Abstract
Keywords
Introduction
Food provides essential supplies of life sustaining nutrients, vitamins, and minerals but it may also contain a variety of naturally-occurring toxins in varying quantities. Some toxins present a health risk to consumers but this usually occurs only when certain food items have not been properly prepared. Research has identified a beneficial role of some of these naturally occurring substances, such as preventing illnesses. However, there is a more important risk of contamination by synthetic compounds such as pesticides. Public policy should be directed toward reducing any unnecessary overburden of pesticide residue in the human diet (1). Cereal production, among other food types, has a historical association to human development. Cultivation of cereal crops has played a very important role in the so-called ‘‘Neolithic revolution’’, in which humans first achieved full control over their food supply. This is accepted as the point that human development shifted to formation of agricultural societies and, with that a new concept of civilization was borne that has partly survived until today (2). Cereals constitute one of the most intensely produced and consumed food products in the world. They are an important global product and an important part of the human diet, as a source of energy and high contents of essential fatty acids, nutritious proteins, and dietary fiber; cereals also supply important minerals, vitamins, and other micronutrients that are essential for the maintenance of optimal health. In particular, cereal grains are the basis of bread, which is one mankind’s earliest food products and that currently constitutes a major food technology. There is currently huge demand for cereals, and cereal derivatives to feed the ever-growing global population and significant effort is needed to improve production yields. Pest control makes an important contribution to maximizing yields. There are more than 1100 pesticides currently registered on the status list of all active pesticide substances in the European Union (EU) market (3). However there is a negative effect from application of pesticides in agricultural practice, many pesticides are harmful to the environment and are known as or suspected to be toxic to humans. There is increasing public concern about the possible health risks of pesticide residue in the diet that has deeply modified strategies for crop protection, with the main emphasis on food quality and safety. Widespread health concerns have led to strict regulation of maximum residue limits (MRLs) of pesticide residue in food products. To date, more than 17,000 European Community MRLs have been set for various commodities for 133 active pesticide substances (4). Pesticides have been used for many years. In earlier times they were used to protect against fungi and insect pests and/or to provide quality preservation (5). This great increase in the use of pesticides has occurred with the development of new organic chemicals following the two major world wars; WWI and WW2. In addition to the chemicals used to control fungi and insects, new developments have been introduced such as; nematocides, herbicides, rodenticides, avicides, defoliants, and wood preservatives (6). Discussions on risks associated with human exposure to pesticides pay particular attention to direct poisoning and fatality, potential induction of cancer, and effects on reproduction and immune and nervous systems, diabetes, neurodegenerative disorders such as Parkinson’s disease, chronic diseases, and genetic damage, epigenetic modifications, endocrine disruption, mitochondrial dysfunction, oxidative stress, endoplasmic reticulum stress, and diminished intelligence (1, 5, 7, 8). In agriculture for wheat production, pesticides such pirimicarb, chlorpyrifos, carbaryl, malathion, propiconazole, tebuconazole and triadimenol are used to prevent infestations of insects, fungi, and weeds. The European Union (EU) has set maximum residue limits (MRLs) for the above-mentioned pesticides in wheat crops at 0.5, 0.05, 0.5, 8, 0.05, 0.2, and 0.2 mg/kg respectively (9).
Today, the most frequently employed analytical approach to determine levels of pesticide in foods is based on mass spectrometry, such as Gas chromatography–mass spectrometry (GC–MS). In the case of Green aspects and environmental risks, Anastassiades et al. suggested a simple, safe, cheap, high sample throughput method namely QuEChERS in pesticides residue analysis (10). This study was performed according to the safe QUECHERS method.Over the past decades, approaches to trace level determination of pesticides have moved away from the use of GC with selective detectors including electron capture detection (ECD) (11, 12) and nitrogen phosphorus detection (NPD) (13). To mass spectrometer (MS). detectors which are more sensitive and selective (14). The use of mass spectrometry, with its information-rich content and explicit confirmation, is recommended for monitoring pesticide residues in the entire world (15-17).
This is the first attempt in Iran using GC-MS-SIM technique and spike calibration curve for simultaneous determination of determine 58 pesticides with difference in physicochemical properties in wheat flour marketed in Tehran, Iran. The other outcome of this study is calculating the estimated daily intake (EDT) of the pesticides via wheat flour and its comparison with the acceptable daily intake (ADI). Methods
Samples Collection
Tehran city was divided into five districts and samples from each district was collected independently and equally of different Bakery in January of 2014 (Barbari (n=10), Sangak (n=10). Baguette (n=10) and Lavash (n=10)).
Sample preparation
For sample preparation, an aliquot of 5 μL of internal standard solution (Triphenylmethane: 1000 mg/L) was added to 10 g of wheat flour samples in a warring blender and after being left for 1 h at ambient temperature in dark, 12 mL acetonitrile was added. The mixture was blended at high speed for 1 min. One gram of NaCl and tow grams MgSO4 were added to the mixture and blending was continued for an additional 90 sec. After centrifugation for 5 min at 4500 rpm in – 5 °C, 1 g of Mg SO4 and 0.3 g of PSA added to the supernatant. The mixture was blended at high speed for 2 min. The mixture was centrifuged again. The supernatant was evaporated to dryness by Nitrogen gas. The residue was reconstituted in 1 mL toluene and the mixture was blended at high speed for 3 min, then 2 μL of the solution was injected into gas chromatograph (Figure 1) (18).
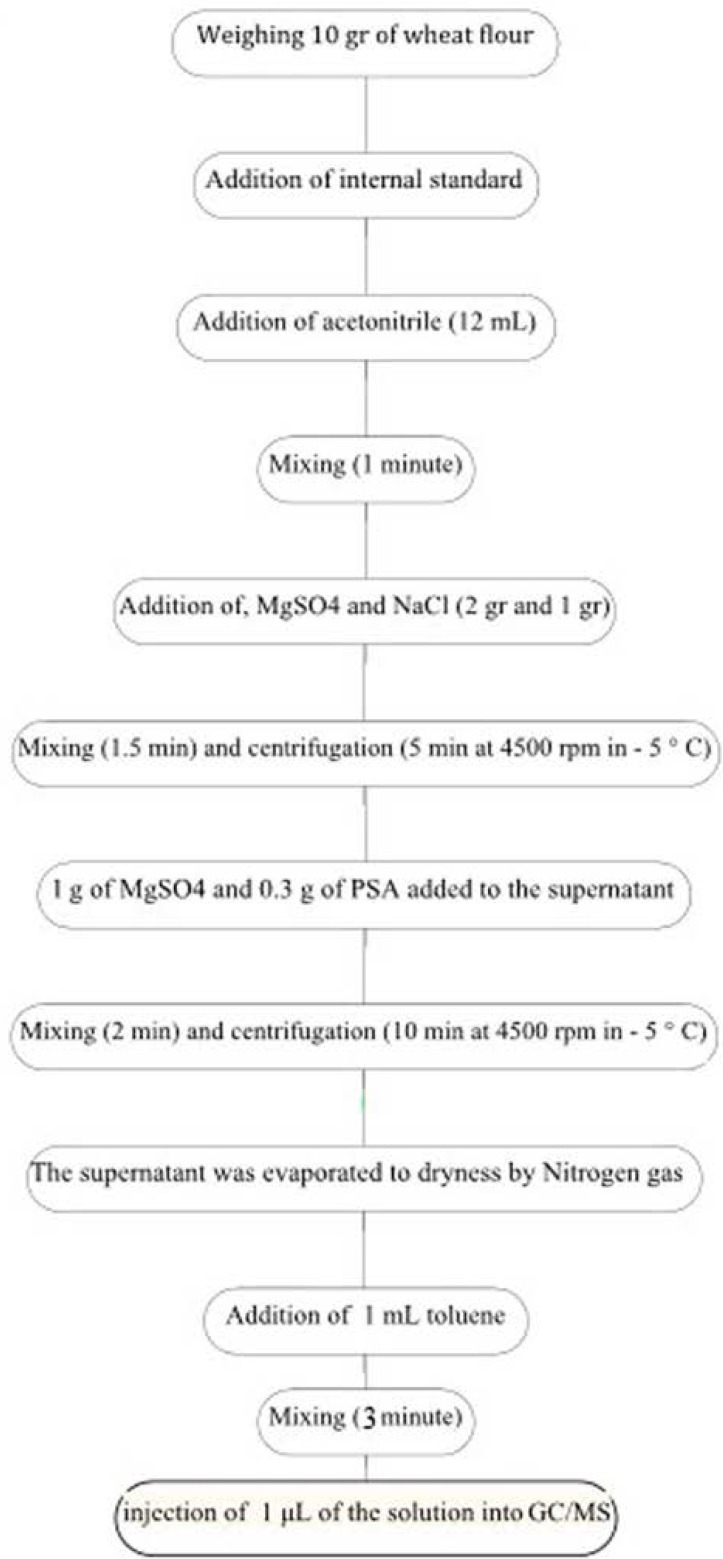
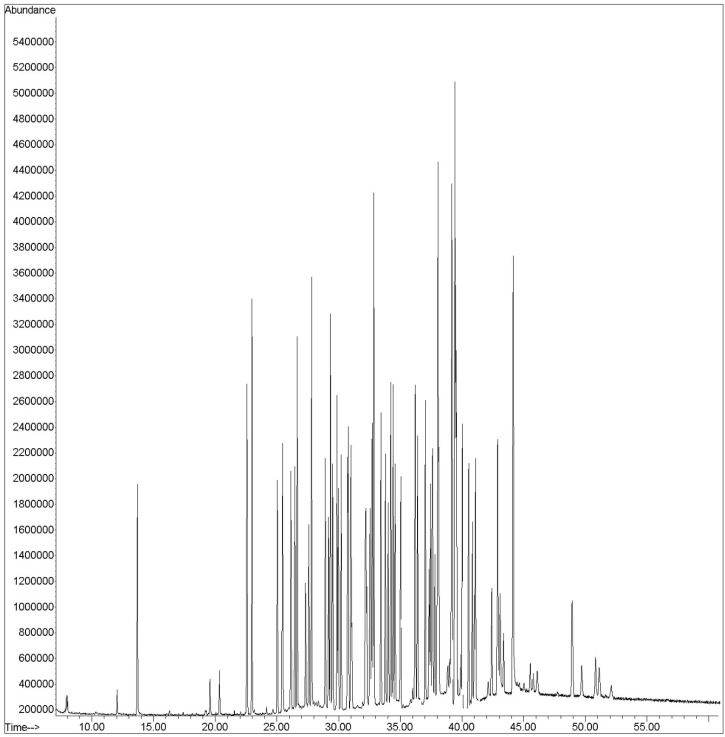
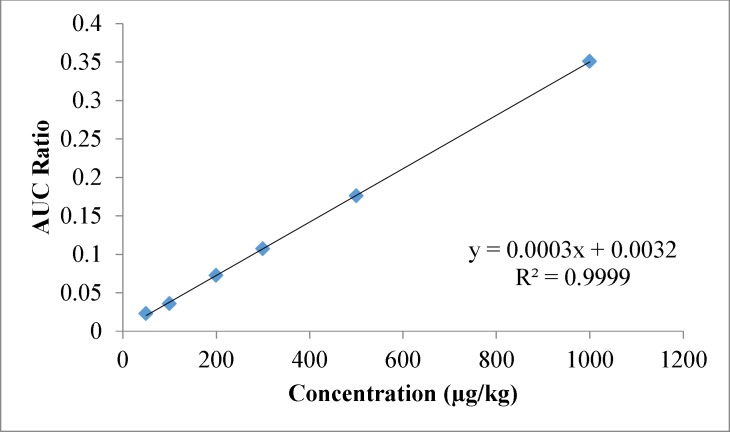
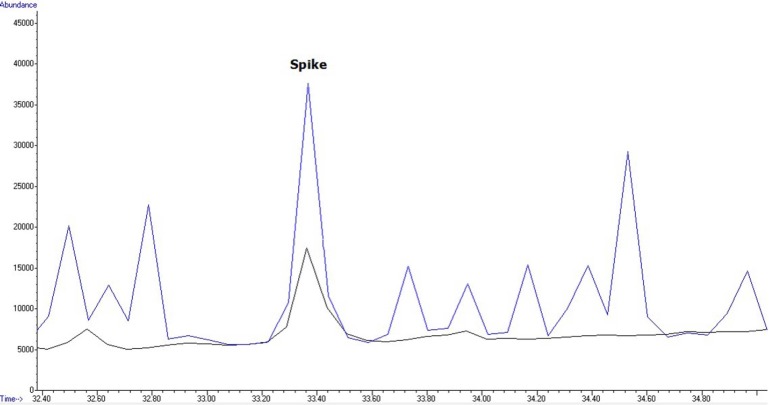
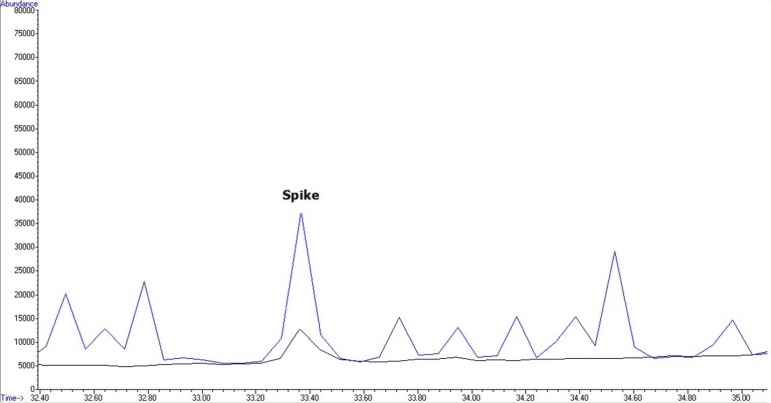
Average recoveries (%) and relative standard deviations (%) obtained by GC-MS analysis of wheat flour at 6 spiking levels (n = 3) in wheat flour samples
Compound | Total average recovery(%), (n=18) | Range of RSDr(%) (n=6) |
---|---|---|
Propoxure 1 | 116.60 | 8.22-15.07 |
Dichlorvos | 99.64 | 7.13-16.69 |
Captan | 115.77 | 0.88-19.56 |
Carbaryl 1 | 110.06 | 1.00-18.80 |
Propoxure 2 | 108.84 | 1.20-19.60 |
Diphenyl amine | 110.92 | 3.80-14.90 |
Alpha HCH | 102.08 | 5.90-16.60 |
Dimetoate | 99.17 | 1.72-14.93 |
Beta HCH | 96.10 | 3.70-14.70 |
Gamma HCH | 102.43 | 7.40-18.40 |
Diazinon | 114.56 | 2.00-19.63 |
Etrimfos | 111.56 | 5.11-18.91 |
Chlortalonil | 101.48 | 4.92-19.30 |
Pirimicarb | 101.62 | 3.48-16.21 |
Chlorpyrifos- methyl | 112.17 | 1.58-12.03 |
Carbaryl 2 | 89.68 | 7.34-19.75 |
Metalaxyl | 104.03 | 4.20-18.23 |
Heptachlor | 95.34 | 5.20-19.55 |
Primiphos methyl | 107.82 | 3.85-13.60 |
Fenitrothion | 106.22 | 2.80-19.00 |
Malathion | 109.13 | 3.30-17.80 |
Fention | 109.85 | 3.40-19.75 |
Chlorpyrifos | 104.28 | 2.80-18.85 |
Aldrine | 106.33 | 3.96-19.13 |
Dicofol | 113.57 | 4.85-16.42 |
Bioalthrin | 100.89 | 6.16-19.30 |
Heptachlor-exo-epoxide | 109.00 | 5.69-15.82 |
Triadimenol 1 and 2 | 100.01 | 4.50-13.90 |
Heptachlor-endo-epoxide | 88.48 | 8.15-19.51 |
Procymidone | 89.42 | 2.63-17.51 |
2,4 DDE | 101.35 | 9.09-17.60 |
Fenamiphos | 105.40 | 5.85-17.25 |
Alpha-endosulfan | 97.25 | 5.67-17.40 |
Hexaconazole | 106.81 | 2.34-12.26 |
4,4 DDE | 107.61 | 6.32-12.56 |
Dieldrin | 100.43 | 4.89-19.58 |
Ethion | 86.61 | 5.32-18.15 |
Trifloxystrobin | 102.19 | 3.51-16.85 |
Propiconazole 1 | 91.91 | 8.08-18.88 |
Propiconazole 2 | 106.21 | 7.71-13.95 |
Edifenphos | 88.80 | 4.52-14.40 |
Endosulfan-suiphat | 110.65 | 1.64-18.61 |
Propargite | 115.80 | 10.49-19.93 |
Piperonyl butoxide | 102.08 | 2.63-17.10 |
Tebuconazole | 102.48 | 2.98-18.96 |
Fenpropathrin | 94.73 | 10.97-18.38 |
Etoxazole | 115.73 | 7.47-11.79 |
Fenazaquin | 108.26 | 11.19-18.62 |
Tetradifon | 111.46 | 4.19-18.49 |
Phosalon | 111.43 | 2.84-16.91 |
Azinphos-Methyl | 82.46 | 5.68-17.91 |
Lambda cyhalothrin | 101.46 | 5.08-14.62 |
Azinphos- Ethyl | 108.30 | 5.89-18.14 |
Permethrin 1 | 104.54 | 2.80-11.10 |
Permethrin 2 | 104.97 | 1.27-19.96 |
Fenvalerate 1 | 102.04 | 2.43-17.14 |
Fenvalerate 2 | 94.43 | 2.45-18.80 |
Range | 81.46-116.06 | 0.88-19.96 |
Levels of pesticide residues in flour samples collected from the Tehran, Iran, 2014
Sample | Pesticides | Concentration(µg/Kg) | MRL(µg/Kg) |
---|---|---|---|
Baguette | 2,4-DDE | 19.88±15.24 | 50 |
Barbari | 2,4-DDE | 13.7±15.24 | 50 |
Sangak1 | Malathion | 50.96±11.38 | 500 |
Sangak2 | Malathion | 62.088±11.38 | 500 |
ADI and EDI of pesticides residue for an adult(µg/kg bw/day).
Chemicals and reagents
All pesticide standards were purchased from Dr. Ehrenstorfer Co. (Augsburg, Germany). All organic solvents, intended for extraction, were at least of Chromatography grade and purchased from Merck (Darmstadt, Germany). Bulk quantities of anhydrous MgSO4 and NaCl were obtained from Merck (Darmstadt, Germany).
GC–SQ/MS
An Agilent Technologies 6890N Network GC System chromatographs (Wilmington, USA) with a SQ mass detector and equipped with an Agilent 7683B autosampler (Agilent technologies, USA) was used. A HP-5 capillary column (30 m × 0.25 mm I.D., 1 μm film thicknesses) was used for separation
Quality assurance/Quality control
For quality assurance of the results method validation was carried out according to ICH criteria and for quality assurance of the tests in each run two quality control samples were tested with along with the real samples.
Calibration standards
Individual stock standard solutions (10 µg/mL) were prepared in ethyl acetate and stored in dark at – 20 °C. They were kept for 1 h at ambient temperature prior to their use.
Spiked calibration standards were prepared by addition of 10 μL, 25 μL, 50 μL, 100 μL, 200 μL, 300 μL, 500 μL and 1000 μL of mixed standard stock solution respectively to 10 g of blank wheat flour samples in each case. A stock solution of triphenylmethane (TPM) in ethyl acetate at concentration of 500 µg/L was used as internal standard. An aliquot of 5 μL of TPM solution in ethyl acetate was added to the spiked flour sample as internal standard. The samples so obtained were treated as described in sample preparation section.
Recovery studies
For recovery determination, spiked wheat flour blank samples at concentration levels of 15, 25, 75, 150, 250, 450 and 750 µg/mL were prepared in triplicates and then treated according to the procedure described in sample preparation. Calibration curve was drawn for each pesticide by Excel program
GC-SQ–MS analysis
The GC-SQ-MS was employed with helium as the carrier gas at a constant flow rate of 1 mL/min. The oven temperature started at 75 °C and remained at this temperature for 3 min increasing to 120 °C in a ramp rate of 25 °C/min and then increased to 300 °C at 5 °C/min ramp, holding at 300 °C for 11 min. The injection port was adjusted at 250 °C and the splitless mode was used.
After acquisition of the total ion chromatogram for the mixed stock standard solutions in scan mode, peaks were identified by their retention time and mass spectra. The identification was confirmed by comparing the relative abundances for three ions (one quantifier and two qualifiers) of the experimental standards to the known relative abundances of the Pest Library reference spectra. The most abundant ion that showed no evidence of chromatographic interference and had the highest signal-to-noise ratio was taken for quantification purposes.
Quantitation
The concentrations of pesticides were determined by interpolation of the relative peak areas for each pesticide to internal standard peak area in the sample on the spiked calibration curve. In order to compensate for losses during sample processing and instrumental analysis, internal standard (TPM) was used.
Uncertainty management
Uncertainty using the coverage factor of 2 and at the confidence level of 95% was expressed (19, 20).
Results and Discussion
Gas chromatographic determination
Analysis was performed in the SIM mode based on the use of one target and two qualifier ions. Pesticides were identified according to their retention times, target, and qualifier ions. The quantitation was based on the peak area ratio of the targets to that of internal standard. Calibration curves were constructed for each compound using six different concentration levels. For identification of pesticides, the retention time and three ions (one for quantitation and two for identification) were used. A GC–SQ–MS chromatogram of 58 pesticides and internal standard (TPM) analyzed in spiked flour is shown in Figure 2
Method validation
Linearity of the standards in spiked calibration curves
The 58 pesticides showed linearity in the SIM mode. Linear spiked calibration curves for all the pesticides were obtained with correlation coefficients between 0.977 to 0.999. The spiked calibration curve for Propoxure in wheat flour sample is shown in Figure 3 as a representative
Limitation of quantification
Limits of quantification (LOQs) of the proposed method (21) were calculated by considering a value 10 times more than that of background noise in spiked samples at lowest levels. LOQ is the lowest concentration of analyte in a spike sample, which can be quantitatively determined with suitable precision and accuracy.
The limits of detection and limit of quantifications for all the pesticides were < 10 ng/g and < 25 ng/g, respectively. The LOQs for 37 pesticides were 15 ng/g and for the other 21 pesticides were calculated as 25 ng/g in this method.
Recovery
Table 1 presents the recovery and repeatability for the six concentration levels. The recovery of pesticides at these six levels was in the range of 81.61-118.41%. In terms of repeatability, the vast majority of pesticides gave RSD < 20% with n = 3 at each spiking level. The recoveries and repeat abilities are in accordance with the criteria set by SANTE Guideline (22)
Real samples
For application of the method to real samples, 40 wheat flour samples were purchased from local markets across Tehran in January 2014. Samples were analyzed according to the method described above. For evaluation and analysis, one QC sample at 200 µg/mL was used in each working round. Four of the 40 samples showed contamination with Malathion (2 samples: 50.96 ± 11.38 and 62.088 ± 11.38 ppb) and 2, 4-DDE (2 samples: 19.88±15.24 and 13.7 ± 15.24 ppb), that had levels below MRLs of these pesticides in Iran. Levels of pesticide residue in flour samples collected from Tehran, Iran, 2014 are shown in Table 2Figures 4 and 5 show an overlaid chromatogram of a spiked flour sample at 200 µg/mL levels and contaminated flour samples in SIM mode.
According to percentages of residues observed in the wheat flour samples, it was determined that 5% of the samples contained 2, 4-DDE and 5% malathion, while the remaining 90% did not contain any pesticide residue. In the present study, residue of malathion and 2, 4-DDE was observed in 4 samples, but concentrations were not higher than the EU MRLs.
Estimated daily intake (EDI) of the determined pesticides residue in wheat was calculated, and compared with the ADI. The estimated daily intake (EDI) of pesticides was determined based on capita consumption of wheat (482.2 g per day) of an adult (body weight taken as 60 kg) as follows: 0.08 for Malathion and 0.1 µg/kg bw/day for 2, 4-DDE (Table 3). The recommended ADI for malathion for an adult is reported to be 300 µg/kg bw/day (23).
The main reason of low pesticide residues in the wheat flour samples in this study, are likely to impact processes such as grinding and debranning of the wheat.
Although the declared value in our study is lower than the recommended ADI, these results do not preclude the need for continuing comprehensive studies for pesticides residue.
The health risk from consumption of organophosphorus pesticide residue has also been investigated in cereals in other countries. For example, Maver et al., (2007) analyzed organophosphorus pesticide residue of many commodities, including cereals in Slovenia (24). In Another report, Bai et al., (2006) investigated organophosphorus pesticide residue in market foods including cereals in China. They also, found that organophosphorus residue levels were below the MRLs in cereals (25). Bakore et al., (2004) reported that all wheat samples were contaminated with various organochlorine pesticide residue in tests conducted in India. The report also stated that aldrin and heptachlor levels were higher than those of the practical limit prescribed by the WHO (26).
Malekirad et al., (2013) reported that farmers who work with organophosphorus pesticide are prone to neuropsychological disorders and diabetes (27).
Taghavian et al., (2016) showed that the chronic exposure to organophosphate pesticides can affect the quality of life, depression, anxiety and stress and may endanger their mental health (28).
In the study reported by Toteja et al. (2006), residues of DDT (p, p-DDT, o, p-DDT, p, p-DDE) were detected in 78.2% of 632 wheat flour samples but only 1.7% of the total samples analyzed were found to be contaminated with DDT above the maximum residue limit recommended by the Codex (29). In our study, 2,4-DDE was observed in 5% of 40 wheat flour samples, but none of the levels determined in samples exceeded the EU MRLs. Naik et al. (2006) states that endosulfan residue in wheat was reported in all samples in India but levels of pesticide residue were well below the MRLs. These results were unlike those of the present study, in which none of the samples were contaminated with endosulfan. A study-conducted by Naik stated that wheat and rice samples taken from a KGS organic farm showed traces of organochlorine pesticide (30). Saeed et al., (2001) reported the presence of chlorpyrifos-methyl in most samples containing wheat or wheat flour. Levels in wheat flour (both white and brown) ranged from 37 to 720 ng/g but imported wheat flour was not found to contain any detectable residue. In their study, non-wheat cereals and products did not contain any detectable residue of chlorinated pesticide (31). In our study, none of the wheat flour samples were found to be contaminated with chlorpyrifos-methyl. Guler et al. (2010) reported that all the tested wheat samples were contaminated by organochlorine pesticide residue. In some samples levels of organochlorine pesticide residue were higher than limits set by the EU (32). Reports on recent national monitoring programs of pesticide residue in food tested in Europe, USA and Canada, of which data in the US and Canada included imported samples, were all found to have residue in fruit and vegetable products as well as in wheat samples (33-36). Malting is a process applied to cereal grains; it is a combination of two processes; germination and kiln-drying. The condition of pesticides was determined in the malting process from barley to malt. Kaushik et al. reported that concentrations of residue after malting ranged from 13–51% for fenitrothion and nuarimol (37). Navarro et al. stated that stages of the malting process such as steeping, germination, and kilning contributed to loss of pesticide residue. Steeping was the most important stage in terms of removal of residue (52%) followed by germination (25%) and then kilning (drying and curing) 23% (38). Holland et al. (1994), reported that most residue loss occurred during malting on account of high dilution with water (39).
The bread making process involves two major steps; firstly, yeast-mediated fermentation and then subjection of the substrate to a high temperature for final baking. Both of these factors contribute to degradation of pesticide residue (40). Kaushik et al., (2009), observed that in general, the range of pesticide degradation during bread making was highest (75–89%) in samples fortified with 1 ppm. However, variation in residue dissipation of individual pesticides during bread making was observed. At the level of 4 ppm fortification, degradation was in the following order; endosulfan (70%), deltamethrin (63%), malathion (60%), propiaconazole (52%), chlorpyriphos (51%) and hexaconazole (46%), (37).
A weakness of this study was that only 40 samples were included in the study, this constraint was determined by budget. However, the study did include samples from all the major wheat flour sources consumed in Tehran, Iran.
Conclusions
In conclusion, the wheat flour consumed in Tehran, was analyzed for 58 pesticides residues by using GC-MS-SIM technique and spike calibration curve. In total 4 samples contained detectable residues (frequency 10%) of pesticides. The residue levels encountered were relatively low and none of these residues exceeded the EU and ISIRI maximum residue limit (MRLs).
Although, concentration of pesticides in all of the samples under study meets EU limits, still it is recommended to improve residue control at production, tighter regulation of pesticide spraying and also tighter regulation in the sale of pesticides as well as for education of farm personnel and the implementation of integrated pest management methods as ways to reduce contamination with pesticides residue. In this regard, a food control system (i.e. GAP and HACCP). training courses for farmers can be helpful. Moreover, pesticides residue put emphasis on implementing regular monitoring and stricter food safety management system (FSMS).
The proposed method not only allowed the simultaneous determination and confirmation of 58 pesticides with good recoveries and low detection limits, but also showed to be useful in routine analysis due to its fast and easy procedure. Also, in this study EDI of pesticides in wheat flour was compared with the ADI.
Competing interests
The authors declare that they have no competing interests.
Acknowledgements
References
-
1.
Culliney TW, Pimentel D, Pimentel MH. Pesticides and natural toxicants in foods. Agric. Ecosyst. Environ. 1992;41:297-320.
-
2.
González-Curbelo MÁ, Herrera-Herrera AV, Ravelo-Pérez LM, Hernández-Borges J. Sample-preparation methods for pesticide-residue analysis in cereals and derivatives. Trends Anal. Chem.; TrAC. 2012;38:32-51.
-
3.
-
4.
-
5.
Hercegová A, Dömötörová M, Matisová E. Sample preparation methods in the analysis of pesticide residues in baby food with subsequent chromatographic determination. J. Chromatogr. A. 2007;1153:54-73. [PubMed ID: 17258222].
-
6.
Mrak EM. Pesticides: the good and the bad. Regul. Toxicol. Pharmacol. 1984;4:28-36. [PubMed ID: 6425996].
-
7.
Mostafalou S, Abdollahi M. Pesticides and Human Chronic Diseases; Evidences, Mechanisms, and Perspectives. Toxicol. Appl. Pharmacol. 2013;268:157-77. [PubMed ID: 23402800].
-
8.
Gupta P. Pesticide exposure—Indian scene. Toxicol. 2004;198:83-90.
-
9.
EU. Pesticide EU-MRLs Database Regulation. Corrigendum to Commission Regulation (EC); 2011. 396 p.
-
10.
Anastassiades M, Lehotay SJ, Štajnbaher D, Schenck FJ. Fast and easy multiresidue method employing acetonitrile extraction/partitioning and “dispersive solid-phase extraction” for the determination of pesticide residues in produce. J. AOAC Int. 2003;86:412-31. [PubMed ID: 12723926].
-
11.
Dórea HS, Lima Sobrinho L. Analysis of pesticide residues in rice using matrix solid-phase dispersion (MSPD). J. Braz. Chem. Soc. 2004;15:690-4.
-
12.
Chen S, Shi L, Shan Z, Hu Q. Determination of organochlorine pesticide residues in rice and human and fish fat by simplified two-dimensional gas chromatography. Food Chem. 2007;104:1315-19.
-
13.
Oishi M, Onishi K, Kano I, Nakazawa H, Tanabe S. Capillary gas chromatographic determination of thiabendazole in citrus and apple juices. J. AOAC Int. 1993;77:1293-6.
-
14.
Lehotay SJ, Eller KI. Development of a method of analysis for 46 pesticides in fruits and vegetables by supercritical fluid extraction and gas chromatography/ion trap mass spectrometry. J. AOAC Int. 1994;78:821-30.
-
15.
Pengyan L, Qingxue L, Yusong M, Jinwei L, Xuan J. Analysis of pesticide multiresidues in rice by gas chromatography-mass spectrometry coupled with solid phase extraction. Chin. J. Chromatogr. 2006;24:228-34.
-
16.
Liu L, Yuki H, Qin Y, Zhou H, Lin J. Rapid analysis of multiresidual pesticides in agricultural products by gas chromatography-mass spectrometry. Chin. J. Anal. Chem. 2006;34:783-6.
-
17.
Nguyen TD, Lee BS, Lee BR, Lee DM, Lee GH. A multiresidue method for the determination of 109 pesticides in rice using the Quick Easy Cheap Effective Rugged and Safe (QuEChERS) sample preparation method and gas chromatography/mass spectrometry with temperature control and vacuum concentration. Rapid Commun. Mass Spectrom. 2007;21:3115-22. [PubMed ID: 17705253].
-
18.
Shoeibi S, Amirahmadi M, Yazdanpanah H, Pirali-Hamedani M, Pakzad SR, Kobarfard F. Effect of cooking process on the residues of three carbamate pesticides in rice. Iran. J. Pharm. Res. 2011;10:119. [PubMed ID: 24363690].
-
19.
-
20.
Fotowicz P. A method of approximation of the coverage factor in calibration. Measurement. 2004;35:251-6.
-
21.
ICH Guidance for Industry- Q2B Validation of Analytical Procedures: Methodology. EMA; 1995.
-
22.
SANTE. Guidance document on analytical quality control and method validation procedures for pesticides residues analysis in food and feed. 2015. 11945 p.
-
23.
ISIRI. Pesticides -Maximum residue limit of pesticides - Cereals. ISIRI; 2010. 13120 p.
-
24.
Maver L, Stajnbaher D, Gros L, Kahne-Jurisevic B, Repse B, Sinigoj-Gacnik K. Residues of organophosphorus pesticides in different food commodities in Slovenia, 1997? 1998. Int. J. Environ. Pollut. 2007;31:142-54.
-
25.
Bai Y, Zhou L, Wang J. Organophosphorus pesticide residues in market foods in Shaanxi area, China. Food Chem. 2006;98:240-2.
-
26.
Bakore N, John P, Bhatnagar P. Organochlorine pesticide residues in wheat and drinking water samples from Jaipur, Rajasthan, India. Environ. Monit. Assess. 2004;98:381-9. [PubMed ID: 15473547].
-
27.
Malekirad AA, Faghih M, Mirabdollahi M, Kiani M, Fathi A, Abdollahi M. Neurocognitive, mental health, and glucose disorders in farmers exposed to organophosphorus pesticides. Arch Ind Hyg Toxicol. 2013;64:1-8.
-
28.
Taghavian F, Vaezi G, Abdollahi M, Malekirad AA. A Comparative Study of the Quality of Life, Depression, Anxiety and Stress in Farmers Exposed to Organophosphate Pesticides with those in a Control Group. JCHR. 2016;6:143-52.
-
29.
Toteja G, Diwakar S, Mukherjee A, Singh P, Saxena B, Kalra R, Kapoor S, Kaur H, Raizada R, Singh V. Residues of DDT and HCH in wheat samples collected from different states of India and their dietary exposure: A multicentre study. Food Addit. Contam. 2006;23:281-8. [PubMed ID: 16622952].
-
30.
Naik S, Prasad R. Pesticide residue in organic and conventional food-risk analysis. Chem. Health Saf. 2006;13:12-9.
-
31.
Saeed T, Sawaya WN, Ahmad N, Rajagopal S, Al-Omair A, Al-Awadhi F. Chlorinated pesticide residues in the total diet of Kuwait. Food Control. 2001;12:91-8.
-
32.
Guler G, Cakmak Y, Dagli Z, Aktumsek A, Ozparlak H. Organochlorine pesticide residues in wheat from Konya region, Turkey. Food Chem. Toxicol. 2010;48:1218-21. [PubMed ID: 20156519].
-
33.
Canadian Food Inspection Agency. Young Children’s Food Chemical Residues Project Report on Agricultural Pesticides Residues 2003-2004. CFIA. Canada; 2005.
-
34.
CEC. Monitoring of Pesticide Residues in Products of Plant Origin in the European Union. Norway, Iceland and Liechtenstein: CEC; 2005.
-
35.
FDA. Pesticide Program Residue Monitoring. Food and Drug Administration. 2005.
-
36.
PRC. Annual Review of the Pesticides Residues Committee. PRC, UK. PRC Stands; 2006.
-
37.
Kaushik G, Satya S, Naik S. Food processing a tool to pesticide residue dissipation–A review. Food Res. Int. 2009;42:26-40.
-
38.
Navarro S, Pérez G, Navarro G, Vela N. Decline of pesticide residues from barley to malt. Food Addit. Contam. 2007;24:851-9. [PubMed ID: 17613072].
-
39.
Holland P, Hamilton D, Ohlin B, Skidmore M. Effects of storage and processing on pesticide residues in plant products. Pure Appl. Chem. 1994;66:335-56.
-
40.
Sharma J, Satya S, Kumar V, Tewary DK. Dissipation of pesticides during bread-making. Chem. Health Saf. 2005;12:17-22.