Abstract
Keywords
Introduction
Cancer is characterized by uncontrolled cell growth and division. Nowadays cancer is an important cause of mortality worldwide that affects a huge number of people during their life (1). On the basis of recent reports, approximately 17 million cancer deaths per year might occur by 2030 (2). Despite the remarkable progress in the prevention and treatment of cancer, chemotherapy methods are hindered with significant limitations (3). Therefore development of efficient and selective antitumor agents is one of the fundamental challenges of researchers working in the field of pharmaceutical sciences.
The 1,4-dihydropyridines (DHPs) are an important class of calcium channel blockers (4) and a common feature of various medicinal scaffolds such as anti-hypertensive (5), antidiabetic (6), antituberculosis (7), anti-coagulant (8), antidyslipidemic (9), antioxidant (9, 10), and multidrug resistance (MDR) reversal (11, 12) agents.
Importantly, DHPs and other calcium channel blockers have demonstrated efficacy as anticancer agents in several studies (13-15). In this regard, several reports indicating anti-tumor activity of symmetric 3,5-dicarboxamide (16), 3,5-diketo (13, 17), 3,5-dicarboxylate (17, 18) and 3,5-dicyano (19) derivatives of 1,4-DHPs have been documented in the literature. Dexniguldipine (Scheme 1) is a prototype of the asymmetrical 1,4-DHPs exhibiting therapeutic activity in canine osteosarcoma (20).
Moreover, there are some reports highlighting the effect of DHPs on potentiation of anti-tumor activity of common chemotherapeutic drugs. For instance, structural conjugation of homocamptothecins with DHP scaffold enhanced the inhibition of DNA topoisomerase I (21). It has been also shown that 3,5-dibenzoyl-4-(2-methylthiazol-4-yl)-1,4-dihydro-2,6-dimethylpyridine and 3,5-diacetyl-4-[2-(2-chlorophenyl)thiazol-4-yl)]-1,4-dihydro-2,6-dimethylpyridine exhibited lower cytotoxic effect compared to doxorubicin, however, it could significantly potentiate doxorubicin cytotoxicity in T47D and RS cancer cells (13).
Considering the available evidence on the potential antitumoral effect of DHP structures and also based on our interest in assessment of biological activities of 1,4-DHP structures (22-24), herein we report the cytotoxic activities of new 2,6-dimethyl-3,5-bis-N-(aryl/heteroaryl) carbamoyl-4-aryl-1,4-dihydropyridine and 1,4-bis(2,6-dimethyl-3,5-dialkyloxylcarbonyl-1,4- dihydropyridine-4-yl) benzene derivatives on three different human cancer cell lines.
Experimental
Materials and methods
Chemistry
All the synthesized compounds were characterized by mass spectroscopy, IR and 1H NMR. IR spectra were recorded on a Nicolet FT-IR Magna 550 spectrophotometer. 1H NMR spectra were determined by a Bruker FT-500 MHz spectrometer in chloroform-d1 or DMSO-d6. All the chemical shifts were reported as δ values (ppm) against tetramethylsilane as an internal standard. The MS spectra were recorded using a Finnigan TSQ-70 spectrometer at 70 eV. CHN/CHNS analysis was performed using CHNS-932 Leco analyzer and the results were within ± 0.4% of the theoretical values.
General procedure for the synthesis of 1,4-bis(2,6-dimethyl-3,5-dialkyloxycarbonyl-1,4-dihydropyridine-4-yl) benzenes (4a and 4b)
A mixture of ammonium acetate 1 (3 mmol), corresponding alkyl-3-oxo butanoate 2 (4 mmol) and terephthalaldehyde 3 (1 mmol) was refluxed in 20 mL iso-propyl alcohol in an oil bath for 24 h.
The progress of the reaction was monitored by TLC. On completion of the reaction, the solvent was removed to some extent under reduced pressure. The product was filtered, washed with small portions of cold methanol and then dried to afford the final product 4b. As for compound 4a, similar procedure was carried out while further purification by column chromatography and preparative TLC using ethylacetate/petroleum ether as eluent afforded the pure product. Physical characteristics data of the final compounds are summarized in Table 1.
1,4-bis(2,6-dimethyl-3,5-diisobutyloxylcarbonyl-1,4-dihydropyridine-4-yl) benzene (4a)
Pale yellow solid in 28%; m.p.: 232-234 °C; 1H NMR (CDCl3) δ (ppm): 7.09-7.28 (d, 4H, CH-aromatic), 5.59 (brs, 2H, NH-DHP), 4.99 (s, 2H, C4-H DHP), 3.81 (d, 8H, CH2), 2.35 (m, 4H, CH), 1.90 (s, 12H, CH3-DHP), 0.88 (d, 24H, CH3); IR (KBr) νmax (cm-1): 3343.5 (NH, DHP), 3098.8 (CH, aromatic), 2961.6 and 2874.6 (CH, aliphatic), 1698.2 (C=O, ester); MS m/z (%): 692 (M+, 36), 619 (34), 591 (72), 384 (100), 308 (100), 252 (21), 196 (50), 150 (20), 41 (6); Anal. Calcd. for C40H56N2O8: C, 69.34; H, 8.15; N, 4.04; Found: C, 69.45; H, 8.11; N, 4.18.
1,4-bis(2,6-dimethyl-3,5-diallyloxylcarbonyl-1,4-dihydropyridine-4-yl) benzene (4b)
Pale yellow solid in 44%; m.p.: 208-211 °C; 1H NMR (CDCl3) δ (ppm): 7.09 (d, 4H, CH-aromatic), 5.82-5.89 (m, 4H, CH-alkene), 5.59 (brs, 2H, NH-DHP), 5.11-5.16 (d, 8H, CH2-alkene), 5.02 (s, 2H, C4-H DHP), 4.55 (m, 8H, CH2), 2.33 (s, 12H, CH3-DHP); IR (KBr) νmax (cm-1): 3306.7 (NH, DHP), 3081.4 (CH, aromatic), 2923.9 and 2853.1 (CH, aliphatic), 1717.8 (C=O, ester); MS m/z (%): 628 (M+, 36), 587 (34), 543 (72), 276 (100), 236 (88), 218 (81), 191 (37), 150 (20), 41 (35); Anal. Calcd. for C36H40N2O8: C, 68.77; H, 6.41; N, 4.46; Found: C, 68.95; H, 6.31; N, 6.60.
General procedure for the synthesis of N-aryl-3-oxo butanamides
Required N-(aryl)-3-oxobutanamides were synthesized according to the modified method of Clemens via condensation of 2,2,6-trimethyl-1,3-dioxine-4-one with the appropriate arylamines (25). Detailed synthetic procedure to N-aryl-3-oxo butanamides may be found in our previous report (26).
General procedure for the synthesis of 2,6-dimethyl-3,5-bis-N-(aryl/heteroaryl) carbamoyl-4-aryl-1,4 dihydropyridines (7a-i)
Desired compounds (7a-i) were obtained via reaction of ammonium acetate 1 (2 mmol), corresponding N-arylacetoacetamides (5a-c) (2 mmol) and appropriate aldehyde (6a-d) (1 mmol) in 20 mL absolute ethanol in an oil bath within 24–48 h. Detailed synthetic procedure to compounds 7a-i may be found in our previous publication (26). Some characteristics of final products (7a-i) are summarized in Table 2.
Biological activity
Reagents and Chemicals
RPMI 1640, fetal bovine serum (FBS), trypsin and phosphate buffered saline (PBS) were purchased from Biosera (Ringmer, UK).3-(4,5-Dimethylthiazol-2-yl)-2,5 diphenyltetrazolium bromide (MTT) was obtained from Sigma (Saint Louis, MO, USA) and penicillin/streptomycin was purchased from Invitrogen (San Diego, CA, USA). Cisplatin and dimethyl sulphoxide were obtained from EBEWE Pharma (Unterach, Austria) and Merck (Darmstadt, Germany), respectively.
Cell lines
MCF-7 (human breast adenocarcinoma), LS180 (human colon adenocarcinoma), and MOLT-4 (human acute lymphoblastic leukemia) cells were obtained from the National Cell Bank of Iran, Pasteur Institute, Tehran, Iran. All cell lines were maintained in RPMI 1640 supplemented with 10% FBS, and 100 units/mL penicillin-G and 100 m g/mL streptomycin. Cells were grown in monolayer (MCF-7 and LS180) or in suspension (MOLT-4) cultures.
Cytotoxic effect
Cell viability following exposure to synthetic compounds was estimated by using the MTT reduction assay (22, 27). LS180 and MOLT-4 cells were plated in 96-well microplates at a density of 5 × 104 cells/mL (100 μL per well), while MCF-7 cells were plated at a density of 3 × 104 cells/mL. Control wells contained no drugs and blank wells contained only growth medium for background correction. After overnight incubation at 37 °C, half of the growth medium was removed and 50 μL of medium supplemented with 10, 25, 50 and 100 μM of synthetic compounds were added. Compounds were all first dissolved in DMSO and then diluted in medium so that the maximum concentration of DMSO in the wells did not exceed 0.5%. Cells were further incubated for 72 h. At the end of the incubation time, the medium was removed and MTT was added to each well at a final concentration of 0.5 mg/mL and plates were incubated for another 4 h at 37 °C. Then formazan crystals were solubilized in 200 μL DMSO. The optical density was measured at 570 nm with background correction at 655 nm using a Bio-Rad microplate reader (Model 680). The percentage of inhibition of viability compared to control wells was calculated for each concentration of the compound and IC50 values were calculated with the software CurveExpert version 1.34 for Windows. The absorbance of wells containing no cells was subtracted from sample well absorbance before calculating the percentage of inhibition. Each experiment was repeated 4 times.
Chemical structures of the aromatized 1,4-bis(2,6-dimethyl-3,5-dimethoxylcarbonyl-1,4-dihydropyridine-4-yl) benzene derivatives (IVa and IVb)
Chemical structures of the 2,6-dimethyl-3,5-bis-N-(aryl/heteroaryl) carbamoyl-4-aryl-1,4-dihydropyridine derivatives (VIIa-i
Cytotoxic activity of 2,6-dimethyl-3,5-bis-N-(aryl/heteroaryl) carbamoyl-4-aryl-1,4-dihydropyridines assessed by the MTT reduction assay
Compound No. | IC50 (µM) | ||
---|---|---|---|
MCF-7 | LS180 | MOLT-4 | |
IVa | NA | NA | NA |
IVb | NA | NA | NA |
VIIa | 28.8 ± 2.8 | 29.7 ± 4.7 | 17.4 ± 2.0 |
VIIb | 52.4 ± 5.9 | 90.6 ± 7.3 | 55.9 ± 7.9 |
VIIc | 56.1 ± 11.5 | 63.0 ± 5.1 | 32.8 ± 0.7 |
VIId | 28.5 ± 3.5 | 47.0 ± 18.4 | 18.7 ± 1.2 |
VIIe | 36.3 ± 3.1 | 71.4 ± 4.8 | 36.0 ± 0.7 |
VIIf | NA | NA | NA |
VIIg | NA | NA | NA |
VIIh | NA | NA | NA |
VIIi | NA | NA | NA |
Cisplatin | 14.8 ± 8.1 | 5.0 ± 2.0 | 3.9 ± 0.3 |
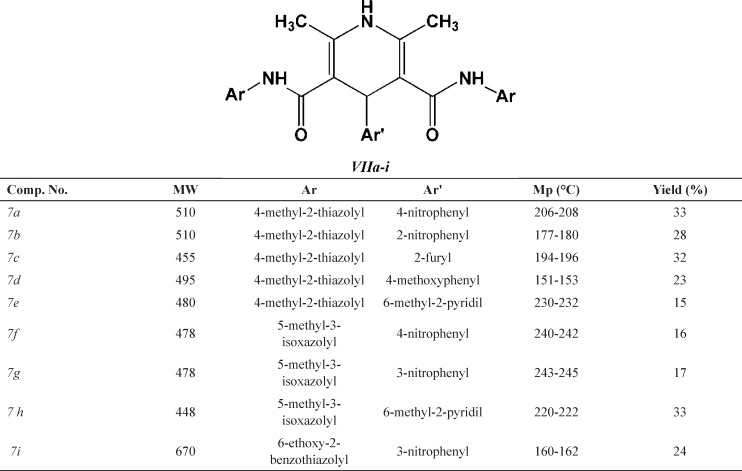
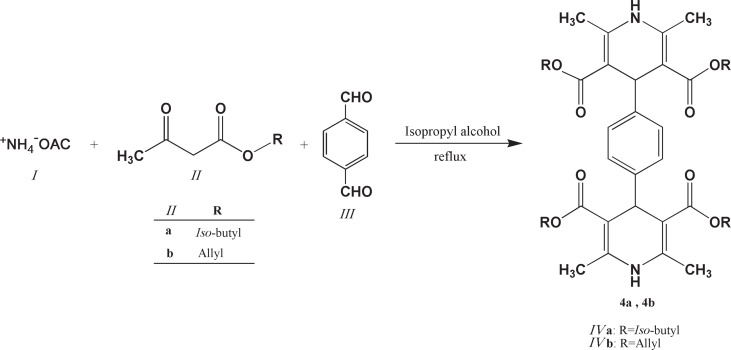
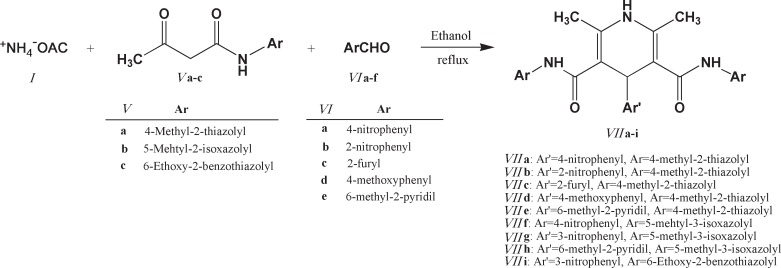
Results and Discussion
Chemistry
The structures of all synthesized 1,4-bis(2,6-dimethyl-3,5-dialkyloxylcarbonyl-1,4-dihydropyridine-4-yl) benzene and 2,6-dimethyl-3,5-bis-N-(aryl/heteroaryl) carbamoyl-4-aryl-1,4-dihydropyridines were confirmed by spectroscopic and elemental analysis methods. Schematic representation of the synthesis of compounds 4a, 4b and 7a-i are depicted in Schemes 2 and 3.
Cytotoxic activity
Synthesized1,4-bis(2,6-dimethyl-3,5-dialkyloxylcarbonyl-1,4-dihydropyridine-4-yl) benzene and 2,6-dimethyl-3,5-bis-N-(aryl/heteroaryl)carbamoyl-4-aryl-1,4-dihydropyridine derivatives were assayed for their cytotoxicity against three human cancer cell lines (MCF-7, LS180, and MOLT-4) in terms of their IC50 values (Table 3.). It should be noted that none of the evaluated compounds showed equal or superior activity compared to cisplatin.
Considering the data in Table 3, the following structure activity relationships (SAR) may be envisaged:
DHP compounds bearing 3,5-bis-N-(4-methyl-2-thiazolyl) substituents (7a-e) showed superior cytotoxic effect compared to 3,5-bis-N-(5-methyl-3-isoxazolyl) substituted DHPs (7f-g). Higher activity of thiazole containing DHPs might be attributed to the role of sulfur atoms in enhancing the lipophilicity of the molecule and thus enhancing the penetration through cell membranes.
3,5-bis-N-(6-Ethoxy-2-benzothiazolyl) substituted DHP molecule (7i) did not show cytotoxic activity against MCF-7, LS180 and MOLT-4 cancer cell lines. This activity loss might be attributed to the possible steric hindrance of this derivative at the target site.
1,4-bis(2,6-dimethyl-3,5-dialkyloxylcarbonyl-1,4-dihydropyridine-4-yl) benzenes (4a and 4b) had no cytotoxic activity against any of the tested cells. One possible explanation to this is their bulky chemical structure leading to steric clash in their site of action.
Similar trend of activity could be observed for derivatives 7a-i against the LS180 and MOLT-4 cells. Due to the structural similarities of these compounds, observed order of activity could be related to their different substitution pattern on C4 of DHP ring (4-nitrophenyl > 4-methoxyphenyl > 2-furyl > 6-methyl-2-pyridil > 2-nitrophenyl). It appeared that DHP derivatives possessing H-acceptor/donor groups on their para position of C4-substituted aromatic ring showed superior activity against LS180 and MOLT-4 cells (Table 3.).
The presence of H-acceptor/donor groups on the para position of C4-substituted aromatic ring might be more determinant in MOLT-4 cells (7a; IC50=17.4 µM and 7d; IC50=18.7 µM).
Conclusion
2,6-Dimethyl-3,5-bis-N-(aryl/heteroaryl) carbamoyl-4-aryl-1,4-dihydropyridine analogues showed weak to relatively good cytotoxic activity against MCF-7, LS180 and MOLT-4 human cancer lines, while a superior potency was observed in the case of MOLT-4 cells (7a: IC50 = 17.4±2.0 µM). It was revealed that 1,4-bis(2,6-dimethyl-3,5-dialkyloxylcarbonyl-1,4 dihydropyridine-4-yl) benzenes (4a and 4b) exhibited no cytotoxic effect on tested cancer cell lines possibly due to their bulky scaffold and hence steric hindrance in their site of action. The outcomes of this study may provide helpful information to guide the rational design and synthesis of more potent cytotoxic molecules on the basis of 1,4-DHP scaffold.
Acknowledgements
References
-
1.
Seffrin JR, Hill D, Burkart W, Magrath I, Badwe RA, Ngoma T, Mohar A, Grey N. It is time to include cancer and other noncommunicable diseases in the millennium development goals. CA Cancer J. Clin. 2009;59:282-4. [PubMed ID: 19684303].
-
2.
Thun MJ, DeLancey JO, Center MM, Jemal A, Ward EM. The global burden of cancer: priorities for prevention. Carcinogenesis. 2010;31:100-110. [PubMed ID: 19934210].
-
3.
Chabner BA, Roberts TG. Chemotherapy and the war on cancer. Nat. Rev. Cancer. 2005;5:65-72. [PubMed ID: 15630416].
-
4.
Davood A, Mansouri N, Dehpour AR, Shafaroudi H, Alipour E, Shafiee A. Design, synthesis, and calcium channel antagonist activity of new 1,4-dihydropyridines containing 4-(5)-chloro-2-ethyl-5-(4)-imidazolyl substituent. Arch. Pharm. Chem. Life Sci. 2006;339:299-304.
-
5.
Shafiee A, Rastkary N, Jorjani M, Shafaghi B. Synthesis and antihypertensive activities of new 1,4-dihydropyridine containing nitroimidazolyl substituent with a nitrooxy group at the 3- ester position. Arch. Pharm. 2002;2:69-76.
-
6.
Ogawa AK, Willoughby CA, Bergeron R, Ellsworth KP, Geissler WM, Myers RW, Yao J, Harris G, Chapman KT. Glucose-lowering in a db/db mouse model by dihydropyridine diacid glycogen phosphorylase inhibitors. Bioorg. Med. Chem. Lett. 2003;13:3405-8. [PubMed ID: 14505637].
-
7.
Iman M, Davood A, Dehqani G, Lotfinia M, Sardari S, Azerang P, Amini M. Design, synthesis and evaluation of Antitubercular activity of novel Dihydropyridine containing imidazolyl substituent. Iran. J. Pharm. Res. 2015;14:1067-75. [PubMed ID: 26664373].
-
8.
Kumar RS, Idhayadhulla A, Jamal Abdul Nassera A, Selvin J. Synthesis and anticoagulant activity of a new series of 1, 4-dihydropyridine derivatives. Eur. J. Med. Chem. 2011;46:804-810. [PubMed ID: 21220179].
-
9.
Kumar A, Maurya RA, Sharma S, Kumar M, Bhatia G. Synthesis and biological evaluation of N-aryl-1, 4-dihydropyridines as novel antidyslipidemic and antioxidant agents. Eur. J. Med. Chem. 2010;45:501-9. [PubMed ID: 19962217].
-
10.
Leon R, de los Rı´os C, Marco-Contelles J, Lo´pez MG, Garcı´a AG, Villarroya M. Synthesis of 6-amino-1, 4-dihydropyridines that prevent calcium overload and neuronal death. Eur. J. Med. Chem. 2008;43:668-674. [PubMed ID: 17692434].
-
11.
Kawase M, Shah A, Gaveriya H, Motohashi N, Sakagami H, Varga A, Molnar J. 3,5-Dibenzoyl-1,4-dihydropyridines: synthesis and MDR reversal in tumor cells. Bioorg. Med. Chem. 2002;10:1051-5. [PubMed ID: 11836114].
-
12.
Firuzi O, Javidnia K, Mansourabadi E, Saso L, Mehdipour AR, Miri R. Reversal of multidrug resistance in cancer cells by novel asymmetrical 1,4-dihydropyridines. Arch. Pharm. Res. 2013;36:1392-1402. [PubMed ID: 23674129].
-
13.
Bazargan L, Fouladdel S, Shafiee A, Amini M, Ghaffari SM, Azizi E. Evaluation of anticancer effects of newly synthesized dihydropyridine derivatives in comparison to verapamil and doxorubicin on T47D parental and resistant cell lines in vitro. Cell Biol. Toxicol. 2008;24:165-174. [PubMed ID: 17805981].
-
14.
Lee YS, Sayeed MM, Wurster RD. Inhibition of cell growth and intracellular Ca2+ mobilization in human brain tumor cells by Ca2+ channel antagonists. Mol. Chem. Neuropathol. 1994;22:81-95. [PubMed ID: 7522451].
-
15.
Yoshida J, Ishibashi T, Nishio M. Antiproliferative effect of Ca2+ channel blockers on human epidermoid carcinoma A431 cells. Eur. J. Pharmacol. 2003;472:23-31. [PubMed ID: 12860469].
-
16.
Sirisha K, Achaiah G, Reddy VM. Facile synthesis and antibacterial, antitubercular, and anticancer activities of novel 1,4-dihydropyridines. Arch. Pharm. Chem. Life Sci. 2010;343:342-352.
-
17.
Engi H, Sakagami H, Kawase M, Parecha A, Manvar D, Kothari H, Adlakha P, Shan A, Motohashi NIO, Molnar J. Tumour-specific cytotoxicity and MDR-reversal activity of dihydropyridines. In Vivo. 2006;20:637-644. [PubMed ID: 17091771].
-
18.
Foroughinia F, Javidnia K, Amirghofran Z, Mehdipour AR, Mir R. Design and synthesis of new symmetrical derivatives of dihydropyridine containing a pyridyl group on the 3, 5-positions and evaluation of their cytotoxic and multidrug resistance reversal activity. J. Pharm. Pharmacol. 2008;60:1481-9. [PubMed ID: 18957169].
-
19.
Abbas HAS, El Sayed WA, Fathy NM. Synthesis and antitumor activity of new dihydropyridine thioglycosides and their corresponding dehydrogenated forms. Eur. J. Med. Chem. 2010;45:973-982. [PubMed ID: 20004044].
-
20.
Hahn KA, Legendre AM, Schuller HM. Amputation and dexniguldipine as treatment for canine appendicular osteosarcoma. J. Cancer Res. Clin. 1997;123:34-8.
-
21.
Zhu LJ, Zhuang CL, Lei N, Sheng CQ, Guo W, Miao ZY, Liu WF, Yao JZ, Zhang WN. Synthesis and pharmacological evaluation of novel homocamptothecin-dihydropyridine derivative conjugates as potent topoisomerase I inhibitors. Aust. J. Chem. 2011;64:1390-6.
-
22.
Miri R, Javidnia K, Hemmateenejad B, Azarpira A, Amirghofran Z. Synthesis, cytotoxicity, QSAR, and intercalation study of new diindenopyridine derivatives. Bioorg. Med. Chem. 2004;12:2529-36. [PubMed ID: 15110835].
-
23.
Miri R, Motamedi R, Rezaei MR, Firuzi O, Javidnia A, Shafiee A. Design, synthesis and evaluation of cytotoxicity of novel chromeno [4,3-b] quinoline derivatives. Arch. Pharm. 2010;1:111-8.
-
24.
Sarkarzadeh H, Miri R, Firuzi O, Amini M, Razzaghi-Asl N, Edraki N, Shafiee A. Synthesis and antiproliferative activity evaluation of imidazole-based indeno[1,2-b]quinoline-9,11-dione derivatives. Arch. Pharm. Res. 2013;36:436-447. [PubMed ID: 23440577].
-
25.
Clemens RJ, Hyatt JA. Acetoacetylation with 2,2,6-trimethyl-4H-1,3-dioxin-4-one: a convenient alternative to diketene. J. Org. Chem. 1985;50:2431-5.
-
26.
Razzaghi-Asl N, Firuzi O, Hemmateenejad B, Javidnia K, Edraki N, Miri R. Design and synthesis of Novel 3,5-bis-N-(aryl/heteroaryl) carbamoyl-4-aryl-1,4-dihydropyridines as small molecule BACE-1 inhibitors. Bioorg. Med. Chem. 2013;21:6893-6909. [PubMed ID: 24113238].
-
27.
Mohammadhosseini N, Pordeli M, Safavi M, Firoozpour L, Amin F, Ardestani SK, Edraki N, Shafiee A, Foroumadi A. Novel n-2-(Furyl)-2-(chlorobenzyloxyimino) ethyl piperazinyl quinolones: Synthesis, cytotoxic evaluation and structure-activity relationship. Iran. J. Pharm. Res. 2015;14:1095-1103. [PubMed ID: 26664376].