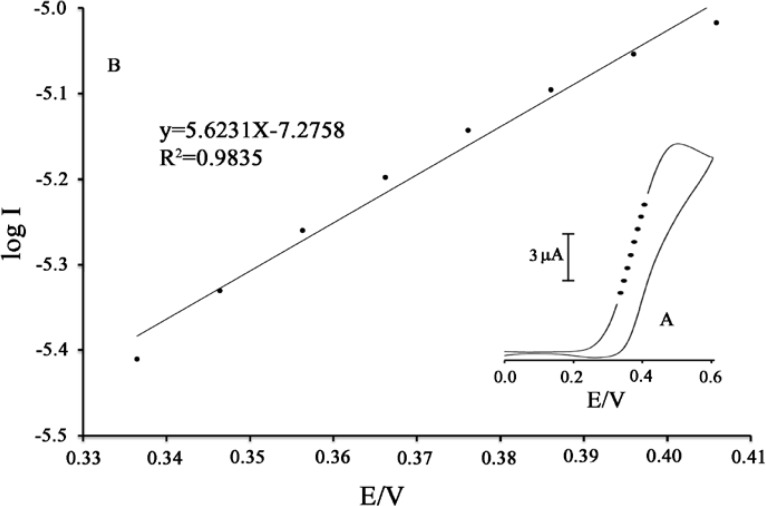
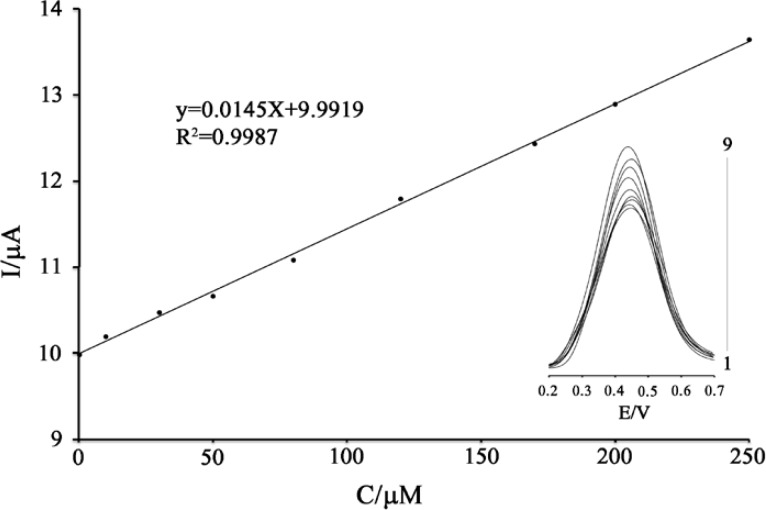
Comparison of the efficiency of some electrochemical methods in the determination of PA
Electrode | Modifier | LOD | LDR | Ref. |
---|---|---|---|---|
Carbon paste | P-Aminophenol | 0.1 | 0.4-200 | (41) |
Carbon paste | FC-derivative | 0.01 | 0.06-140 | (42) |
Carbon paste | FC-derivative | 3.9 | 7.0-230.0 | (43) |
Carbon paste | Methyldopa | 0.1 | 0.2-250 | This work |
Interference study for the determination of 5.0 µM PA under the optimized conditions
Species | Tolerante limits |
---|---|
Glucose , Fructose, Lactose, Sucrose, Valine, Methionine, Glycine, Lucine, Histidine, Glutamic acid, Alanine , Glycine, Phenylalanine | 900 |
Na+, NO3-, Cl-, SO4+2, ClO4-, K+, Li+, CO32-, F-, SCN-, Br-, Mg2+ | 800 |
Thiourea, urea, Tryptophan, Caffeine, Methanol, Ethanol | 600 |
Starch | Saturation |
Ascorbic acid* | 500 |
Determination of PA in tablet and urine samples (n=3).
Sample | PA added | Expected value | PA founded | Published Method | tex | ttab | Fex | Ftab |
---|---|---|---|---|---|---|---|---|
Tablet | −− | 5.0 | 5.2 ± 0.4 | 4.8 ± 0.5 | 1.9 | 3.8 | 7.5 | 19.0 |
15.0 | 20.0 | 19.6 ± 0.7 | 20.8 ± 1.0 | --- | --- | --- | --- | |
15.0 | 45.0 | 45.8 ± 0.9 | 45.8 ± 1.0 | --- | --- | --- | --- | |
Urine | −− | −− | <Limit of detection | <Limit of detection | --- | --- | --- | --- |
10.0 | 10.0 | 10.6 ± 0.8 | 10.9 ± 0.9 | 2.0 | 3.8 | 8.5 | 19.0 | |
10.0 | 20.0 | 20.7 ± 0.8 | 21.0 ± 1.1 | --- | --- | --- | --- | |
Urine a | −− | −− | 4.9 ± 0.9 | 5.2 ± 0.3 | 1.5 | 3.8 | 6.5 | 19.0 |
Recommended procedure
The MWCNTs/MCPE was polished with a white and clean paper. To prepare a blank solution for analysis, 20.0 mL of buffer solution (optimum condition; pH 5.0), was transferred into an electrochemical cell. The initial and final potentials were adjusted to –0.2 and +0.70 V, respectively. Different amounts of PA solution were added to the cell, using a micropipette, and the SWV was recorded again to get the analytical signal (Ips) in the presence of mediator. Calibration curves were constructed by plotting the catalytic peak current vs. the PA concentration.
Results and Discussion
SEM Characterization
The morphology of the unmodified and nanostructures modified electrodes were characterized by SEM. Figure 1 shows SEM images for MWCNTs/MCPE and carbon paste electrode (CPE). As can be seen at a surface of CPE (Figure 1A), the layer of irregularly flakes of graphite powder was present and isolated with each other. After multiwall carbon nanotubes added to carbon paste, it can be seen that MWCNTs were distributed on the surface of electrode with special three-dimensional structure (Figure 1B), indicating that the MWCNTs were successfully modified on the MWCNTPE.
Electrochemical investigation
As MDOP is soluble in an aqueous solution, it can be easily used as a homogeneous mediator for electrocatalytic process. The electrochemical behavior of MDOP at a surface of MWCNTs/MCPE was investigated. The cyclic voltammograms of the modified electrode in 0.04 M universal buffer (pH 5.0) are shown in Figure 2 insert. As can be seen, the cyclic voltammogram exhibits an anodic peak at the forward scan of the potential related to the oxidation of the MDOP (Red) to MDOP (Ox) form. In the reverse scan of the potential, a cathodic peak appears related to the reduction of MDOP (Ox) form, to MDOP (Red). A pair of quasi reversible peaks are observed at Epa = 0.45 V and Epc = 0.19 V vs. Ag/AgCl. The half-wave potential (E1/2) was 0.32 V vs. Ag/AgCl and ΔEp (Epa −Epc) was 0.26 V.
The electrode process was quasi reversible, with ΔEp, greater than the expected value (59/n mV) for a reversible system. The plot of the anodic peak current was linearly dependent on ν1/2 for all scan rates (Figure 2). This behavior indicates that the nature of the redox process is diffusion controlled.as we know, the electrochemical behavior of PA and methyldopa depend on pH value of the aqueous solution. So, it is very necessary for optimization of pH value to obtain the best condition in electroanalysis of PA. to this end, the electrooxidation behavior of PA in 0.04 M universal buffer solution with various pH (3.00 < pH < 6.00) at the surface of modified electrode were studied. The Result showed, the maximum electrocatalytic current was obtained at pH 5.0. Therefore, all of the electrochemical experiments were done at this pH.
Figure 3 depicts the cyclic voltammogram responses for the electrochemical oxidation of 100 µM PA at modified electrode in the presence of MDOP (curve c), carbon paste electrode (CPE) in the presence of MDOP (curve b) and MWCNTs/MCPE and CPE without MDOP (curve f and e), respectively.
Result shows, while the anodic peak potential for PA oxidation at the MWCNTs/MCPE, and CPE in the presence of mediator is ≈900, the corresponding potential at MWCNTPE and CPE without mediator are ≈497 mV. These results indicate that the peak potential for PA oxidation at the MWCNTs/MCPE, and CPE in the presence of mediator shifts by 403 mV toward negative values compared to MWCNTs/MCPE and CPE (without MDOP), respectively. However, MWCNTs/MCPE in the presence of mediator shows a much higher anodic peak current for the oxidation of analyte compared to CPE (with MDOP), indicating that the combination of CNTs and the MDOP has significantly improved the performance of the electrode toward PA oxidation. On the other hand, MWCNTs/MCPE (with mediator in the solution) in the absence of PA exhibited a well-behaved redox reaction (Figure 3, curve a) in 0.04 M universal buffer solution (pH 5.0). However, there was a drastic increase in the anodic peak current in the presence of 100 µM PA (curve b or c), which can be related to the strong electrocatalytic effect of the mediator towards this compound (see scheme 1) (26-28).
The effect of scan rate on the electrocatalytic oxidation of PA at the MWCNTs/MCPE and in the presence of 500 µM MDOP as a mediator was investigated by cyclic voltammetry (Figure 4A). Result shows the oxidation peak potential shifted towards a more positive potential with increasing scan rate, confirming the kinetic limitation of the electrochemical reaction
In addition, a plot of peak height (Ip) against square root of scan rate (ν1/2) was constructed (Figure 4B) which was found to be linear, suggesting that, at sufficient over potential, the process is diffusion rather than surface controlled (33-35). In order to obtain information about the rate-determining step, Tafel plots (plots of log I vs. potential) were drawn (Figure 5) which were derived from points of the Tafel region of the cyclic voltammogram in Figure 5 (insert).
The results of the polarization studies for electro-oxidation of PA at the MWCNTs/MCPE in the presence of mediator showed that for all potential sweep rates, the average Tafel slope was 5.6231 V-1 decade. The slope of the Tafel plot was equal to n(1 − α)F/2.3RT. We obtained nα equal to 0.67. Assuming n = 1, then α = 0.67. In addition, the value of αnα was calculated for the electro-oxidation of PA at pH 5.0 for modified electrode in the presence of mediator using the following equation:
αnα = 0:048/ (EP-EP/2) (1)
where EP/2 is the potential corresponding to IP/2. The value for αnα was found to be 0.66.
Chronoamperometry results were obtained for the various concentrations of PA solution using MWCNTs/MCPE and in the presence of 500 µM MDOP (Not shown). The plot of current (I) versus t-1/2 for PA solution at various concentrations using MWCNTs/MCPE and in the presence 0f 500 µM MDOP gives straight lines with different slopesFrom the slopes, a diffusion coefficient of 5.9×10−4 cm2 s−1 for PA using the Cottrell equation was calculated.
Chronoamperometry can also be employed to evaluate the catalytic rate constant, kh for the reaction between PA and MWCNTs/MCPE and in the presence 0f 500 µM MDOP according to the method of Galus (36):
IC/IL=γ1/2[π1/2erf (γ1/2) + exp(−γ)/γ1/2] (2)
Where IC is the catalytic current of PA at MWCNTs/MCPE and in the presence of 500 µM MDOP, IL is the limiting current in the absence of PA and γ=khCbt (Cb is the bulk concentration of PA) is the argument of the error function. In the cases where γ exceeds two the error function is almost equal to 1 and therefore, the above equation can be reduced to:
IC/IL= γ1/2 π1/2=π1/2 (khCbt)1/2 (3)
Where t is the time elapsed in seconds. The above equation can be used to calculate the rate constant of the catalytic process kh. Based on the slope of IC/ILvs.t1/2 plot, kh can be obtained for a given PA concentration. From the values of the slopes, an average value of kh was found to be kh= 5.83 × 102 mol−1 L s−1. The value of kh explains the sharp feature of the catalytic peak observed for catalytic oxidation of PA at the surface of MWCNTs/MCPE and in the presence of 500 µM MDOP.
Double potential step chronocoloumetry, as well as other electrochemical methods, was also employed for the investigation of electrode processes at MWCNTs/MCPE and in the presence of 500 µM MDOP (Not shown).
Forward and backward potential step chronocoloumetry on the modified electrode in a blank buffer solution showed very symmetrical chronocolougrams. These had about an equal charge consumed for both oxidation and reduction of the quinone/hydroquinone redox system in MDOP. However, in the presence of PA, the charge value associated with forward chronocoloumetry was significantly greater than what was observed for backward chronocoloumetry. This behavior is typical of what expected for electrocatalysis of chemically modified electrodes (37-40).
Calibration plot and detection limit
Since square wave voltammetry (SWV) has a much higher current sensitivity and better resolution than cyclic voltammetry, the SWV was used for the determination of PA (Figure 6 inset). SWVs clearly show that the plot of the peak current vs. PA concentration is linear for 0.2 – 250 µM of PA, with a regression equation of Ip(µA) = (0.0145 ± 0.0025) CPA + (9.9919 ± 0.9235) (r2 = 0.9989, n = 9), where C is µM concentration of PA, and Ip is the peak current (Figure 6). The detection limit (LOD) was 0.1 µM PA according to the definition of LOD = 3sb/m (where Sb is the standard deviation of the blank signal (n = 12) and m is the slope of the calibration. Comparisons of the results from the different methods are shown in Table 1.
Stability and reproducibility
The repeatability and stability of MWCNTs/MCPE and in the presence of 500 µM MDOP was investigated by SWV measurements of 10.0 µM PA. The relative standard deviation (RSD%) for seven successive assays are 1.4%. When using five different electrodes, the RSD for four measurements is 2.0%. When the electrode stored in our laboratory at room temperature, the modified electrode retains 97% of its initial MWCNTs/MCPE and in the presence of 500 µM MDOP has good stability and reproducibility, and could be used for PA.
Interference study
The influence of various substances as potential interfering compounds with the determination of PA was studied under the optimum conditions with 5.0 µM PA at pH 5.0. The potentially interfering substances were chosen from the group of substances commonly found with PA in pharmaceuticals and/or in biological fluids. Tolerance limit was defined as the maximum concentration of the interfering substance that caused an error less than ±5% for the determination of PA. The results are presented in Table 2.
Real sample analysis
Electrochemical methods are powerful techniques for determination of pharmaceutical and biological compounds in real samples (44-52). In order to demonstrate the ability of the modified electrode to the determination of PA in real samples, determination of PA in pharmaceutical and in urine samples were examinedwhose results are given in Table 3. These results demonstrated the ability ofproposing sensor for voltammetric determination of PA with high selectivity and good reproducibility.
Conclusion
This research describes the ability of the MWCNTs/MCPE in the presence of 500 µM MDOP as a suitable mediator for electro-catalytic determination of PA. The proposed sensor is highly sensitive to PA levels as low as 0.1 μM. Under the best conditions in square wave voltammetric determination, the oxidation peak current was proportional to the PA concentration in the range of 0.2–250 μM.
The kinetic parameter of the electrocatalytic process and the diffusion coefficients of PA in an aqueous solution were determined. Finally, the proposed sensor was also examined as a selective, simple, and precise electrochemical sensor for the determination of PA in real samples such as drug and urine.
Acknowledgements
References
-
1.
Shahrokhian S, Bozorgzadeh S. Electrochemical oxidation of dopamine in the presence of sulfhydryl compounds: application to the square-wave voltammetric detection of penicillamine and cysteine. Electrochim. Acta. 2006;51:4271-6.
-
2.
Zhang Z, Baeyens WRG, Zhang X, Zhao Y, Van Der Weken G. Chemiluminescence detection coupled to liquid chromatography for the determination of penicillamine in human urine. Anal. Chim. Acta. 1997;347:325-32.
-
3.
Peisach J, Blumberg WE. A mechanism for the action of penicillamine in the treatment of Wilson’s disease. Mol. Pharmcol. 1969;5:200-9.
-
4.
Liang SC, Wang H, Zhang ZM, Zhang HS. Determination of thiol by high-performance liquid chromatography and fluorescence detection with 5-methyl-(2-(m-iodoacetylaminophenyl) benzoxazole. Anal. Bioanal. Chem. 2005;381:1095-100. [PubMed ID: 15744519].
-
5.
Ensafi AA, Ghiaci M, Arshadi M, Karimi-Maleh H. Synthesis and characterization of ferrocenecarboxaldehyde immobilized on modified SiO2–Al2O3 in nanoscale, application for determination of penicillamine. J. Nanopart. Res. 2013;15:1610.
-
6.
Walash MI, El-Brashy AM, Metwally ME, Abdelal AA. Spectrophotometric determination of penicillamine and carbocisteine based on formation of metal complexes. Farmaco. 2004;59:493-503. [PubMed ID: 15178313].
-
7.
Ensafi AA, Karimi-Maleh H, Mallakpour S. N-(3,4-dihydroxyphenethyl)-3,5-dinitrobenzamide-modified multiwall carbon nanotubes paste electrode as a novel sensor for simultaneous determination of penicillamine, uric acid, and tryptophan. Electroanalysis. 2011;23:1478-87.
-
8.
Ensafi AA, Karimi-Maleh H, Mallakpour S, Rezaei B. Highly sensitive voltammetric sensor based on catechol-derivative-multiwall carbon nanotubes for the catalytic determination of captopril in patient human urine samples. Colloids Surf. B Biointerfaces. 2011;87:480-8. [PubMed ID: 21726986].
-
9.
Ensafi AA, Karimi-Maleh H, Mallakpour S, Hatami M. Simultaneous determination of N-acetylcysteine and acetaminophen by voltammetric method using N-(3,4-dihydroxyphenethyl)-3,5-dinitrobenzamide modified multiwall carbon nanotubes paste electrode. Sens. Actuators B Chem. 2011;155:464-72.
-
10.
Tavana T, Khalilzadeh MA, Karimi-Maleh H, Ensafi AA, Beitollahi H, Zareyee D. Sensitive voltammetric determination of epinephrine in the presence of acetaminophen at a novel ionic liquid modified carbon nanotubes paste electrode. J. Mol. Liq. 2012;168:69-74.
-
11.
Beitollah H, Goodarzian M, Khalilzadeh MA, Karimi-Maleh H, Hassanzadeh M, Tajbakhsh M. Electrochemical behaviors and determination of carbidopa on carbon nanotubes ionic liquid paste electrode. J. Mol. Liq. 2012;173:137-43.
-
12.
Ansari M, Kazemi S, Khalilzadeh MA, Karimi-Maleh H, Pasha Zanousi MB. Sensitive and stable voltammetric measurements of norepinephrine at ionic liquid–carbon nanotubes paste lectrodes. Int. J. Electrochem. Sci. 2013;8:1938-48.
-
13.
Naushad M, Gupta VK, Wabaidur SM, Alothman ZA. Simultaneous determination of benserazide and levodopa in pharmaceutical tablet, human serum and urine sample by differential pulse voltammetry using modified glassy carbon electrode. Int. J. Electrochem. Sci. 2013;8:297-311.
-
14.
Sanghavi BJ, Srivastava AK. Adsorptive stripping differential pulse voltammetric determination of venlafaxine and desvenlafaxine employing Nafion–carbon nanotube composite glassy carbon electrode. Electrochim. Acta. 2011;56:4188-96.
-
15.
Yola LM, Atar N, Üstündağ Z, Solak AO. A novel voltammetric sensor based on p-aminothiophenol functionalized graphene oxide/gold nanoparticles for determining quercetin in the presence of ascorbic acid. J. Electroanal. Chem. 2013;698:9-16.
-
16.
Yola ML, Gupta VK, Eren T, Sen AE, Atar N. A novel electro analytical nanosensor based on graphene oxide/silver nanoparticles for simultaneous determination of quercetin and morin. Electrochim. Acta. 2014;120:204-11.
-
17.
Sanghavi BJ, Kalambate PK, Karna SP, Srivastava AK. Voltammetric determination of sumatriptan based on a graphene/gold nanoparticles/Nafion composite modified glassy carbon electrode. Talanta. 2014;120:1-9. [PubMed ID: 24468334].
-
18.
Karimi-Maleh H, Biparva P, Hatami M. A novel modified carbon paste electrode based on NiO/CNTs nanocomposite and (9,10-dihydro-9,10-ethanoanthracene-11,12-dicarboximido)-4-ethylbenzene-1,2-diol as a mediator for simultaneous determination of cysteamine, nicotin amide adenine dinucleotide and folic acid. Biosens. Bioelectron. 2013;48:270-5. [PubMed ID: 23707873].
-
19.
Karimi-Maleh H, Tahernejad-Javazmi F, Ensafi AA, Moradi R, Mallakpour S, Beitollahi H. A high sensitive biosensor based on FePt/CNTs nanocomposite /N-(4-hydroxyphenyl)-3,5-dinitrobenzamide modified carbon paste electrode for simultaneous determination of glutathione and piroxicam. Biosens. Bioelectron. 2014;60:1-7. [PubMed ID: 24755294].
-
20.
Raoof JB, Ojani R, Karimi-Maleh H. Carbon paste electrode incorporating 1-[4-(ferrocenyl ethynyl) phenyl]-1-ethanone for electrocatalytic and voltammetric determination of tryptophan. Electroanalysis. 2008;20:1259-62.
-
21.
Elyasi M, Khalilzadeh MA, Karimi-Maleh H. High sensitive voltammetric sensor based on Pt/CNTs nanocomposite modified ionic liquid carbon paste electrode for determination of Sudan I in food samples. Food Chem. 2013;141:4311-7. [PubMed ID: 23993620].
-
22.
Vashist SK, Zheng D, Al-Rubeaan K, Luong JHT, Sheu FS. Advances in carbon nanotube based electrochemical sensors for bioanalytical applications. Biotechnol. Adv. 2011;29:169-88. [PubMed ID: 21034805].
-
23.
Ensafi AA, Karimi-Maleh H. Modified multiwall carbon nanotubes paste electrode as a sensor for simultaneous determination of 6-thioguanine and folic acid using ferrocenedicarboxylic acid as a mediator. J. Electroanal. Chem. 2010;640:75-83.
-
24.
Beitollahi H, Karimi-Maleh H, Khabazzadeh H. Nanomolar and selective determination of epinephrine in the presence of norepinephrine using carbon paste electrode modified with carbon nanotubes and novel 2-(4-Oxo-3-phenyl-3,4-dihydroquinazolinyl)-N′-phenyl-hydrazinecarbothioamide. Anal. Chem. 2008;80:9848-51. [PubMed ID: 19072278].
-
25.
Merkoci A. Carbon nanotubes in analytical sciences. A review. Microchim. Acta. 2006;152:155-74.
-
26.
Ojani R, Raoof JB, Zamani S. Electrochemical behavior of chloranil chemically modified carbon paste electrode. application to the electrocatalytic determination of ascorbic acid. Electroanalysis. 2005;17:1740-5.
-
27.
Moradi R, Sebt SA, Karimi-Maleh H, Sadeghi R, Karimi F, Bahari A, Arabi H. Synthesis and application of FePt/CNTs nanocomposite as a sensor and novel amide ligand as a mediator for simultaneous determination of glutathione, nicotinamide adenine dinucleotide and tryptophan. Phys. Chem. Chem. Phys. 2013;15:5888-97. [PubMed ID: 23486920].
-
28.
Roodbari Shahmiri M, Bahari A, Karimi-Maleh H, Hosseinzadeh R, Mirnia N. Ethynylferrocene–NiO/MWCNT nanocomposite modified carbon paste electrode as a novel voltammetric sensor for simultaneous determination of glutathione and acetaminophen. Sens. Actuators B Chem. 2013;177:70-7.
-
29.
Keyvanfard M, Salmani-mobarakeh R, Karimi-Maleh H, Alizad K. Application of 3, 4-dihydroxycinnamic acid as a suitable mediator and multiwall carbon nanotubes as a sensor for the electrocatalytic determination of L-cysteine, Chinese J. Catal. 2014;35:1166-72.
-
30.
Keyvanfard M, Tahmasbi M, Karimi-Maleh H, Alizad K. A voltammetric sensor with a multiwall carbon nanotube paste electrode and naphthol green as a mediator for the determination of N-actylcysteine in the presence of tryptophan. Chinese J. Catal. 2014;35:501-8.
-
31.
Beitollahi H, Mohadesi A, Ghorbani F, Karimi-Maleh H, Baghayeri M, Hosseinzadeh R. Electrocatalytic measurement of methionine concentration with a carbon nanotube paste electrode modified with benzoylferrocene. Chinese J. Catal. 2013;34:1333-8.
-
32.
Baghizadeh A, Karimi-Maleh H, Khoshnama Z, Hassankhani A. A voltammetric sensor for simultaneous determination of vitamin C and vitamin B6 in food samples using ZrO2 nanoparticle/ionic liquids carbon paste electrode. Food Anal. Methods. 2015;8:549-57.
-
33.
Najafi M, Khalilzadeh MA, Karimi-Maleh H. A new strategy for determination of bisphenol A in the presence of Sudan I using a ZnO/CNTs/ionic liquid paste electrode in food samples. Food Chem. 2014;158:125-31. [PubMed ID: 24731323].
-
34.
Karimi-Maleh H, Moazampour M, Ahmar H, Beitollahi H, Ensafi AA. A sensitive nanocomposite-based electrochemical sensor for voltammetric simultaneous determination of isoproterenol, acetaminophen and tryptophan. Measurement. 2014;51:91-9.
-
35.
Ensafi AA, Karimi-Maleh H, Ghiaci M, Arshadi M. Characterization of Mn-nanoparticles decorated organo-functionalized SiO2–Al2O3 mixed-oxide as a novel electrochemical sensor: application for the voltammetric determination of captopril. J. Mater. Chem. 2011;21:15022-30.
-
36.
Galus Z. Fundumentals of Electrochemical Analysis. New York: Ellis Horwood; 1976.
-
37.
Karimi-Maleh H, Hatami M, Moradi R, Khalilzadeh MA, Amiri S, Sadeghifar H. Synergic effect of Pt-Co nanoparticles and a dopamine derivative in a nanostructured electrochemical sensor for simultaneous determination of N-acetylcysteine, paracetamol and folic acid. Microchim. Acta. 2016;183:2957-64.
-
38.
Bagheri H, Karimi-Maleh H, Karimi F, Mallakpour S, Keyvanfard M. Square wave voltammetric determination of captopril in liquid phase using N-(4-hydroxyphenyl)-3,5-dinitrobenzamide modified ZnO/CNT carbon paste electrode as a novel electrochemical sensor. J. Mol. Liq. 2014;198:193-9.
-
39.
Karimi-Maleh H, Mehdipour-Ataei S, Hatami M, Khalilzadeh MA. Voltammetric determination of captopril using a novel ferrocene-based polyamide as a mediator and multi-wall carbon nanotubes as a sensor. J. Anal. Chem. 2014;69:162-8.
-
40.
Keyvanfard M, Sami S, Karimi-Maleh H, Alizad K. Electrocatalytic Determination of cysteamine using multiwall carbon nanotube paste electrode in the presence of 3,4-dihydroxycinnamic acid as a homogeneous mediator. J. Braz. Chem. Soc. 2013;24:32-9.
-
41.
Ensafi AA, Khoddami E, Rezaei B, Karimi-Maleh H. p-Aminophenol–multiwall carbon nanotubes–TiO2 electrode as a sensor for simultaneous determination of penicillamine and uric acid. Colloids Surf. B. 2010;81:42-9.
-
42.
Ensafi AA, Ghiaci M, Arshadi M, Karimi-Maleh H. Synthesis and characterization of ferrocenecarboxaldehyde immobilized on modified SiO2–Al2O3 in nanoscale, application for determination of penicillamine. J. Nanopart. Res. 2013;15:1610.
-
43.
Raoof JB, Ojani R, Chekin F, Hossienzadeh R. Carbon paste electrode incorporating 1-[4-(ferrocenyl ethynyl) phenyl]-1-ethanone for voltammetric determination of D-penicillamine. Int. J. Electrochem. Sci. 2007;2:848-60.
-
44.
Yola ML, Eren T, Atar N. A sensitive molecular imprinted electrochemical sensor based on gold nanoparticles decorated graphene oxide: application to selective determination of tyrosine in milk. Sens. Actuators B Chem. 2015;210:149-57.
-
45.
Yola ML, Eren T, Atar N. Molecularly imprinted electrochemical biosensor based on Fe@Au nanoparticles involved in 2-aminoethanethiol functionalized multi-walled carbon nanotubes for sensitive determination of cefexime in human plasma. Biosens. Bioelectron. 2014;60:277-85. [PubMed ID: 24832202].
-
46.
Yola ML, Eren T, İlkimen H, Atar N, Yenikaya C. A sensitive voltammetric sensor for determination of Cd(II) in human plasma. J. Mol. Liq. 2014;197:58-64.
-
47.
Yola ML, Eren T, Atar N. Determination of amikacin in human plasma by molecular imprinted SPR nanosensor, Sens. Actuators B Chem. 2014;198:70-6.
-
48.
Yola ML, Eren T, Atar N. A novel efficient photocatalyst based on TiO2 nanoparticles involved boron enrichment waste for photocatalytic degradation of atrazine. Chem. Engin. J. 2014;250:288-94.
-
49.
Yola ML, Eren T, Atar N. A novel and sensitive electrochemical DNA biosensor based on Fe@Au nanoparticles decorated graphene oxide. Electrochim. Acta,. 2014;125:38-47.
-
50.
Karimi-Maleh H, Fallah Shojaei A, Tabatabaeian K, Karimi F, Shakeri S, Moradi R. Simultaneous determination of 6-mercaptopruine,6-thioguanine and dasatinib as three important anticancer drugs using nanostructure voltammetric sensor employing Pt/MWCNTs and 1-butyl-3- methyl imidazolium hexafluoro phosphate. Biosens. Bioelectron. 2016;86:879-84. [PubMed ID: 27494812].
-
51.
Bavandpour R, Karimi-Maleh H, Asif M, Gupta VK, Atar N, Abbasghorbani M. Liquid phase determination of adrenaline uses a voltammetric sensor employing CuFe2O4 nanoparticles and room temperature ionic liquids. J. Mol. Liq. 2016;213:369-73.
-
52.
Karimi-Maleh H, Rostami S, Gupta VK, Fouladgar M. Evaluation of ZnO nanoparticle ionic liquid composite as a voltammetric sensing of isoprenaline in the presence of aspirin for liquid phase determination. J. Mol. Liq. 2015;201:102-7.