Abstract
Keywords
Quinolines COX-2 inhibitory Aromatase inhibitory Breast cancer
Introduction
Breast cancer is one of the leading causes of cancer-related mortality among women worldwide (1). In most of cases, it is hormone-dependent because tumor progression is dependent on high levels of circulating estrogens, which help the cancer cells to proliferate. Moreover, in postmenopausal women, biologically active estrogens are locally produced from circulating inactive steroids in an intracrine mechanism in breast cancer tissues and confer estrogenic activities to carcinoma cells (2) A series of enzymes are involved in this intratumoral or in situ production of estrogens in breast carcinoma tissues, aromatase, a member of the cytochrome P450 family, is the key enzyme in this process, promoting the aromatization of theAring of androgen precursors (3) the other enzyme involved in breast cancer are COX-2.In addition to the role of COX-2 in inflammatory disorders such as rheumatoid arthritis and osteoarthritis, it is also implicated in cancer and angiogenesis. In this regard, several epidemiologic studies have been reported that inhibitors of COX-2 enzyme reduce the risk of colorectal, breast, and lung cancer, and COX-2 is expressed in these cancers(4-6). So we decided to design quinoline derivatives as dual inhibitors of COX-2 and aromatase to find new approaches for the prevention and treatment of breast cancer.
We recently reported several investigations describing the design, synthesis, and a molecular modeling study for a group of 2-phenyl-4-carboxyl quinolones (Figure 1, A) possessing a methyl sulfonyl COX-2 pharmacophore at the para position C-2 phenyl ring (7, 8).Our results showed that quinoline ring is a suitable scaffold for COX-2 inhibitory activity. On the other hand, the structure of nonsteroidal aromatase inhibitors can be considered to consist of two parts. One part is the azole part with a nitrogen atom which interacts with the heme iron atom of the cytochrome P450 of aromatase. The second part is the bulky aryl part, which mimics the steroid ring of the substrate (andrestendione) (Figure1, B)(8, 9).
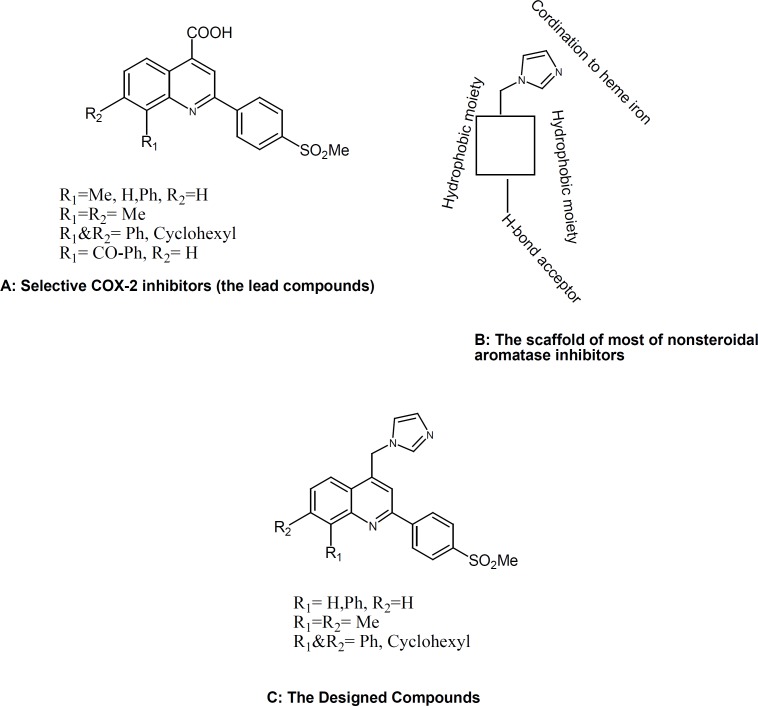
So we changed the carboxyl group of our selective COX-2 inhibitors (possessing a methyl sulfonyl COX-2 pharmacophore at the para position C-2 phenyl ring) with the imidazolering, the main pharmacophore for anti-aromatase activityn(9) and as shown in Fig. 1, our designed compounds (C)are possessing bulky aryl part, which are important for inhibiting both aromatase and COX-2 enzymes.
Experimental
Chemistry
All chemicals and solvents used in this study were purchased from Merck AG and Aldrich Chemical. Melting points were determined with a Thomas-Hoover capillary apparatus. Infrared spectra were acquired using a Perkin Elmer Model 1420 spectrometer. A Bruker FT-500 MHz instrument (Brucker Biosciences, USA) was used to acquire 1H NMR spectra with TMS as internal standard. Chloroform-D and DMSO-d6 were used as solvents. Coupling constant (J) values are estimated in hertz (Hz) and spin multiples are given as s (singlet), d (double), t (triplet), q (quartet), m (multiplet), and br (broad). The mass spectral measurements were performed on an 6410Agilent LCMS triple quadrupole mass spectrometer(LCMS) with an electrospray ionization (ESI) interface. Microanalyses, determined for C and H, were within ± 0.4% of theoretical values.
General procedure for preparation of 2-(4-(methylthio)phenyl)-7,8-substituted-quinoline-4-carboxylic acid (4a-4e)
These compounds were synthesized according to our pervious methods(7, 11).
General procedure for preparation of 2-(4-methylthio-phenyl)-7,8-substituted-quinoline-4-yl)methanol (7a-7e)
LiAlH4 (0.45 g, 12 mmol) was suspended in dry THF (20 ml) under a nitrogen atmosphere. Under vigorous stirring a solution of appropriate acid(4a-4e) (5.67 mmol) in dry THF was added dropwise to keep the reaction mixture slightly boiling. After stirring for 2 h at room temperature, the suspension was carefully hydrolyzed with NaOH solution (10%) till no more hydrogen was produced. The solid was filtered off and washed thoroughly with chloroform(12). The filtrate was dried over Na2SO4 and the solvent was removed under reduced pressure to yield a yellow oil which was crystallized from ether/hexane (25:75 v/v) (yield: 62-79 %).
2-(4-Methylthio-phenyl)-8-phenylquinoline-4-yl)methanol (7a)
Yield: 77%; yellow crystalline powder; mp=131-133˚C; IR (KBr): ν (cm-1), 3745-2371(OH);LCMS (ESI): 380.6 (M+23) +100.
2-(4-Methylthio-phenyl)-7,8-dimethylquinoline-4-yl)methanol (7b)
Yield: 62%; pale yellow crystalline powder; mp=140-141˚C; IR (KBr): ν (cm-1), 3406(OH);
LCMS (ESI): 310.2 (M+1) +100.
2-(4-Methylthio-phenyl)-benzo[h]quinoline-4-yl)methanol (7c)
Yield: 68%; yellow crystalline powder; mp=111-113˚C; IR (KBr): ν (cm-1), 3290(OH);
LCMS (ESI): 332.9 (M+1) +100.
7,8,9,10-Tetrahydro-2-(4-methylthio-phenyl)-benzo[h]quinoline-4-yl)methanol (7d)
Yield: 79%; pale yellow crystalline powder; mp=139-140ºC; IR (KBr): ν (cm-1), 3234(OH);
LCMS (ESI): 336.9 (M+1) +100.
2-(4-Methylthio)phenyl- quinoline-4-yl)methanol (7e)
Yield: 63%; yellow crystalline powder; mp=120-122ºC; IR (KBr): ν (cm-1), 3224(OH);
LCMS (ESI): 282.1 (M+1) +100.
General procedure for preparation of 2-(4-methylsulfonyl-phenyl)-7,8-substituted-quinoline-4-yl)methanol (8a-8e)
One gram of 2-(4-methylthio)phenyl-7,8-substituted-quinoline-4-yl)methanol (7a-7e)was dissolved in 10 ml THF and 5 g oxone in THF/water was added. The mixture was stirred at room temperature for 3-5 h, after evaporation of THF, the residue was extracted with chloroform and dried with sodium sulfate and then evaporated(13), the product was recrystallized in chloroform/hexane (yields: 67-70%).
2-(4-Methylsulfonyl-phenyl)-8-phenylquinoline-4-yl)methanol (8a)
Yield: 48%; yellow crystalline powder; mp=178-179ºC; IR (KBr): ν (cm-1), 3442(OH) 1312, 1157(SO2);LCMS (ESI): 390.8 (M+1) +100.
2-(4-Methylsulfonyl-phenyl)-7,8-dimethylquinoline-4-yl)methanol (8b)
Yield42%; orange crystalline powder; mp=148-150ºC; IR (KBr): ν (cm-1), 3241(OH), 1303, 1149(SO2);LCMS (ESI): 342.1(M+1) +100.
2-(4-Methylsulfonyl-phenyl)-benzo[h]quinoline-4-yl)methanol (8c)
Yield: 65%; yellow crystalline powder; mp=210-211ºC; IR (KBr): ν (cm-1), 3485 (OH), 1296, 1145(SO2);1HNMR (DMSO-d6 ): δ(ppm) 3.28(s, 3H, SO2Me), 5.18 (s, 2H, CH2), 5.71 (s, 1H, OH), 7.74-7.80 (m, 2H, benzoquinoline H8&H9), 7.96-7.80(m, 2H,benzoquinoline H6&H5), 7.99 (d, 1H,benzoquinoline H7) 8.11 (d, 2H, 4-methylsulfonylphenyl H2&H6, J=8.46Hz), 8.37 (s, 1H,benzoquinoline H3), 8.63 (d, 2H, 4-methylsulfonylphenyl H3&H5, J=8.46Hz), 9.4 (d, 1H, quinoline H10, J=7.34Hz); LCMS(ESI): 364.1 (M+1)+100.
7,8,9,10-Tetrahydro-2-(4-methylsulfonyl-phenyl)-benzo[h]quinoline-4-yl)methanol (8d)
Yield: 57%; yellow crystalline powder; mp=162-163ºC; IR (KBr): ν (cm-1), 3365 (OH), 1309, 1159(SO2);LCMS (ESI): 368.9 (M+1) +100.
2-(4-Methylsulfonyl)phenyl-quinoline-4-yl)methanol (8e)
Yield: 75%; yellow crystalline powder; mp=164-166ºC IR (KBr): ν (cm-1), 3340(OH), 1301, 1151(SO2);LCMS (ESI): 314.9 (M+1) +100.
General procedure for preparation of 4-((1H-imidazol-1-yl)methyl)-2-(4-methylsulfonyl-phenyl)-7,8-substituted-quinoline
To a solution of the corresponding alcohol (1.59 mmol) in 15 ml NMP (N-methyl-2-pyrrolidone), CDI (carbonyl 1, 1-diimidazole) (1.29 g, 7.9 mmol) was added. Then the solution was heated to reflux for 20 h at 170 ºC. After cooling to ambient temperature, it was diluted with water (50 ml) and extracted with ethyl acetate. The combined organic phases was washed with brine and water, dried over Na2SO4, and evaporated under reduced pressure (14). Then the desired product was purified by flash chromatography on silica gel (dichloromethane/methanol, 90:10 v/v), (yield: 13-62%).
4-((1H-Imidazol-1-yl)methyl)-2-(4-methylsulfonyl phenyl)-8-phenyl-quinoline (9a)
Yield: 25%; cream crystalline powder; mp=196-198ºC;IR (KBr): ν (cm-1), 1310, 1150 (SO2);1HNMR (CDCl3 ):δ(ppm) 3.03 (s, 3H, SO2Me) 5.73 (s, 2H, CH2), 7.04 (s, 1H, imidazole H5), 7.23(s, 1H, imidazole H2), 7.34(s, 1H, imidazole H4), 7.45 (t, 1H, phenyl H4, J=7.15Hz), 7.52 (t, 2H, phenyl H3&H5, J=7.31Hz), 7.69-7.76(m, 4H, quinoline H6&H7&phenyl H2 &H6), 7.86 (d, 1H, quinoline H5, J=6.88 Hz ),7.92 (s, 1H, quinoline H3), 7.95 (d, 2H, 4-methoxysulfonylphenyl H2&H6, J=8.13Hz), 8.14 (d, 2H, 4-methoxysulfonylphenyl H3&H5, J=8.13Hz); LCMS(ESI): 440.6 (M+1)+100.
4-((1H-Imidazol-1-yl)methyl)-2-(4- methylsulfonyl phenyl)-7,8-dimethyl-quinoline (9b)
Yield: 61%; yellow crystalline powder; mp=218-219ºC; IR (KBr): ν (cm-1), 1301, 1150 (SO2); 1HNMR (CDCl3 ): δ(ppm) 2.58(s, 3H, Me), 2.91(s, 3H, Me), 3.12(s, 3H, SO2Me), 5.76 (s, 2H, CH2), 7.05 (s, 1H, imidazole H5), 7.26(s, 1H, imidazole H4), 7.39(s, 1H, imidazole H2), 7.50 (d, 1H, quinoline H6, J=8.53Hz), 7.73 (d, 1H, quinoline H5,J=8.53Hz), 8.01 (s, 1H, quinoline H3), 8.08 (d, 2H, 4-methylsulfonylphenyl H2&H6, J=8.53Hz), 8.26 (d, 2H, 4-methylsulfonylphenyl H3&H5, J=8.53Hz); LCMS(ESI): 392.1 (M+1)+100.
4-((1H-Imidazol-1-yl)methyl)-2-(4- methylsulfonyl phenyl)-benzo[h]-quinoline (9c)
Yield: 25%; cream crystalline powder; mp=216-217ºC; IR (KBr): ν (cm-1) 1303, 1148 (SO2);1HNMR (DMSO-d6 ): δ(ppm) 3.26(s, 3H, SO2Me), 5.90 (s, 2H, CH2 ), 7.01 (s, 1H, imidazole H5), 7.36(s, 1H, imidazole H2), 7.79 (m, 2H, benzoquinoline H8&H9), 7.93(s, 1H, imidazole H4), 8.03-8.05(m, 3H,benzoquinoline H3&H6&H7), 8.10 (d, 2H, 4-methylsulfonylphenyl H2&H6, J=8.32Hz), 8.14 (d, 1H,benzoquinoline H10), 8.50 (d, 2H, 4-methylsulfonylphenyl H3&H5, J=8.32Hz), 9.4 (d, 1H, quinoline H5); LCMS(ESI): 414.6 (M+1)+100.
4-((1H-Imidazol-1-yl)methyl)-7,8,9,10-tetrahydro-2-(4-methylsulfonylphenyl)-benzo[h]-quinoline (9d)
Yield: 35%; cream crystalline powder; mp=247-248ºC; IR (KBr): ν (cm-1) 1318, 1163(SO2);1HNMR (CDCl3 ): δ(ppm) 1.9-2.01(m, 4H, CH2), 2.96 (m, 2H, CH2), 3.08 (s, 3H, SO2Me), 3.46 (m, 2H, CH2),5.67 (s, 2H, CH2 ), 7.0 (s, 1H, imidazole H5 ), 7.2(s, 1H, imidazole H2 ), 7.29 (s, 1H, imidazole H4 ), 7.35 (d, 1H, quinoline H6 J=8.57 Hz), 7.66 (d, 1H, quinoline H5, J=8.58 Hz), 7.70 (s, 1H, quinoline H3), 8.03 (d, 2H, 4-methoxysulfonylphenyl H2&H6, J=8.46Hz), 8.29 (d, 2H, 4-methoxysulfonylphenyl H3&H5, J=8.46Hz); LCMS(ESI): 418.7 (M+1)+100.
4-((1H-Imidazol-1-yl)methyl)-2-(4- methylsulfonyl phenyl)-quinoline (9e)
Yield: 15%; cream crystalline powder; mp=189-190ºC; IR (KBr): ν (cm-1) 1300, 1150(SO2);1HNMR (CDCl3 ): δ (ppm) 3.1(s, 3H, SO2Me), 5.76 (s, 2H, CH2), 7.07 (s, 1H, imidazole H5), 7.26(s, 1H, imidazole H4), 7.29(s, 1H, imidazole H2), 7.69-7.72 (m, 2H, quinoline H6&H3), 7.87 (t, 1H, quinoline H7, J=7.27Hz), 7.98 (d, 1H, quinoline H5, J=8.28Hz), 8.09 (d, 2H, 4-methylsulfonylphenyl H2&H6, J=8.37Hz), 8.26 (d, 2H, 4-methylsulfonylphenyl H3&H5, J=8.37Hz), 8.3 (d, 1H, quinoline H8, J=8.40Hz); LCMS(ESI): 364.8 (M+1)+100.
Molecular modeling (docking) studies
Docking studies were performed using Autodock software Version 3.0. The coordinates of the X-ray crystal structure of the selective COX-2 inhibitor SC-558 bound to the murine COX-2 enzyme was obtained from the RCSB Protein Data Bank (1cx2) and hydrogens were added. The ligand molecules were constructed using the Builder module and were energy minimized for 1000 iterations reaching a convergence of 0.01 kcal/mol.The energy minimized ligands were superimposed on SC-558 in the PDB file 1cx2 after which SC-558 was deleted. The purpose of docking is to search for favorable binding configuration between the small flexible ligands and the rigid protein. Protein residues with atoms greater than 7.5 A from the docking box were removed for efficiency. These docked structures were very similar to the minimized structures obtained initially. The quality of the docked structures was evaluated by measuring the intermolecular energy of the ligand–enzyme assembly (15, 16).
In-vitro cyclooxygenase (COX) inhibition assays
The assay was performed using an enzyme chemiluminescentkit (Cayman Chemical, MI, USA) according to our previously reportedmethod(17)The Cayman chemical chemiluminescent COX
(ovine) inhibitor screening assay utilizes the heme-catalyzedhydroperoxidase activity of ovine cyclooxygenases to generateluminescence in the presence of a cyclic naphthalene hydrazideand the substrate arachidonic acid. Arachidonate-induced luminescencewas shown to be an index of real-time catalytic activity anddemonstrated the turnover inactivation of the enzyme. Inhibition
of COX activity, measured by luminescence, by a variety of selectiveand non-selective nhibitors showed potencies similar to those observedwith other in-vitro and whole cell methods.
Cytotoxicity assay
Cell line and culture conditions
The human breast cancer T47D and MCF-7 cell lines were obtained from Pasteur Institute Cell Bank of IRAN (Tehran, IRAN). Cells were maintained in RPMI-1640(Gibco, UK) culture medium supplemented with 10% fetal bovine serum (Gibco, UK) and 100 U Ml-1 of penicillin and 100 ng Ml-1 of streptomycin (Gibco, UK) at 37 C in 5% CO2 incubator. All reagent and chemicals used in this experiment were of cell culture or molecular biology grade, purchased from different international sources.
General procedure
The MTT (3-[4, 5-dimethylthiazol-2-yl]-2,5-diphenyl tetrazolium bromide) based assay was performed by seeding 5000 cells (T47D and MCF-7) per 180 µL RPMI complete culture medium in each well of 96-well culture plates. The day after seeding, culture medium was changed with medium containing standard anti-tumor drug Doxorubicin as well as different concentrations of newly synthesized compounds and RPMI control (no drug). Cells were then incubated at 37ºC in 5% Co2 incubator for 48h and 72h. Then 25 µL of MTT solution (4mg Ml-1) were added to each well and further incubated at 37ºC for 3h. At the end of incubation, formazan crystals were dissolved in 100 µL of DMSO and plates were read in a plate reader (TECAN, Austria) at 540 nm. This experiment was performed in triplicate determination each time (18,19).
Result and discussion
The synthesis of target compounds was carried out according to Scheme 1. All the synthesized compounds were assayed for their COX-2 inhibitoryandalso cytotoxic activities against breast cancer cell lines (MCF7 and T47D). The results are presented in Table 2.
SAR data (IC50 values) acquired by determination of the in-vitro ability of the title compoundsto inhibit the COX-1 and COX-2 isozymes showed thatthe COX inhibition was sensitive to the lipophilic nature of substituents.As shown in Table 1, our results showed that the increase oflipophilic properties of substituents on the C-7 and C-8 quinoline ring increased COX-2 inhibitory potency and selectivity. The relative COX-2 potency, and COX-2 selectivity profiles for the 4-imidazolylmethylquinoline derivatives, with respect to the C-7 and C-8 substituents was9d > 9c > 9a > 9b >9e. However, among the 4-imidazolylmethylquinoline derivatives,compound 9d possessing an unsaturated cyclohexyl ring attachedto C-7 and C-8 quinoline ring exhibited highest COX-2 inhibitorypotency and selectivity (COX-2 IC50 = 0.063 µM; SI =547.6) thatwasas potent as the reference drug celecoxib and more selective COX-2 inhibitor than celecoxib (COX-2IC50 = 0.060 µM; SI = 405).
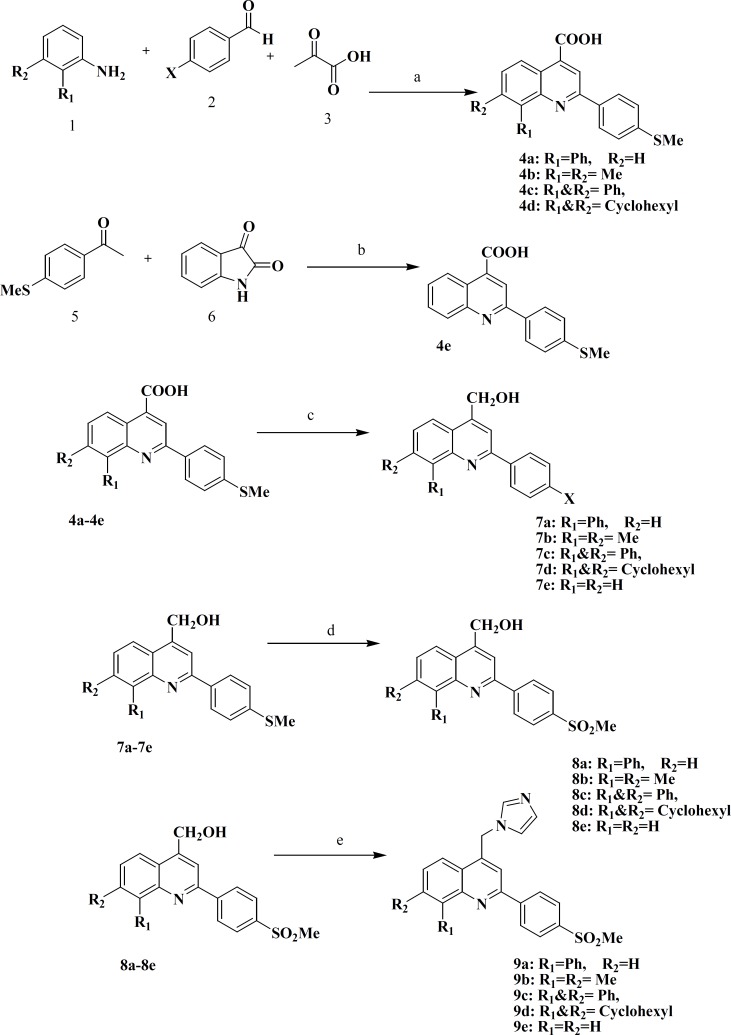
In-vitro COX-1 and COX-2 enzyme inhibition assay data for 4-(Imidazolylmethyl)-2-(4- methylsulfony phenyl)-Quinolinederivatives(9a-9e).
In-vitro cytotoxicity of quinolines (9a-9e).
SAR data (IC50 values)also showed that the COX inhibition was sensitive to the nature of substituents on the C-4 quinoline ring. All of the 4-imidazolylmethylquinoline derivatives were less potent but more selective COX-2 inhibitors than their corresponding 4-carboxyl derivatives. Our molecular modeling studies showed that the carboxyl group can interact with Arg120 in COX-2, so replacement of carboxyl group withimidazolylmethyl may decrease COX-2 inhibitory activity. In addition, carboxyl group can also interact with Arg120 as a key amino acid in COX-1 enzyme, so 4-imidazolylmethylquinoline derivatives have less affinity to bind to COX-1 than the 4-carboxyl derivatives and as a consequence are more selective COX-2 inhibitors.
The binding interactions of the three most potent and selective COX-2 inhibitor compound (9a, 9c and 9d) within the COX-2 binding site were investigated. They all were docked well in the COX-2 binding site. The most stable enzyme-ligand complex of (9a, 9c and 9d)whichpossessing a MeSO2 COX-2 pharmacophore at para position of C-2 phenyl ring within the COX-2 binding site (Figure 2) shows that the p-MeSO2-phenyl moiety is oriented towards the COX-2 secondary pocket (Val523, Phe518 and Arg513). These observations together with experimental results provide a good explanation for design of potent and selective COX-2 inhibitors possessing 4-((1H-imidazol-1-yl)methyl)-2-(4-methylsulfonylphenyl)quinoline framework.
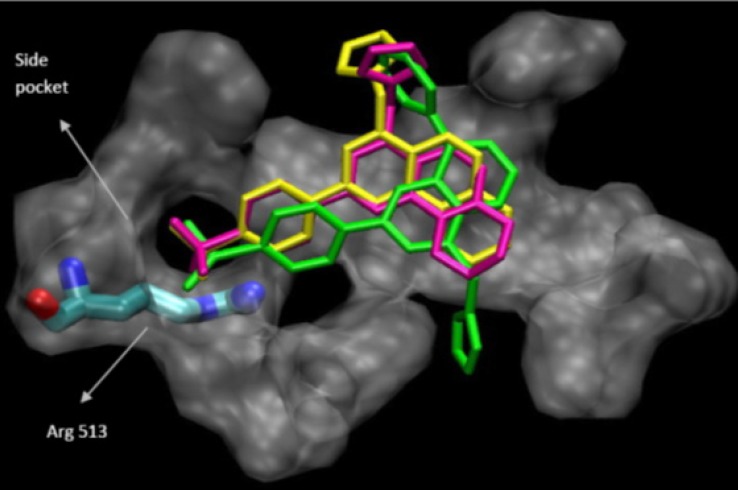
The cytotoxicity of quinolines9a-e against human breast cancer MCF-7 and T47D cells by MTT assayafter 2 and 3 days of exposure was also evaluated. After some initial evaluations concentrations of 10 and 25 μM of quinolines were used for evaluation and comparison of cytotoxicity of these compounds with doxorubicin at concentration of 250 nM against MCF-7 cells and concentrations of 25 and 50 μM of quinolines were used for evaluation and comparison of cytotoxicity of these compounds with doxorubicinat concentration of 100 nM against T47D cells. Results for each compound as the percentage of growth of the treated cells in comparison to untreated cells are shown in Table 1. The most active compound against MCF-7 cells was9d, which exhibited 4% of survival (after 2 days exposure) (IC50< 5μM). The IC50 of 9a was less than 10 μM and the IC50 of9c was 10 μM but the IC50of 9b and 9e were more than 10μMwhichwere the least potent compounds. The most potent compound against T47D cells was9a which exhibited 33.8% of survival (after 2 days exposure) (IC50 ≤ 25μM). Compounds 9c and 9e were the least potent compounds with IC50 = 50μM. The calculated IC50 values of all tested compounds after two days exposure showed that the order of the cytotoxicity against MCF-7 cells were 9d>9a >9c >9b = 9e and in T47D cells were 9a >9d>9e>9b = 9c. These data showed that the increase of lipophilic properties of substituents on the C-7 and C-8 quinoline ring increased their cytotoxicity on MCF-7. This may be due to their ability to penetrate the cell membrane or their ability to inhibit or suppress some factors and enzymes such as COX-2.As our result showed that the order of the cytotoxicity of quinolines 9a-9eagainst MCF-7 cells were the same as that of their COX-2 inhibitory so one of their cytotoxic mechanism of these compoundsmay be through their COX-2 inhibitory activity. In addition, thesequinolines9a-9e were more cytotoxic against MCF-7 cells in comparison with those of T47D. So this may be due to their different mechanism of action in these two breast cancer cellssuch as more anti-aromatase activity in MCF-7 cells in comparison with T47D cells which express aromatase mRNA less than MCF-7 cells.
Acknowledgements
References
-
1.
Cancer Facts and Figures 2009-2010. American Cancer Society: Atlanta. 2010:1-40.
-
2.
Sasano H, Miki Y, Nagasaki S, Suzuki T. In-situ estrogen production and its regulation in human breast carcinoma: From endocrinology to intracrinology. Pathol Int. 2009;59:777-789. [PubMed ID: 19883428].
-
3.
Santen RJ, Brodie H, Simpson ER, Siiteri PK, BrodieA. History of biological mediator and therapeutic target. Endocr Rev. 2009;30:343-375. [PubMed ID: 19389994].
-
4.
Hosomi Y, YokoseTY, Hirose Y, Nakajima R, Izumi N, Tada H. Increased cyclooxygenase 2 (COX-2) expression occurs frequently in precursor lesions of human adenocarcinoma of the lung. LungCancer. 2000;30:73-81.
-
5.
Oshima M, Dinchuk JE, Kargman SL, Oshima H, Hancock B, KwongE, Trzaskos JM, EvansJF, Taketo MM. Suppression of intestinal polyposis in Apc delta716 knockout mice by inhibition of cyclooxygenase 2 (COX-2). Cell. 1996;87:803-809. [PubMed ID: 8945508].
-
6.
Zarghi A andArfaei S. Selective COX-2 Inhibitors: A Review of Their Structure-Activity Relationships. Iran J Pharm Res. 2011;10:655-683. [PubMed ID: 24250402].
-
7.
Zarghi A, Ghodsi R, Azizi E, Daraie B, Hedayati M andDadrass O. Synthesis and biological evaluation of new 4-carboxyl quinoline derivatives as cyclooxygenase-2 inhibitors. Bioorg Med Chem. [PubMed ID: 19560931].
-
8.
Zarghi A andGhodsi R. Design, synthesis and biological evaluation of new Ketoprofen analogs as potent cyclooxygenase-2 inhibitors. Bioorg Med Chem. 2010;18:5855-5860. [PubMed ID: 20650641].
-
9.
Pouget C, Yahiaoui S, Fagnere C, Habriox G andChulia A. Synthesis and biological evaluation of 4- imidazolylflavans as nonsteroidal aromatase inhibitors. Bioorg Med Chem. 2004;32:494-503.
-
10.
Cavalli A andRecanatini M. Looking for selectivity among cytochrome P450inhibitors. J Med Chem. 2002;45:251-254. [PubMed ID: 11784128].
-
11.
Ghodsi R, Zarghi A, DaraieB, Hedayati M. Synthesis and biological evaluation of new 2,3-diaryl quinoline derivatives as cyclooxygenase-2 inhibitors. Bioorg Med Chem. 2010;18:1029-1033. [PubMed ID: 20061161].
-
12.
Jacobs C, Frotscher M, Dannhardt G, Hartmann RW. 1-Imidazolyl(alkyl)-substituted di- and tetrahydroquinolines and analogues: syntheses and evaluation of dual inhibitors of thromboxane A(2) synthase and aromatase. J Med Chem. 2000;43:1841-1851. [PubMed ID: 10794700].
-
13.
Zarghi A, Arfaee S, Praveen Rao PN. Design and synthesis, and biological evaluation of 1, 3- diarylprop-2-en-1-ones: A novel class of cyclooxygenase-2 Inhibitors. Bioorg Med Chem. 2006;14:2600-2605. [PubMed ID: 16356730].
-
14.
Mendieta M, Negri M, Hu Q, Hille U, Jagusch C, Jahn-Hofmann K, Muller-Viera U, Schmidt D, Lauterbach T, Hartmann RW. CYP17 inhibitors. Annulations of additional rings in methylene imidazole substituted biphenyls: synthesis, biological evaluation and molecular modeling. Arch Pharm Chem Life Sci. 2008;341:597-609.
-
15.
Morris GM, Goodsell DS, Halliday RS, Huey R, Hart WE, Belew RK, Olson AJ. Automated docking using a Lamarckian genetic algorithm and an empirical binding free energy function. J Comput Chem. 1998;19:1639-1662.
-
16.
Kurumbail RG, Stevens AM, Gierse JK, McDonald JJ, Stegeman RA, Pak JY, Gildehaus D, Miyashiro JM, Penning TD, Seibert K, Isakson PC, Stallings WC. Structural basis for selective inhibition of cyclooxygenase-2 by anti-inflammatory agents. Nature. 1996;384:644-648. [PubMed ID: 8967954].
-
17.
Zarghi A, Najafnia L, Daraee B, Dadrass OG, Hedayati M. Synthesis of 2, 3- diaryl -1, 3-thiazolidine- 4- one derivatives as selective cyclooxygenase (COX-2) inhibtors. Bioorg Med Chem Lett. 2007;17:5634-5637. [PubMed ID: 17822894].
-
18.
Zarghi A, Sabakhi I, Topuzyan V, Hajimahdi Z, Daraie B. Design, synthesis and biological evaluation of 5-oxo-1,4,5,6,7,8 hexahydroquinolinederivatives as selective cyclooxygenase-2 inhibitors. Iran J Pharm Res. 2014;13:61-69. [PubMed ID: 24711830].
-
19.
Bazargan L, FouladdelSh, Shafiee A, Amini M, Ghaffari SM andAzizi E. Evaluation of anticancer effects of newly synthesized dihydropyridine derivatives in comparison to verapamil and doxorubicin on T47D parental and resistant cell lines in-vitro. Cell Biol Toxicol. 2008;24:165-174. [PubMed ID: 17805981].