Abstract
Keywords
Peganum harmala Parkinson’s disease 6-hydroxydopamine angiotensin converting enzyme rat
Introduction
Parkinson's disease (PD) is the most prevalent neurodegenerative disease after Alzheimer's disease (1). In PD the basal ganglia cells and substantia nigra (SN) cells are destroyed and then the level of dopamine is decreased (2).
Increasing evidence showed the role of oxidative stress as a pathogenic factor in PD (3). Oxidative stress is the release of reactive oxygen species (ROS). Some of the most important causes of oxidative stress are aging, genetic factors, metals, changing in vital macromolecules, diet, lifestyle, free radicals, poisons, and Angiotensin II (4, 5).
Angiotensin converting enzyme (ACE) converts angiotensin І to angiotensin П. Angiotensin П activates oxidases related to NADPH and creates superoxide (4, 6). SN and striatum have higher ACE activity compared to other brain regions, and AT1 receptors co-localize with nigral dopamine neurons (7-9). Angiotensin П creates ROS by AT1 receptors and destroys the dopaminergic neurons, so manipulation of renin angiotensin system (RAS) may be effective in treatment of PD (10). ACE inhibitors like perindopril and captopril and angiotensin II receptor blockers like losartan had neuroprotective effect in the striatum and the SN in rats receiving 6-hydroxydopamine (6-OHDA) (11-13).
Aqueous extract of Peganum harmala's seeds (AEPHS) showed ACE inhibitory effect in-vitro (14, 15). This study examined possible anti-Parkinsonism effect of AEPHS compared to captopril in 6-OHDA induced PD in rats.
Experimental
Animals
Male Wistar rats 200-250 g, were allocated in the 4 groups. In each group 6 rats were killed for biochemical tests and measurement of ACE activity 24 h after the 6-OHDA neurotoxin injection and the 6 others were kept for behavioral tests and histopathological study for 2 weeks after the 6-OHDA injection. All experiments were performed in accordance with the National Institutes of Health Guide for the Care and Use of Laboratory Animals.
1) Toxin group: their left hemisphere’s substantia nigra (SN) was destroyed by 6-OHDA.
2) Control group: or sham operated group, was received normal saline instead of AEPHS or 6-OHDA.
3) Peganum harmala group: AEPHS (10mg/kg) was injected i.p -144, -120, -96, -72, -48, -24, -2, 4 and 24 h relative to injection time of 6-OHDA into SN.
4) Captopril group: captopril (5mg/kg) was injected i.p -144, -120, -96, -72, -48, -24, -2, 4 and 24 h relative to injection time of 6-OHDA into SN.
Aqueous extraction of Peganum harmala seeds
100 grams of dried plant's seeds was poured into 1 liter boiling water in a beaker and kept in room temperature for 2 h. After that the solution was filtered and freeze-dried.
Parkinsonism induction
Each rat was anaesthetized by i.p injection of 100 mg/kg ketamin and 5 mg/kg xylazine and then his head was fixed on stereotaxic device (Stoelting, USA). Stereotaxic parameters for SN: AP: -4.8 mm to brigma, ML (left): 2 mm, DV: -8.3 mm from the surface of scalp by Watson & Paxinos atlas. 4μL of toxin (2mg/mL 6-OHDA with 0.1% vitamin C in normal saline) was injected by Hamilton syringe at a rate of 1 μl/min (16).
Rotation test
We tested rats' unidirectional rotation test induced by apomorphine hydrochloride (2.5 mg/kg) in PD rats. Whole (right-sided minus left-sided) rotation in a cylinder box (33 cm diameter, 35 cm height) was measured in an isolated room in a 60 min. period.
Murprogo's test
This is a method to measure muscle stiffness (17), by laying the animal on a flat surface the rat received a score of 0.5 if it did not move when touched. After that the right paw of the rat was laid on the edge of a box with 3 cm height. If the animal did not take its paw off after 10 sec, it received a score of 0.5. The same method was used for the left hand. In the next step, only the right paw of the rat was placed on the edge of a box with a height of 9 cm. If the rat did not take its paw off after 10 s, it received a score of 1. The last step was repeated for the left hand of the rats. The sum of the scores of movement on the floor and movement of hands while being hanged on the edge of boxes with 3 cm and 9 cm heights was 3.5.
ACE enzyme activity in serum blood and brain tissue homogenate
Brains were kept in -80ºC freezers until analysis time. Brain tissue was homogenated and 10 μl of homogenate was incubated with 40 μl substrate (hippuryl histidyl leucine) in a thermo-mixer (eppendorf- MTP model) for 30 min. in 37˚C and 300 rpm. After that, 150 μl phosphoric acid (5M) was added to each well to stop the reaction. 20 μl of the reactant mixture was injected into HPLC (Shimadzu, pump: LC-10ADVP, control system: SCL-10AVP, detector: SPD-10AV) and area under the curve of hippuric acid (enzyme product) was detected in 228 nm with 1 mL/min flow rate of mobile phase consisting of 1: 1 methanol: KH2PO4 0.1M, pH = 3. One unit of enzyme activity was defined as: 1 nmol of hippuric acid produced in one mg of brain tissue protein in one min in 37˚C.
Lipid peroxidation
Lipid peroxidation was tested by complex formation between malondialdehyde and thiobarbitoric acid. Thiobarbitoric acid reactive substances (TBARS) were measured by spectrophotometer at 532 nm (5).
Protein concentration was measured by Bradford method with BSA (bovine serum albumin) as standard (18).
Protein oxidation
Protein oxidation was tested by measuring the concentration of carbonyl groups of proteins. Carbonyl group content of protein was assayed by spectrophotometer at 370 nm (19). Carbonyl group concentration was calculated based on e = 22 mM-1cm-1.
Histology examination
After decapitation, 5 to 8 cut of SN was processed for the number of dopaminergic neurons. We counted Nissl-stained dopaminergic neurons in the substantia nigra pars compacta and substantia nigra pars reticulate region in left and right hemispheres at 200x zoom.
Chemicals used in experiments
1, 1, 3, 3-Tetraethoxy propane, 2, 4- Dinitrophenyl Hydrazine, Apomorphine hydrochloride, Cresyle violet acetate, Guanidine hydrochloride, Hippuryl-His-Leu, Streptomycin sulfate, Tritonx-100, Desferrioxamine, and 6-hydroxydopamine were purchased from Sigma-Aldrich. Ketamine, Xylazine, Magnesium acetate tetrahydrate, Sucrose, Thiobarbituric acid, and Trichloro acetic acid were obtained from Merck.
Statistical analysis
Because of failure of normality distribution, we used non parametric Kruskal-Wallis test, and comparisons between 2 groups were made by Mann-Whitney U test. All analysis were done by IBM SPSS Statistics ver. 20.
Results
Number of unilateral rotation
Left handed rotation in 1 h period in toxin group was significantly higher than the control and two treatment groups (Figure1).
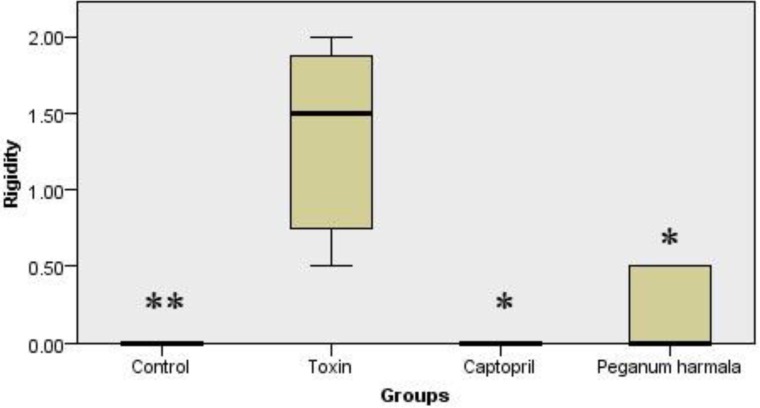
Murprogo's test
Rigidity was significantly higher in toxin group compared to other groups (Figure 2).
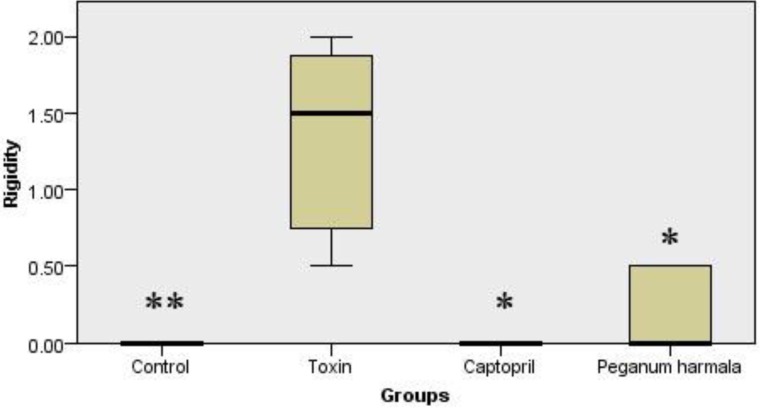
Lipid peroxidation
Malondialdehyde production as a lipid peroxidation index was significantly higher in toxin group than other groups (Figure 3).
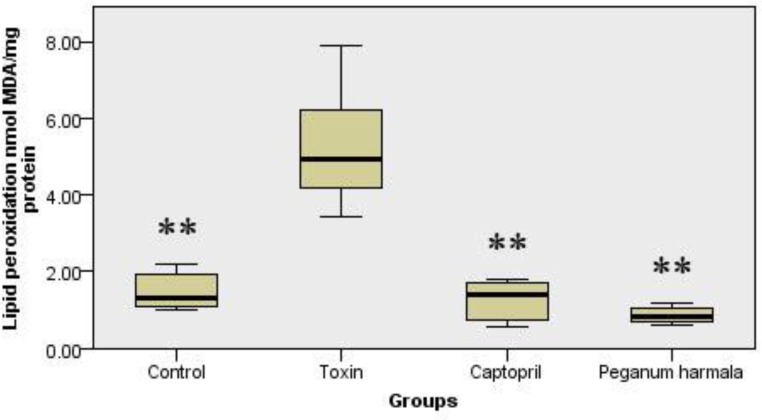
Protein oxidation
Protein oxidation in Peganum harmala group was significantly lower than toxin group (Figure 4).
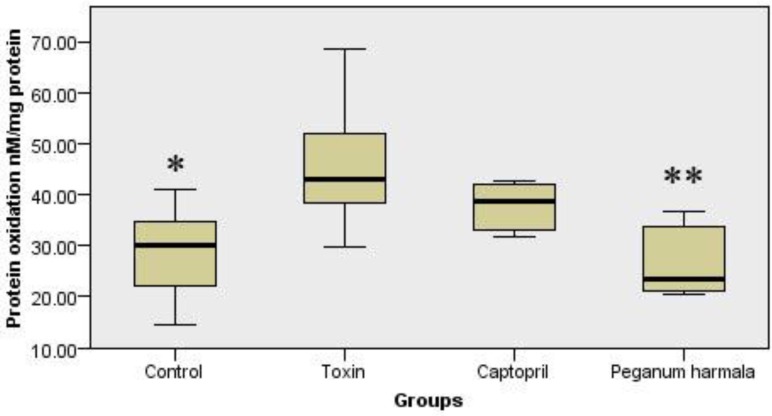
Brain ACE activity
Peganum harmala significantly inhibited ACE activity in the brain compared to toxin group (Figure 5).
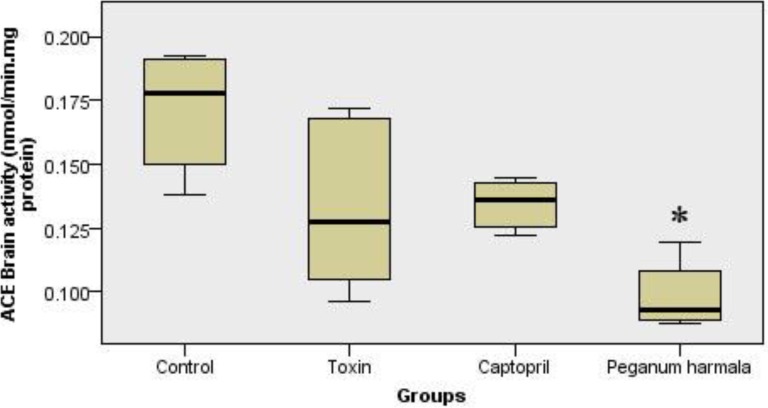
Histology examination
There were no significant differences in the number of dopaminergic neurons in left and right hemispheres in control group, but in toxin, captopril and PHS groups these diferrences were significant (Figure 6 and Table 1).
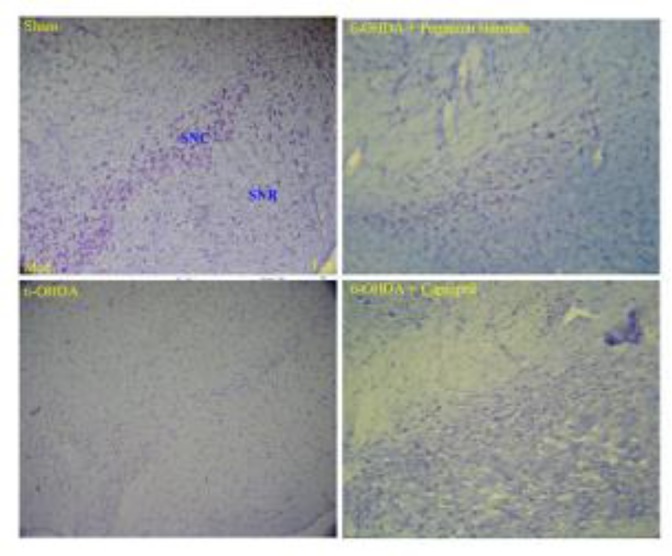
Total number of Nissl-stained neurons of SN on the left and right hemisphere.
Discussion
We studied the protective effect of AEPHS on male rats with Parkinson's disease induced by 6-OHDA. Data showed AEPHS (10 mg/kg) improved movement criteria in diseased rats by lowering rigidity and apomorphine induced rotation. Oxidative stress markers such as lipid peroxidation and protein oxidation in the brain of Peganum group were significantly lower than toxin group. Brain ACE activity in the Peganum was significantly lower than toxin and control groups. In histology study, AEPHS group had more vital dopaminergic neurons compared to toxin group. These results were compared to captopril and showed that the crude extract of Peganum was more potent than pure captopril in reducing PD signs and oxidative stress markers.
Many studies have shown that free radicals are destructive chemical substrates in PD (20-22). Imbalance between oxidant and antioxidant system can induce destructive effects of free radicals. Increasing in lipid peroxidation and decreasing antioxidants can induce PD (23). 6-OHDA is a catecholaminergic neurotoxin that is widely used as a laboratory chemical in PD model studies. Many data show that 6-OHDA has a close relationship with free radicals, because malondialdehyde increases in the presence of 6-OHDA (5, 24).
Captopril can reduce oxidative stress by 6-OHDA significantly and it is suggested that this ACE inhibitor can reduce dopaminergic neurodegeneration and progression of disease (12, 25). ACE inhibitors are efficient by scavenging ROS (26). Although some studies suggest that ACE inhibitors with "SH" group (like captopril) scavenge ROS, other studies show that this capacity is unrelated to "SH" group, and ACE inhibitors without "SH" group have the same antioxidant power (26, 27). This effect of ACE inhibitors may relate to prevention of angiotensin П synthesis (28). Angiotensin II induces oxidative stress in the brain by NADPH (12, 29). NADPH oxidase has distribution in brain (30, 31). Nontoxic doses of some neurotoxins can help to destruction of dopaminergic neurons related to NADPH, and production of ROS (23).
Brain angiotensin can promote dopaminergic degeneration and PD (28, 32), and blocking of this system could improve PD (11-13, 26, 33-36).
Peganum harmala L. is a full source of β carboline alkaloids. Some of its important alkaloids are harmine, harmaline, and harmalol (37). Harmaline inhibits ACE comparable to captopril (14). In a study of 135 herbal medicines for their ACE inhibitory effect, Peganum harmala showed a complete inhibition (15). β carboline alkaloids are benzodiazepine antagonists and inhibitors of amine oxidases, too (38).
We showed that Peganum harmala extract had antioxidant and ACE inhibitory effect. Peganum harmala decreased lipid peroxidation and protein oxidation in the brain of rats with 6-OHDA induced PD, and increased vital neurons in SN, which improved PD symptoms.
Conclusion
These findings demonstrate that peganum harmala L. has protective effect on 6-OHDA induced hemi-Parkinsonism rats, which might be through ACE inhibition.
Acknowledgements
References
-
1.
Greenamyre JT, Hastings TG. Biomedicine Parkinson's--divergent causes, convergent mechanisms. Science. 2004;304:1120-2. [PubMed ID: 15155938].
-
2.
Dauer W, Przedborski S. Parkinson's disease: mechanisms and models. Neuron. 2003;39:889-909. [PubMed ID: 12971891].
-
3.
Jenner P. Oxidative stress in Parkinson's disease. Ann Neurol. 2003;53(Suppl 3):S26-36. discussion S36-8. [PubMed ID: 12666096].
-
4.
Mariani E, Polidori MC, Cherubini A, Mecocci P. Oxidative stress in brain aging, neurodegenerative and vascular diseases: an overview. J Chromatogr B Analyt Technol Biomed Life Sci. 2005;827:65-75.
-
5.
Soto-Otero R, Mendez-Alvarez E, Hermida-Ameijeiras A, Lopez-Real AM, Labandeira-Garcia JL. Effects of (-)-nicotine and (-)-cotinine on 6-hydroxydopamine-induced oxidative stress and neurotoxicity: relevance for Parkinson's disease. Biochem Pharmacol. 2002;64:125-35. [PubMed ID: 12106613].
-
6.
Dikalov S. Cross talk between mitochondria and NADPH oxidases. Free Radic Biol Med. 2011;51:1289-301. [PubMed ID: 21777669].
-
7.
Arregui A, Barer GR. Chronic hypoxia in rats: alterations of striato-nigral angiotensin converting enzyme, GABA, and glutamic acid decarboxylase. J Neurochem. 1980;34:740-3. [PubMed ID: 6243699].
-
8.
Joglar B, Rodriguez-Pallares J, Rodriguez-Perez AI, Rey P, Guerra MJ, Labandeira-Garcia JL. The inflammatory response in the MPTP model of Parkinson's disease is mediated by brain angiotensin: relevance to progression of the disease. J Neurochem. 2009;109:656-69. [PubMed ID: 19245663].
-
9.
Rodriguez-Perez AI, Valenzuela R, Villar-Cheda B, Guerra MJ, Lanciego JL, Labandeira-Garcia JL. Estrogen and angiotensin interaction in the substantia nigra Relevance to postmenopausal Parkinson's disease. Exp Neurol. 2010;224:517-26. [PubMed ID: 20580712].
-
10.
Mendelsohn FA, Jenkins TA, Berkovic SF. Effects of angiotensin II on dopamine and serotonin turnover in the striatum of conscious rats. Brain Res. 1993;613:221-9. [PubMed ID: 7514480].
-
11.
Kurosaki R, Muramatsu Y, Kato H, Watanabe Y, Imai Y, Itoyama Y, Araki T. Effect of angiotensin-converting enzyme inhibitor perindopril on interneurons in MPTP-treated mice. Eur Neuropsychopharmacol. 2005;15:57-67. [PubMed ID: 15572274].
-
12.
Lopez-Real A, Rey P, Soto-Otero R, Mendez-Alvarez E, Labandeira-Garcia JL. Angiotensin-converting enzyme inhibition reduces oxidative stress and protects dopaminergic neurons in a 6-hydroxydopamine rat model of Parkinsonism. J Neurosci Res. 2005;15:865-873. [PubMed ID: 16015598].
-
13.
Moradganjeh A, Ziai SA, Roghani M. Losartan pretreatment reduces neurodegeneration and behavioural symptoms in 6-hydroxydopamine induced unilateral rat model of Parkinson's disease. Pathophysiology. 2013;20:243-48.
-
14.
Hivrale VK, Lomate PR. Angiotensin-Converting Enzyme Inhibitory Potential of Harmaline Isolated from Peganum Harmala L Seeds. Journal of Herbs, Spices & Medicinal Plants. 2013;19:48-53.
-
15.
Ziaei S, Dastpak A, Poorhoseini L, Rezazadeh SA, Taghizadeh M, Shabestari A, Naghdibadi H. Study of the ACE inhibitory effect of medicinal plants used in Iranian folk-medicine as antihypertensive remedy. Journal of Medical Plants. 2006.
-
16.
He Y, Lee T, Leong SK. Effect of 6-OHDA injection on the AMPA glutamate receptor subunits in the substantia nigra of Sprague-Dawley rats. Neurosci Lett. 1998;241:1-4. [PubMed ID: 9502201].
-
17.
Morpurgo C. Effects of antiparkinson drugs on a phenothiazine-induced catatonic reaction. Arch Int Pharmacodyn Ther. 1962;137:84-90. [PubMed ID: 14476206].
-
18.
Kruger NJ. The Bradford method for protein quantitation. Methods Mol Biol. 1994;32:9-15. [PubMed ID: 7951753].
-
19.
Hermida-Ameijeiras A, Mendez-Alvarez E, Sanchez-Iglesias S, Sanmartin-Suarez C, Soto-Otero R. Autoxidation and MAO-mediated metabolism of dopamine as a potential cause of oxidative stress: role of ferrous and ferric ions. Neurochem Int. 2004;45:103-16. [PubMed ID: 15082228].
-
20.
Dias V, Junn E, Mouradian MM. The role of oxidative stress in Parkinson's disease. J Parkinsons Dis. 2013;3:461-91. [PubMed ID: 24252804].
-
21.
Hwang O. Role of oxidative stress in Parkinson's disease. Exp Neurobiol. 2013;22:11-7. [PubMed ID: 23585717].
-
22.
Zuo L, Motherwell MS. The impact of reactive oxygen species and genetic mitochondrial mutations in Parkinson's disease. Gene. 2013;532:18-23. [PubMed ID: 23954870].
-
23.
Gao HM, Liu B, Zhang W, Hong JS. Synergistic dopaminergic neurotoxicity of MPTP and inflammogen lipopolysaccharide: relevance to the etiology of Parkinson's disease. FASEB J. 2003;17:1957-9. [PubMed ID: 12923073].
-
24.
Monteiro HP, Winterbourn CC. 6-Hydroxydopamine releases iron from ferritin and promotes ferritin-dependent lipid peroxidation. Biochem Pharmacol. 1989;38:4177-82. [PubMed ID: 2512934].
-
25.
Mak IT, Freedman AM, Dickens BF, Weglicki WB. Protective effects of sulfhydryl-containing angiotensin converting enzyme inhibitors against free radical injury in endothelial cells. Biochem Pharmacol. 1990;40:2169-75. [PubMed ID: 2173602].
-
26.
Ravati A, Junker V, Kouklei M, Ahlemeyer B, Culmsee C, Krieglstein J. Enalapril and moexipril protect from free radical-induced neuronal damage in vitro and reduce ischemic brain injury in mice and rats. Eur J Pharmacol. 1999;373:21-33. [PubMed ID: 10408248].
-
27.
Benzie IF, Tomlinson B. Antioxidant power of angiotensin-converting enzyme inhibitors in vitro. Br J Clin Pharmacol. 1998;45:168-9. [PubMed ID: 9491832].
-
28.
Mertens B, Vanderheyden P, Michotte Y, Sarre S. The role of the central renin-angiotensin system in Parkinson's disease. J Renin Angiotensin Aldosterone Syst. 2010;11:49-56. [PubMed ID: 19861346].
-
29.
Rodriguez-Pallares J, Rey P, Parga JA, Munoz A, Guerra MJ, Labandeira-Garcia JL. Brain angiotensin enhances dopaminergic cell death via microglial activation and NADPH-derived ROS. Neurobiol Dis. 2008;31:58-73. [PubMed ID: 18499466].
-
30.
Infanger DW, Sharma RV, Davisson RL. NADPH oxidases of the brain: distribution, regulation, and function. Antioxid Redox Signal. 2006;8:1583-96. [PubMed ID: 16987013].
-
31.
Serrano F, Kolluri NS, Wientjes FB, Card JP, Klann E. NADPH oxidase immunoreactivity in the mouse brain. Brain Res. 2003;988:193-8. [PubMed ID: 14519542].
-
32.
Labandeira-Garcia JL, Rodriguez-Pallares J, Rodriguez-Perez AI, Garrido-Gil P, Villar-Cheda B, Valenzuela R, Guerra MJ. Brain angiotensin and dopaminergic degeneration: relevance to Parkinson's disease. Am J Neurodegener Dis. 2012;1:226-44. [PubMed ID: 23383395].
-
33.
Grammatopoulos TN, Jones SM, Ahmadi FA, Hoover BR, Snell LD, Skoch J, Jhaveri VV, Poczobutt AM, Weyhenmeyer JA, Zawada WM. Angiotensin type 1 receptor antagonist losartan, reduces MPTP-induced degeneration of dopaminergic neurons in substantia nigra. Mol Neurodegener. 2007;2:1. [PubMed ID: 17224059].
-
34.
Jenkins TA, Wong JY, Howells DW, Mendelsohn FA, Chai SY. Effect of chronic angiotensin-converting enzyme inhibition on striatal dopamine content in the MPTP-treated mouse. J Neurochem. 1999;73:214-9. [PubMed ID: 10386973].
-
35.
Reardon KA, Mendelsohn FA, Chai SY, Horne MK. The angiotensin converting enzyme (ACE) inhibitor, perindopril, modifies the clinical features of Parkinson's disease. Aust N Z J Med. 2000;30:48-53. [PubMed ID: 10800878].
-
36.
Sonsalla PK, Coleman C, Wong LY, Harris SL, Richardson JR, Gadad BS, Li W, German DC. The angiotensin converting enzyme inhibitor captopril protects nigrostriatal dopamine neurons in animal models of parkinsonism. Exp Neurol. 2013;250:376-83. [PubMed ID: 24184050].
-
37.
Glasby JS. Encyclopedia of the Alkaloids. NewYork: Springer; 1977. p. 225-6.
-
38.
Herraiz T, Chaparro C. Human monoamine oxidase enzyme inhibition by coffee and beta-carbolines norharman and harman isolated from coffee. Life Sci. 2006;78:795-802. [PubMed ID: 16139309].