Abstract
Keywords
Lipid nanoparticles Palm Griseofulvin Particle size Drug loading Stability Zeta potential
Introduction
Lipid nanoparticles (LN) was first introduced in 1991 to formulate poorly soluble drugs for better drug protection and a controlled drug release profile (1, 2). The LN was developed from the oil-in-water (o/w) emulsion wherein the oil phase in the o/w emulsion is replaced with solid lipid (3). Investigations have reported the significance of LN for topical use and the results are promising (4, 5). LN forms a coherent film that reduces the transepidermal water loss (TEWL) and thus hydrates the skin to facilitate the penetration of compound from LN (6, 7). It has been reported that the occlusive efficiency of LN is 15-folds higher than that of microparticles (2).
Griseofulvin (C17H17ClO6) (Figure 1) was first isolated in 1939 by Oxford from Penicillium griseofulvum (8) and it was introduced as an oral antifungal agent (9). It is a fungistatic agent against dermatophytosis (tinea infection) caused by Epidermophyton, Trichophyton and Microsporum by disrupting the mitotic spindle of the dermatophytes and inhibiting its division (10). Till date, griseofulvin is only available in oral preparation form (11, 12) and it is known as a poorly soluble drug with poor absorption via the gastrointestinal tract (13). The absorption can be improved with fatty meal but it requires a long period of oral treatment (10). Griseofulvin has also been reported for its interactions with drugs like coumarin and oral contraceptives (10). In addition, it causes systemic side effects, namely headaches, gastrointestinal disturbance, blood dyscrasis and hepatotoxicity (14). This has led to the interest in developing a topical formulation for griseofulvin. A single topical application of griseofulvin has been reported to have higher skin concentration of griseofulvin (remained detectable from skin after 4 days of application) when compared with a prolonged oral administration of griseofulvin (15). In another study, patients treated with gel form of griseofulvin showed marked clinical and mycological improvement in treating tinea corporis (16). Griseofulvin hydrogel added with penetration enhancer such as propylene glycol and N-methyl-2-pyrrolidone also been reported of its anti-fungal activity without causing any skin sensitization (13). Besides, vitamin E-tocopheryl and polyethylene glycol succinate have also been used as penetration enhancer for dermal delivery of griseofulvin (11).
The in-vivo and clinical investigations suggested that it is an effective alternative to oral preparation. Recently, griseofulvin was prepared in glyceryl monostearate LN by microemulsion technique (17) and the formulation demonstrated a prolonged release profile. Besides, griseolfulvin LN was prepared using hydrogenated soybean phosphatidylcholine and dimyristoyl phosphatidylglycerol as lipid core and ethanol as solvent prior to high-pressure homogenizer (18). The freeze-dried griseofulvin LN was reported to have mean particles size of 50 nm. Nevertheless, there is no report on preparation of griseofulvin LN using palm-based lipid materials, though it has been known to be safe on human at high concentrations (19).
In view of the efficacy of LN in drug delivery for topical formulation and the urge of formulating griseofulvin in topical formulation to overcome the poor drug absorption in gastrointestinal tract and systemic side effects incurred by the oral dosage forms, we aimed to prepare a palm-based LN loaded with griseofulvin using a solvent-free technique. This investigation reports the preparation and optimisation of lipid nanoparticles loaded with griseofulvin by lipid screening, surfactant selections and lipid to surfactant ratios determination. Drug loading capacity study and stability tests of the lipid nanoparticles were conducted for the development of lipid nanoparticles based drug delivery system.
Experimental
Materials and methods
Palm-based lipids namely Lipid S (palm fatty acid), Lipid G (mixture of palm fatty acid, palm monoglycerides, diglycerides and triglycerides) and Lipid C (palm triglycerides) were provided by Malaysia Palm Oil Board (MPOB). Glycerol, Tween surfactant and methanol (HPLC grade) were obtained from Merck, Germany. Griseofulvin was obtained from Sigma-Aldrich, US.
Preparation of Lipid Nanoparticles (LN)
Lipid phase (blank or with griseofulvin) and aqueous phase: surfactant, glycerol and deionized water were heated to 70 °C separately in a water bath. Both phases were then mixed together to form a premix. The premix was subjected to a high-pressure homogenizer (HPH) (Avestin C3, Canada) at different selected cycles and pressures. The mixture was set aside at room temperature for it to recrystallize and form the LN.
Optimization of High Pressure Homogenisation (HPH) Parameters
Different types of palm-based lipid such as Lipid S, Lipid G and Lipid C were evaluated for their potential and ease to be prepared into LN. Lipid phase (1% w/w Lipid C, G or S) and aqueous phase: Tween 80 surfactant (1% w/w), glycerol (1% w/w) and deionized water (97% w/w) were used to study the effect of high-pressure homogenizer (HPH) parameters at different cycles and pressures (500-1500 bar). The formulations were subjected to photon correlation spectroscopy (PCS) study.
Selection of Lipid and Surfactant
Formulations were prepared using different lipid (lipid S, lipid G, lipid C) to Tween 80 ratios at the optimum high-pressure homogenization parameter (1500 bar and 5 cycles). The formulations were subjected to photon correlation spectroscopy analysis to select the most compatible lipid to be used in the preparation of LN formulations. After the selection of lipid, the solubilities of griseofulvin in different surfactants (Tween 20, Tween 40, Tween 60, Tween 80) were subsequently determined to select the most suitable surfactant for the preparation of griseofulvin loaded LN. The surfactant [1% (w/w)] was saturated with griseofulvin [0.05%(w/w)] and mixed with deionized water [97.95% (w/w)] and glycerol [1%(w/w)]. All ingredients were heated to 70 oC and mixed together. The premix was subjected to high-pressure homogenization at 1500 bar for 5 cycles. The mixture was then filtered through a 0.22 µM membrane filter to remove the excess griseofulvin. The concentration of griseofulvin in the solution (filtrate) was determined using a high performance liquid chromatography (HPLC) (Agilent 1260, US).
Subsequently, a series of blank and griseofulvin loaded LN were formulated using the selected lipid and surfactant. All LN was prepared at the optimum HPH parameter (1500 bar, 5 cycles) and at different lipid to surfactant (L:S) ratios, i.e. 1:4, 1:2 1:1, 1:0.5 and 1:0.33. The LN was harvested by ultracentrifugation (Beckman Coulter, US) at 40 K RPM for 3 h. The blank LN and GF-LN were reconstituted in deionized water and subjected to the PCS study.
Stability Study
Griseofulvin loaded LN was analyzed again for the PCS study after one month of storage at room temperature (25 °C) and 45 oC. The harvested GF-LN was also analysed for its drug loading capacity.
Photon Correlation Spectroscopy (PCS) Study
Zetasizer Nano ZS (Malvern Instruments, UK) with particle size limitation of 0.3 nm to 10 µM was used in this study. The samples were suitably diluted with deionized water and then subjected to the zetasizer to measure the particle size and polydispersity index.
Drug Loading Capacity Study
Drug loading capacity is the amount of griseofulvin encapsulated by the lipid. Griseofulvin loaded LN was separated from the excess griseofulvin by ultracentrifugation. The formulation was diluted 10 times with deionized water and subjected to ultracentrifugation at 40K RPM for 3 h. After ultracentrifugation, the LN formed the top layer, whereas the free griseofulvin formed a pellet at the bottom of the solution. Meanwhile, the middle portion was a clear solution free of griseofulvin, as confirmed by HPLC analysis. The LN was collected using a micropipette and freeze dried using a freeze dryer (Labconco, US).
Griseofulvin, if any, present in the lipid nanoparticles was extracted by the following method. The freeze dried LN was weighed and melted at 70 °C until the solid lipid turned into liquid oil. Warm mobile phase (70% methanol) (5 mL) was added to extract the griseofulvin with the aid of sonication (Elmasonic, Germany) for 10 min. In order to separate the lipid from the solvent, the mixture was centrifuged (Eppendorf 5810R, Germany) at 12K RPM at room temperature (25 °C) for 30 min. The supernatant was filtered and the GF content was quantified using HPLC.
The drug loading capacity was calculated using the formula below:
The drug loading capacity of the GF-LN formulations was analysed again after one month of storage at room temperature (25 °C) and 45 °C.
High Performance Liquid Chromatography (HPLC)
Condition. A modified HPLC method (20, 21) was used for the quantification of griseofulvin. A C18 column was used in the separation. The column was maintained at 25 °C and a mixture of 70:30 methanol and water was used as the mobile phase with a flow rate of 1 mL/min. The mobile phase was filtered with 0.45 µm (nylon) membrane filter and degassed prior to use. Ultra-violet (UV) detector was set at the absorption wavelength of GF at 293 nm.
Calibration. Six known concentrations of griseofulvin were prepared and their HPLC peak areas were determined. A standard stock solution of griseofulvin (100 µgmL-1) was prepared by dissolving 5 mg of griseofulvin in 50 mL of mobile phase. Three sets of calibration standards were prepared from the independent stock solutions. The peak areas were plotted against concentrations to obtain the calibration curve.
Validation. For the validation of accuracy and precision of the HPLC method, three quality control (QC) standards at three levels namely low quality control (LQC), middle quality control (MQC), and high quality control (HQC) were prepared. The LQC, MQC and HQC were at 0.9 µgmL-1, 12.5 µgmL-1 and 18.8 µgmL-1 respectively. The criterion for accuracy is set to be within the range of 95% to 105%, whereas precision requires a coefficient of variation (CV) within 5% (22).
Statistical Analysis
Statistical analysis was performed using GraphPad Prism version 5.00.
Results and Discussion
Optimization of High Pressure Homogenization (HPH) Parameters
Palm-based lipid was used as lipid core in this study as it also serves as an emollient that soothes and moisturizes the skin especially at fungal infected area, which is commonly unsightly and dries (19). Lipid S, Lipid G and Lipid C were first used to prepare blank LN formulations by varying different cycles and pressures (500-1500 bar) of the high-pressure homogenizer. High-pressure homogenization is a well accepted and widely used method for the preparation of LN (23, 24, 25). The main principles involved are high shear stress and cavitation (26). Lipid S and lipid G formulations were subjected to PCS study. However, the zetasizer was not able to record reading for these formulations as the particles size exceeded the measurement capability of the zeta sizer, stated the presence of big particles. On the other hand, LN formulations prepared from Lipid C were able to be analysed by the zetasizer (Table 1). Statistical analysis using One-way ANOVA followed by Tukey’s post hoc analysis showed a significant reduction (p<0.001) in particle size with increasing pressure and cycle of homogenization. The smallest particle size of 112 ± 5.8 nm was produced at 1500 bar, and 5 cycles of homogenization. It has been reported that high-pressure homogenization setting with 3 to 5 cycles and 500 to 1500 bar are sufficient to produce LN with submicron size (27). The polydispersity index (PDI) of all the lipid C formulations was ranged from 0.263 to 0.443. It has been reported that PDI < 0.05 represents a very narrow distribution (28); PDI < 0.2 represents narrow size distribution (29) and PDI > 0.7 indicates a very broad particle size distribution (28) in lipid nanoparticles. In this study, lipid C produced LN with neither narrow (PDI < 0.05) nor very broad (PDI >0.7) particle size distribution. Cho et al. reported on docetaxel-solid LN with neither narrow nor broad particle size distribution (PDI ranging from 0.191 to 0.214) and the LN demonstrated in-vitro sustained release profile, enhanced performance in drug absorption and in-vivo bioavailability (28). The optimum high-pressure homogenization parameters were therefore standardized at 1500 bar with 5 cycles.
Macroscopic Evaluation. Pearling was shown on all lipid S and lipid G formulations prepared with different HPH parameters. When particles tend to arrange in a chain like manner in pearling, their shape transformation is similar to Rayleigh instability (30). The uncontrollable breakage of the pearl structure challenges the stability of the formulation, as reported in a study of an originally oblate lipid vesicle with the insertion of amphiphilic polymer. Pearling has also been reported to be metastable and evolving where they joined to form a larger sphere and intersected to cause destabilization (31). When lipid C was used in formulating lipid nanoparticles, there was no pearling observed in all lipid C formulations.
Selection of Lipid and Surfactant
Formulations of lipid G-LN and lipid S-LN again showed pearling at all ratios but not in lipid C-LN formulations (Table 2). The zetasizer also failed to record the particles size of Lipid G-LN and lipid S-LN formulations at all lipid to surfactant ratio stating the presence of big particle. Lipid C formulations at different ratio were recorded in nano-sized with PDI less than 0.5. Therefore, Lipid C was selected for the subsequent studies.
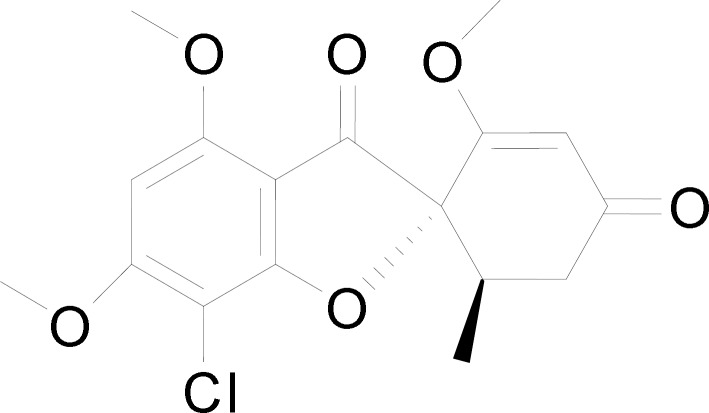
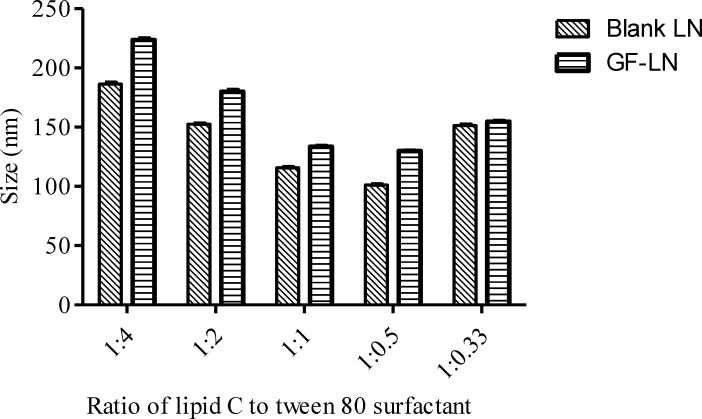
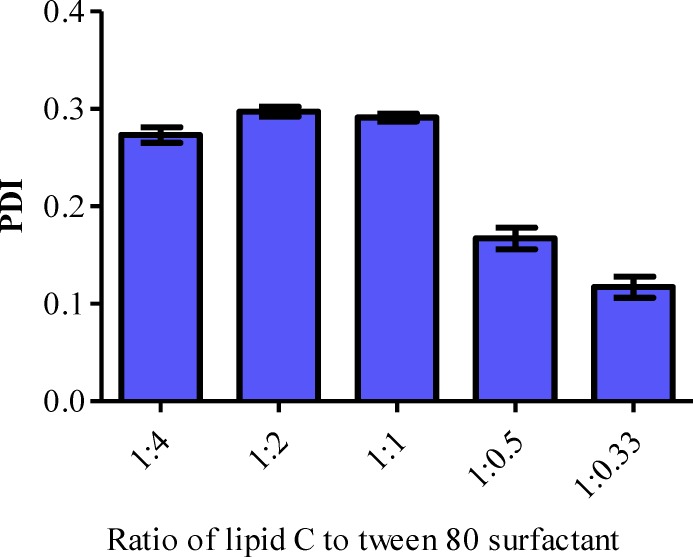
The effect of high pressure homogenization on particle size and polydispersity index of lipida nanoparticles. N = 3
Cycle(s) | Pressure (bar) | Particle size (nm) | Polydispersity index |
---|---|---|---|
3 | 500 | 174.9 ± 4.1 | 0.263 ± 0.006 |
3 | 1000 | 142.7 ± 0.9 | 0.276 ± 0.009 |
3 | 1500 | 131.4 ± 2.6 | 0.279 ± 0.007 |
1 | 1500 | 214.5 ± 13.2 | 0.443 ± 0.041 |
5 | 1500 | 112.0 ± 5.8 | 0.294 ± 0.006 |
Macroscopic observation, particle size and polydispersity index of preliminary formulations prepared using Lipid Sa, Lipid Gb and Lipid Cc.
Lipid | Preliminaryformulation | L:S | Lipid(%) | Tween 80 (%) | Glycerol(%) | Deionisedwater(%) | Macroscopicobservation | Particlesize (nm) Mean ±SD | Polydispersityindex |
---|---|---|---|---|---|---|---|---|---|
Lipid S | PS0 | 1:0 | 1 | - | - | 99 | Pearling | - | - |
PS1 | 1:4 | 0.25 | 1 | 1 | 97.75 | Pearling | >10000 | >10000 | |
PS2 | 1:2 | 0.5 | 1 | 1 | 97.5 | Pearling | >10000 | >10000 | |
PS3 | 1:1 | 1 | 1 | 1 | 97 | Pearling | >10000 | >10000 | |
PS4 | 1:0.5 | 2 | 1 | 1 | 96 | Pearling | >10000 | >10000 | |
PS5 | 1:0.33 | 3 | 1 | 1 | 95 | Pearling | >10000 | >10000 | |
Lipid G | PG0 | 1:0 | 1 | - | - | 99 | Pearling | - | - |
PG1 | 1:4 | 0.25 | 1 | 1 | 97.75 | Pearling | >10000 | >10000 | |
PG2 | 1:2 | 0.5 | 1 | 1 | 97.5 | Pearling | >10000 | >10000 | |
PG3 | 1:1 | 1 | 1 | 1 | 97 | Pearling | >10000 | >10000 | |
PG4 | 1:0.5 | 2 | 1 | 1 | 96 | Pearling | >10000 | >10000 | |
PG5 | 1:0.33 | 3 | 1 | 1 | 95 | Pearling | >10000 | >10000 | |
Lipid C | PC0 | 1:0 | 1 | - | - | 99 | No Pearling | - | - |
PC1 | 1:4 | 0.25 | 1 | 1 | 97.75 | No Pearling | 136.6±4.2 | 0.462±0.045 | |
PC2 | 1:2 | 0.5 | 1 | 1 | 97.5 | No Pearling | 138.9±2.8 | 0.325±0.037 | |
PC3 | 1:1 | 1 | 1 | 1 | 97 | No Pearling | 112.2±5.4 | 0.278±0.008 | |
PC4 | 1:0.5 | 2 | 1 | 1 | 96 | No Pearling | 133.2±0.8 | 0.153±0.017 | |
PC5 | 1:0.33 | 3 | 1 | 1 | 95 | No Pearling | 128.1±4.9 | 0.154±0.018 |
The effect of lipida to surfactant ratio on photon correlation spectroscopy analysis for blank formulation. N = 3.
Formulation | Percentage, % (w/w) | Photon Correlation Spectroscopy | |||||
---|---|---|---|---|---|---|---|
Lipid | Tween | Glycerol | Deionised | Size (nm) | PDI | Zeta potential | |
A (1:4) | 0.25 | 1 | 1 | 97.75 | 186.3 ± 3.2 | 0.273 ± 0.008 | -28.7 ± 1.9 |
B (1:2) | 0.5 | 1 | 1 | 97.50 | 152.6 ± 1.3 | 0.297 ± 0.005 | -27.6 ± 1.8 |
C (1:1) | 1 | 1 | 1 | 97.00 | 115.7 ± 1.2 | 0.291 ± 0.004 | -27.9 ± 2.0 |
D (1:0.5) | 2 | 1 | 1 | 96.00 | 101.1 ± 1.3 | 0.167 ± 0.011 | -26.7 ± 1.5 |
E (1:0.33) | 3 | 1 | 1 | 95.00 | 151.3 ± 2.7 | 0.117 ± 0.011 | -25.7 ± 1.2 |
The effect of lipida to surfactant ratio on photon correlation spectroscopy analysis for griseofulvin lipid nanoparticles. N = 3
Formulation(Lipid: | Percentage, % (w/w) | Photon Correlation Spectroscopy Mean ± SD | ||||||
---|---|---|---|---|---|---|---|---|
Surfactant Ratio) | Lipid | Tween | Glycerol | Griseofulvin | Deionised | Size (nm) | PDI | Zeta potential(mV) |
A (1:4) | 0.25 | 1 | 1 | 0.05 | 97.70 | 223.7 ± | 0.306 ± | -29.0 ± 2.2 |
B (1:2) | 0.5 | 1 | 1 | 0.05 | 97.45 | 179.8 ± | 0.306 ± | -27.9 ± 1.6 |
C (1:1) | 1 | 1 | 1 | 0.05 | 96.95 | 133.4 ± | 0.302 ± | -27.4 ± 2.2 |
D (1:0.5) | 2 | 1 | 1 | 0.05 | 95.95 | 129.8 ± | 0.214 ± | -26.2 ± 1.4 |
E (1:0.33) | 3 | 1 | 1 | 0.05 | 94.95 | 154.7 ± | 0.152 ± | -25.9 ± 0.9 |
Stability studies of the lipida nanoparticles at different lipid to surfactantb ratios. N = 3.
Formulation(Lipid:Surfactant Ratio) | Drug loading capacity(%) | Photon Correlation Spectroscopy Mean ± SD | ||||||||||||||||
---|---|---|---|---|---|---|---|---|---|---|---|---|---|---|---|---|---|---|
Day 1 | After 1 month storage at | After 1 month storage at | Day 1 | After 1 month storage at 25 oC | After 1 month storage at 45 oC | |||||||||||||
Size (nm) Mean | PDI | Zeta potential (mV) | Size (nm) Mean | PDI | Zeta potential (mV) | Size (nm) Mean | PDI | Zeta potential (mV) | ||||||||||
A (1:4) | 0.672 | 0.670 | 0.664 | 223.7 | 0.306 | -29.0 | 0.664 | 0.670 | 223.7 | -29.0 | 0.670 | 0.306 | ||||||
±0.016 | ±0.008 | ±0.048 | ±3.3 | ±0.015 | ± 2.2 | ±0.048 | ±0.008 | ±3.3 | ± 2.2 | ±0.008 | ±0.015 | |||||||
B (1:2) | 0.772 | 0.770 | 0.770 | 179.8 | 0.306 | -27.9 | 0.770 | 0.770 | 179.8 | -27.9 | 0.770 | 0.306 | ||||||
±0.028 | ±0.008 | ±0.013 | ±4.9 | ±0.011 | ±1.6 | ±0.013 | ±0.008 | ±4.9 | ±1.6 | ±0.008 | ±0.011 | |||||||
C (1:1) | 0.724 | 0.716 | 0.711 | 133.4 | 0.302 | -27.4 | 0.711 | 0.716 | 133.4 | -27.4 | 0.716 | 0.302 | ||||||
±0.029 | ±0.010 | ±0.017 | ±1.9 | ±0.007 | ±2.2 | ±0.017 | ±0.010 | ±1.9 | ±2.2 | ±0.010 | ±0.007 | |||||||
D (1:0.5) | 0.624 | 0.616 | 0.586 | 129.8 | 0.214 | -26.2 | 0.586 | 0.616 | 129.8 | -26.2 | 0.616 | 0.214 | ||||||
±0.018 | ±0.032 | ±0.010 | ± 1.0 | ±0.009 | ± 1.4 | ±0.010 | ±0.032 | ± 1.0 | ± 1.4 | ±0.032 | ±0.009 | |||||||
E (1:0.33) | 0.441 | 0.424 | 0.386 | 154.7 | 0.152 | -25.9 | 0.386 | 0.424 | 154.7 | -25.9 | 0.424 | 0.152 | ||||||
±0.010 | ±0.014 | ±0.030 | ±1.9 | ±0.008 | ± 0.9 | ±0.030 | ±0.014 | ±1.9 | ± 0.9 | ±0.014 | ±0.008 |
Tween (polysorbate), a non-ionic surfactant is known to be a milder irritant than the anionic and cationic surfactants (32). Surfactant or surface tension reducing agent is able to stabilize an emulsion. It can also act as a co-solvent that improves the entrapment of drug in the lipid nanoparticles (33). Tween surfactant is widely recognized as a safe surfactant in the drug delivery formulation (34, 35). Hence, Tween series surfactants (Tween 20, 40, 60 and 80) with increasing alkyl chain length were used in this study. It has been reported that drug solubility improves with increasing alkyl chain length of the Tween surfactant if the solubility takes place in the core of the micelle structure (36). Nevertheless, the solubility of griseofulvin did not demonstrate an obvious trend of increment with the increase of alkyl chain length of the Tween surfactants. The solubility of griseofulvin in Tween 20, 40, 60 and 80 were recorded as 47.5 ± 2.29 µgmL-1, 50.1 ± 2.15 µgmL-1, 44.2 ± 1.82 µgmL-1 and 53.1 ± 2.16 µgmL-1 respectively. The solubility of griseofulvin could happen in between the hydrophobic core, the core-surface interface of the micelles and in the palisade layer (36). As reported, non-ionic surfactants such as Tween surfactant can facilitate solubility at the palisade layer. Among all the Tween surfactants, the saturation solubility of griseofulvin in Tween 80 showed the highest concentration of griseofulvin and therefore was selected to be used in the studies.
Blank LN. Blank LN formulations were prepared by different ratios of lipid C and Tween 80 surfactant and subjected to PCS analysis. The data were analysed using One-way ANOVA followed by Tukey’s post hoc analysis (Table 3). In LN formulations, higher concentration of surfactant is associated with smaller particle size (37). This was observed in the preparation of blank LN formulations D (Lipid: Tween 80 surfactant=1:0.5) and E (Lipid: Tween 80 surfactant=1:0.33), whereby the increase in the lipid concentration from 2% to 3% resulted in a significant (p<0.001) increment in the particle size of the lipid nanoparticles (Figure 2). However, beyond the optimum level of the surfactant to emulsify the lipid, there was no further improvement in the particle size (29). The particle size decreased significantly (P < 0.001) from formulation A (Lipid: Tween 80 surfactant = 1:4) to D when the relative amount of surfactant decreased. An increment in the particle size and PDI might be caused by the multilayer on the particle surface formed by the excessive surfactant (38). In addition, the excess surfactants might migrate from the LN interface to form surfactant vesicles, contributing to the increment in particle size (5). This is also in agreement with a report on isotretinoin-loaded LN formulated using stearic acid, glycerylmonostearate, glyceryldistearate, and glyceryldibehnate as lipid phase (39). While maintaining the surfactant (Tween 80) concentration at 5% and increase in the lipid concentration from 7.5% to 10%, the particle size was recorded to be reduced from 359 ± 9 nm to 292 ± 10 nm. However, further increase in the lipid concentration from 5% to 7.5% resulted in an increase of particle size (200 ± 18 nm to 359 ± 9 nm).
The PDI of the formulations A (Lipid: Tween 80 surfactant = 1:4), B (Lipid: Tween 80 surfactant = 1:2) and C (Lipid: Tween 80 surfactant = 1:1) were in the ranged of 0.273, 0.297 and 0.291 respectively. The PDI was significantly decreased when the ratio of lipid and surfactant were further reduced to 1:0.5 (D) and 1:0.33 (E) (Figure 3). According to the Derjaguin-Landau-Verwey-Overbeek (DLVO) theory, the stability of colloidal dispersion is governed by the van der Waals attraction and electrostatic repulsion (40). Hence, the zeta potential of the LN should be as high as possible regardless of its charge, to repel the particles from each other in order to maintain the size and stability. The zeta potential of a stable LN preparation should be at least ± 25 mV (38). It has also been reported that a minimum of ± 30 mV is required for a good physical stability (41). All the blank formulations prepared were categorized as stable since their zeta potential was ranged between -25 mV to around -29 mV (Table 3).
Griseofulvin loaded LN (GF-LN). Griseofulvin was incorporated into the LN and it was found that the incorporation of griseofulvin into the LN did not affect the stability of the LN as it did not lead to any significant changes in the zeta potential (Table 4). All of the preparations were in submicron range and their PDI was still maintained below 0.7. Cho et al. reported that LN with PDI > 0.7 indicates a broad particle size distribution (28). As expected, the particle size of the lipid nanoparticle increased significantly (p < 0.001) after the incorporation of griseofulvin except for formulation E, in which the particle size remained unchanged at around 150 nm (Table 4 and Figure 2). The PDI of all the griseofulvin loaded formulations were significantly higher than that of the blank formulation but still maintained a PDI of less than 0.7 (p < 0.001), except for formulation B with p < 0.05.
Stability Study. The stability of GF-LN upon storage for 1 month at room temperature (25 oC) and 45 oC was monitored (Table 5). There was no significant change in the particle size upon storage except for formulations D and E. There was no significant change in particle size for the latter two formulations after 1-month storage at room temperature (25 oC), but their particle sizes were significantly higher (p < 0.001) after 1-month storage at 45 oC. For PDI, there was no significant difference for formulations A and B after 1-month storage at 25 and 45 °C. However, the PDI increased significantly after storing at 45 oC for formulations C and D (p < 0.001). As for formulation E, the particle size increased significantly upon storage at room temperature (25 oC) (p < 0.05) and 45 oC (p < 0.001). There was no significant difference in the zeta potential of formulations A, B and C upon storage for 1 month at both 25 oC and 45 oC. The zeta potential of formulation D was significantly lower (p < 0.001) upon storage at high temperature (45 oC) for 1 month. Meanwhile, the zeta potential of formulation E reduced significantly (p < 0.001) after 1-month storage at both 25 oC and 45 oC. To conclude the stability study of griseofulvin loaded LN, formulations A and B were stable without significant difference in particle size, PDI and zeta potential upon storage for 1 month at 25 oC and 45 oC.
Drug Loading Capacity Study
The drug loading capacities of the formulations was determined by the established HPLC method. The results obtained in drug loading study were analyzed with One-way ANOVA followed by Tukey’s post hoc analysis. In this study, excess griseofulvin was added to maximize the incorporation. High-pressure homogenizer was used to reduce the particle size of griseofulvin. Such physical modifications allowed greater surface area to volume ratio thus improved the solubility of griseofulvin (42). Hydrophobicity nature of griseofulvin causes it to favor the lipid phase. Thus, as the lipid concentration increased from 0.25% (Formulation A) to 0.5% (Formulation B), the loading capacity of griseofulvin improved from 0.672 ± 0.016% to 0.772 ± 0.028% (Table 5). Further increase of the lipid content to 1% (Formulation C) led to an insignificant change in the loading capacity of GF (0.724 ± 0.029%). Also, the loading capacity decreased when the lipid concentration increased from 1% (Formulation C) to 3% (Formulation E). This could be due to the increased viscosity that caused a decrease in the efficiency of the homogenizer to further reduce the particle size of griseofulvin to facilitate its solubility and incorporation (43).
Formulation B was chosen as the optimum formulation as its drug loading percentage was significantly the highest (p < 0.01 compared to formulation A; p < 0.001 compared to Formulations D and E). Although its drug loading percentage was insignificantly difference from formulation C, however formulation B consumed a lower percentage of lipid (lesser material). Besides, all of the formulations were stable (no significant change) in terms of drug loading percentage after one-month storage at 25 oC and 45 oC.
Conclusion
LN loaded with griseofulvin was successfully prepared from palm-based materials using the simple hot high-pressure homogenization technique. The optimized formulation was obtained from a series of screening and optimisation works including HPH parameters optimization, lipid and surfactant selection and lipid to surfactant ratio optimization in relation to their effects on particle size, formulation stability as well as drug loading capacity. This study suggests that the palm-based triglycerides serve as a potential lipid core of LN for drug delivery. Further studies such as cytotoxicity, antifungal susceptibility tests and skin penetration and retention studies will be reported in another report on the efficacy of the palm-based LN loaded with griseofulvin as topical dosage form.
Acknowledgements
References
-
1.
Muller RH, Mader K, Gohla S. Solid lipid nanoparticles (SLN) for controlled drug delivery - a review of the state of the art. Eur. J. Pharm. Biopharm. 2000;50:161-77. [PubMed ID: 10840199].
-
2.
Pardeike J, Hommoss A, Muller RH. Lipid nanoparticles (SLN, NLC) in cosmetic and pharmaceutical dermal products. Int. J. Pharm. 2009;366:170-84. [PubMed ID: 18992314].
-
3.
Guimaraes K, Re M. Lipid nanoparticles as carriers for cosmetic ingredients: The first (SLN) and the second generation (NLC). In: Beck R, Guterres S, PohlmannA, editors. Nanocosmetics and Nanomedicines. Berlin Heidelberg: Springer; 2011.
-
4.
Agrawal Y, Petkar KC, Sawant KK. Development, evaluation and clinical studies of Acitretin loaded nanostructured lipid carriers for topical treatment of psoriasis. Int. J. Pharm. 2010;401:93-102. [PubMed ID: 20858539].
-
5.
Raza K, Singh B, Singal P, Wadhwa S, Katare OP. Systematically optimized biocompatible isotretinoin-loaded solid lipid nanoparticles (SLNs) for topical treatment of acne. Colloids & Surfaces B: Biointerfaces. 2013;105:67-74. [PubMed ID: 23357735].
-
6.
Wissing SA, Muller RH. The influence of solid lipid nanoparticles on skin hydration and viscoelasticity-in vivo study. Eur. J. Pharm. Biopharm. 2003;56:67-72. [PubMed ID: 12837483].
-
7.
Cevc G, Vierl U. Nanotechnology and the transdermal route: A state of the art review and critical appraisal. J. Control Release. 2010;141:277-99. [PubMed ID: 19850095].
-
8.
Oxford AE, Raistrick H, Simonart R. Studies in the biochemistry of micro-organisms: Griseofulvin, C(17)H(17)O(6)Cl, a metabolic product of Penicillium griseo-fulvum Dierckx. Biochem. J. 1939;33:240-8. [PubMed ID: 16746904].
-
9.
Gentles JC. Experimental ringworm in guinea pigs: oral treatment with griseofulvin. Nature. 1958;182:476-7. [PubMed ID: 13577889].
-
10.
Griffiths. WHO Model Prescribing Information - Drugs Used in Skin Diseases. Clin. Exp. Dermatol. 1999;24:81-2. [PubMed ID: 10233659].
-
11.
Aggarwal N, Giondi S. Preparation and evaluation of antifungal efficacy of griseofulvin loaded deformable membrane vesicles in optimized guinea pig model of Microsporum canis—Dermatophytosis. Int. J. Pharm. 2012;437:277-87. [PubMed ID: 22939964].
-
12.
Mhatre PA, Patil RN, Kumar RS. Formulation, development and evaluation of topical emulgel of griseofulvin. An Int. J. Adv. Pharm. Sci. 2014;5:2298-308.
-
13.
Shishu, Aggarwal N. Preparation of hydrogels of griseofulvin for dermal application. Int. J. Pharm. 2006;326:20-4. [PubMed ID: 16920284].
-
14.
Chakraborty S, Shukla D, Mishra B, Singh S. Lipid--an emerging platform for oral delivery of drugs with poor bioavailability. Eur. J. Pharm. Biopharm. 2009;73:1-15. [PubMed ID: 19505572].
-
15.
Nimni ME, Ertl D, Oakes. Distribution of griseofulvin in the rat: comparison of the oral and topical route of administration. J. Pharm. Pharmacol. 1990;42:729-31. [PubMed ID: 1982148].
-
16.
Kassem MA, Esmat S, Benda ER, El-Komy MH. Efficacy of topical griseofulvin in treatment of tinea corporis. Mycoses. 2006;49:232-5. [PubMed ID: 16681816].
-
17.
Lorwongngam A, Chansiri G, Dangprasert P, Kongmuang S. Griseofulvin solid lipid nanoparticles based on microemulsion technique. Adv. Mater. Res. 2011:197-198. 47-50.
-
18.
Kamiya S, Kurita T, Miyagishima A, Itai S, Arakawa M. Physical properties of griseofulvin-lipid nanoparticles in suspension and their novel interaction mechanism with saccharide during freeze-drying. Eur. J. Pharm. Biopharm. 2010;74:461-6. [PubMed ID: 20018239].
-
19.
Fife B. The Palm Oil Miracle. Piccadilly Books. Colo, USA: Colorado Springs; 2007.
-
20.
Fujii M, Bouno M, Fuita S, Yoshida M, Watanabe Y, Matsumoto M. Preparation of griseofulvin for topical application using N-methyl-2-pyrrolidone. Biol. Pharm. Bull. 2000;23:1341-5. [PubMed ID: 11085363].
-
21.
Venkatadasu V, Muralidhar RV, Panda T. Analytical techniques for griseofulvin. Bioprocess Eng. 2000;22:201-4.
-
22.
Bauer LC, Santana DDA, Macedo MDS, Torres AG, Souza, NED, Simionato JI. Method validation for simultaneous determination of cholesterol and cholesterol oxides in milk by RP-HPLC-DAD. J. Brazilian Chem. Soc. 2014;25:161-8.
-
23.
Jenning V, Schafer-korting M, Gohla S. Vitamin A-loaded solid lipid nanoparticles for topical use: drug release properties. J. Controlled Release. 2000;66:115-26.
-
24.
Muller RH, Runge S, Ravelli V, Mehnert W, Thunemann AF, Souto EB. Oral bioavailability of cyclosporine: Solid lipid nanoparticles (SLN®) versus drug nanocrystals. Int. J. Pharm. 2006;317:82-9. [PubMed ID: 16580159].
-
25.
Asawale R, Rupali HA, Jayant HM, Vijay BK. Solid lipid nanoparticle as drug delivery system: An overview. Pharmacie Globale: Int. J. Comprehensive Pharm. 2014;5:1.
-
26.
Cho H J, Park JW, Yoon IS, Kim DD. Surface-modified solid lipid nanoparticles for oral delivery of docetaxel: enhanced intestinal absorption and lymphatic uptake. Int. J. Nanomedicine. 2014;9:495-504. [PubMed ID: 24531717].
-
27.
Das S, Ng WK, Tan RBH. Are nanostructured lipid carriers (NLCs) better than solid lipid nanoparticles (SLNs): Development, characterizations and comparative evaluations of clotrimazole-loaded SLNs and NLCs? Eur. J. Pharm. Sci. 2012;47:139-51.
-
28.
Yu Y, Granick S. Pearling of lipid vesicles induced by nanoparticles. J. Am. Chem. Soc. 2009;131:14158-9. [PubMed ID: 19775107].
-
29.
Bar-ziv R, Moses E, Nelson P. Dynamic excitations in membranes induced by optical tweezers. Biophysic. J. 1998;75:294-320.
-
30.
Som I, Bhatia K, Yasir M. Status of surfactants as penetration enhancers in transdermal drug delivery. J. Pharm. Bioallied Sci. 2012;4:2-9. [PubMed ID: 22368393].
-
31.
Abdelbary G, Fahmy RH. Diazepam-loaded solid lipid nanoparticles: design and characterization. AAPS Pharm. Sci. Tech. 2009;10:211-9.
-
32.
Helgason T, Awas TS, Kristbergsson K, Decker EA, Mcclements DJ, Weiss J. Impact of surfactant properties on oxidative stability of beta-carotene encapsulated within solid lipid nanoparticles. J. Agric. Food Chem. 2009;57:8033-40. [PubMed ID: 19691283].
-
33.
Florence AT, Attwood D. Physicochemical Principles of Pharmacy. London: Pharmaceutical Press; 2011.
-
34.
Liu J, Hu W, Chen H, Ni Q, Xu H, Yang X. Isotretinoin-loaded solid lipid nanoparticles with skin targeting for topical delivery. Int. J. Pharm. 2007;328:191-5. [PubMed ID: 16978810].
-
35.
Mehnert W, Mader K. Solid lipid nanoparticles: Production, characterization and applications. Adv. Drug Delivery Rev. 2001;47:165-196.
-
36.
Ghorab MM, Abdel-Salm HM, Abdel-Moaty MM. Solid lipid nanoparticles-Effect of lipid matrix and surfactant on their physical characteristics. Bull. Pharm. Sci. 2004;27:155-9.
-
37.
Wei C-C, Ge Z-Q. Influence of electrolyte and poloxamer 188 on the aggregation kinetics of solid lipid nanoparticles (SLNs). Drug Devp. Ind. Pharm. 2012;38:1084-1089.
-
38.
Freitas C, Muller. Effect of light and temperature on zeta potential and physical stability in solid lipid nanoparticle (SLN™) dispersions RH. Int. J. Pharm. 1998;168:221-229.
-
39.
Savjani KT, Gajjar AK, Savjani JK. Drug solubility: importance and enhancement techniques. ISRN Pharm. 2012:195727. [PubMed ID: 22830056].
-
40.
Martins S, Thoi I, Souto E, Ferrreira D, Brandi M. Multivariate design for the evaluation of lipid and surfactant composition effect for optimisation of lipid nanoparticles. Eur. J. Pharm. Sci. 2012;45:613-23. [PubMed ID: 22245538].
-
41.
Freitas C, Muller. Effect of light and temperature on zeta potential and physical stability in solid lipid nanoparticle (SLN™) dispersions RH. Int. J. Pharm. 1998;168:221-229.
-
42.
Savjani KT, Gajjar AK, Savjani JK. Drug solubility: importance and enhancement techniques. ISRN Pharm. 2012:195727. [PubMed ID: 22830056].
-
43.
Martins S, Thoi I, Souto E, Ferrreira D, Brandi M. Multivariate design for the evaluation of lipid and surfactant composition effect for optimisation of lipid nanoparticles. Eur J. Pharm. Sci. 2012;45:613-23. [PubMed ID: 22245538].