Abstract
Keywords
Noscapine D-glucose intracellular calcium levels NO Oxygen-Glucose Deprivation
Introduction
Diabetes mellitus is a common metabolic disease characterized by hyperglycemia which often leads to nerve injury probably through the induction of systemic and neuronal oxidative stress (1, 2). It has been shown that high glucose (HG) induces oxidative stress and cell death via NADPH oxidase dependent generation of reactive oxygen species and NO production (3, 4).
During cerebrovascular accidents, ischemia induced metabolic deficiency appears to promote a cascade of events leading to neuronal cell death via both necrosis and apoptosis (-). In other words, ischemia induced glucose and/or oxygen deficiencies can cause both cellular and functional damage to the brain (8, 9). Also, neuronal cells deprived of means of production of energy appear to be highly susceptible to excitotoxicity (8, -). Although glucose appears to be essential in normal function of the nervous system (14), there is conflicting evidence regarding the effects of extracellular glucose concentration on various forms of ischemic injury (9). Some studies have found that hyperglycemia worsens the effects of ischemic insult, probably due to enhanced lactate generation which results in tissue acidosis and leads to generation of reactive oxygen species (9, -). In contrast, other studies have found that elevated glucose levels before hypoxic insult reduced neuronal damage (9, -), while other studies have demonstrated that hyperglycemia can decrease glutamate levels released during ischemia (9, 15, -). Corroborating evidence of neuroprotective effects of glucose was provided by Dave KR, Pileggi A and Raval AP () who showed that exposure of hippocampal slices to hypoglycemia prior to ischemic insult exacerbated ischemia induced damage.
Research on noscapine, a non-addictive antitussive alkaloid of opium, as a neuroprotective agent in our laboratory had previously shown it efficacy in attenuating brain injury after hypoxia in neonatal rats (33). Also, in a limited clinical trial, it was shown that noscapine reduced sharply the mortality rate of stroke patients (34).
In order to delineate the mechanism of the neuroprotective effects of noscapine, in this study, we investigated if noscapine could protect primary murine fetal cortical neurons exposed to oxygen-glucose deprivation/24 h. recovery during high glucose condition. The intracellular free calcium concentration and NO production were measured as two important factors during ischemic insult.
Experimental
Chemicals and reagents
Noscapine was obtained from Temad-D Pharmaco-Chemical Company (Tehran, Iran). The stock solution was prepared at 1 mol/L in dimethyl sulfoxide (DMSO) and stored at –20 °C. RPMI-1640, glutamine-free Dulbecco's Modified Eagle's Medium (DMEM), foetal bovine serum (FBS), horse serum, penicillin- streptomycin, cytosine arabinoside, 3-(4,5-dimethylthiazol-2-yl)-2,5-diphenyl-tetrazolium bromide (MTT), D-glucose, fura-2 AM, poly-L-lysine, NG nitro-L-arginine methyl ester (L-NAME), Mannitol, sodium-azide (NaN3) and modified Griess reagent were purchased from Sigma Chemical Co. (St. Louis, MO, USA). All other chemicals used were of the purest grade available from regular commercial sources.
Murine Neuronal Cell Cultures
Primary cultures of cerebral cortex neurons were obtained from mouse embryos (E15–18) as described previously (35, 36). Briefly, timed-pregnant mice were anaesthetised with chloroform and killed by cervical dislocation. Foetuses were removed and decapitated with small scissors. Skull cap were removed, cerebral cortex was isolated and the cortex was cut into small pieces. The tissue was then dissociated by repeated pipetting. The resulting homogenate was centrifuged at 300×g and 20 °C for 5 min and plated on poly-L-lysine-coated 12-well culture dishes at a density of 6×104 cells in RPMI-1640 medium supplemented with 10% FBS, 5% horse serum, 100 IU/mL penicillin and 100 µg/ mL streptomycin. Poly-L-lysine (150,000–300,000 MW) was dissolved in deionised water, and the plate bases were filled with this solution (20 µg/ mL) 24 h. before the experiment. After 5 min standing at room temperature, the solution was aspirated, and the plates were left to dry in a laminar flow bench overnight. The culture dishes were kept at 37 °C in humidified 95% air and 5% CO2. After 24–48 h. 10 µM cytosine arabinoside (cytosine 1-β-D-arabinofuranoside) was added to the culture medium to inhibit the proliferation of non-neuronal cells. The culture medium was changed twice weekly, and the neurons were used for experiments after 13–16 days of incubation. All experiments were approved by the Institute of Animal Care Committee at the Iran University of Medical Sciences.
Oxygen–glucose deprivation and drug exposure
Procedures for oxygen–glucose deprivation (OGD) were performed as described previously (37). Neuronal cells were incubated for 24 h. in RPMI-1640 containing 25, 100 mM D-glucose in presence or absence of noscapine. The culture medium was replaced with glucose/glutamine-free DMEM, and cells were exposed to OGD for 30 min in a small anaerobic chamber previously filled with 95 % (v/v) N2 and 5 % (v/v) CO2 at 37 °C. To terminate OGD, the chamber was opened, the medium was replaced with RPMI-1640 and the cultures were then placed in an incubator with 5 % CO2 for 24 h. To examine the effects of noscapine, the drug (0.5-2 µM) was added to cultures in media containing 25 mM or 100 mM D-glucose 24 h. before OGD. The concentrations of noscapine were chosen based on the results of preliminary experiments to identify nontoxic levels (data not shown). All measurements were repeated at least three times.
Analysis of neuronal cell viability
Neuronal cell viability was measured using the colorimetric MTT assay, as previously described by Mosmann T () . Briefly, cells were incubated with 0.5mg/mL MTT in RPMI at 37 ºC under 5 % CO2 for 3 h. The blue formazan reduction product, which is generated by the action of the succinate dehydrogenase on the dye only in living cells, was dissolved in 100µL DMSO, and its absorbance was read at 570nm using a Dynex MMX microplate reader (Dynex, Richfield, MN, USA). The data were expressed as the percentage of viable cells in oxygen–glucose deprivation-exposed plates compared with control normoxic plates.
Measurement of nitrite levels
The level of nitrite as an indicator of NO production in the culture medium was measured using modified Griess reagent. In brief, after the experiment, the medium in each well was removed and centrifuged at 10,000 g for 10 min at 20 ºC. Then, 100 µL of the supernatant was mixed with an equal volume of Griess reagent at room temperature for 10 min, and the absorbance was measured at 540 nm using a microplate reader. The nitrite concentration was determined from a sodium nitrite standard curve. Plates not exposed to OGD were used as external control, and plates exposed to OGD without the addition of any drug were used as an internal control.
Measurement of intracellular free calcium
Measurement of intracellular free calcium concentration was performed using the Ca2+-sensitive indicator fura-2 bya Olympus IX-71 inverted microscope and CCD camera equipped with an automated filter changer. Cortical cell cultures grown on a coverslips were loaded with 4 µM fura-2/AM at room temperature for 30 min. Cells were washed three times with a salt solution containing (in mM): 140 NaCl, 3 KCl, 2.5 CaCl2, 1.2 MgCl2, 7.7 glucose and 10 HEPES, pH 7.4. The fura-2 fluorescent images (Ex 340/380 nm; Em 510 nm) were acquired. Ratio metric analyses were carried out using Image J software (NIH).
Statistical analysis
All results are expressed as the means ± S.E.M. The significance of differences was evaluated with student's t-test. A probability level of P<0.05 was considered statistically significant.
Results
The effects of noscapine in high glucose conditions on primary cortical neurons exposed to 30 minutes OGD
We examined the effect of D-glucose in cell viability as measured by the MTT assay. Our results demonstrated that after 24 h. of exposure to 25 or 100 mM D-glucose neuronal cell viability was reduced by 73.5±1.04 and 53.15±0.47 respectively. As shown in Figure 1 the decrease in cell viability induced by HG media was concentration dependent and statistically significant when compared to the control cultures which were incubated in media with normal glucose concentration (4.5mM). We explored the effects of high osmolarity on neuronal cell death by pre-treating neuronal culture with 100 mM mannitol (negative control for osmolarity). Our results indicated that there was no significant difference in cell viability between neuronal cultures in control group (100 %) versus 100 mM mannitol (98.67±0.1). Thus any observed change in cell viability is due, directly, to the effect of glucose on neuronal cells.
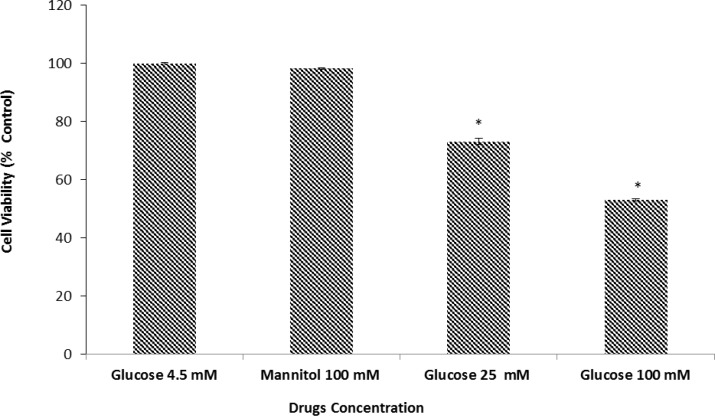
Exposure to 30 min of OGD decreased viability of neuronal cells which had been kept in media with normal glucose to 55.58±1.09. Neuronal cells pretreated for 24 h. with 25 or 100 mM glucose prior to OGD exposure showed an increase in cell viability (71.47±0.79 and 80.56±0.66 respectively) when compared with normal glucose medium (Figure 2a-b).
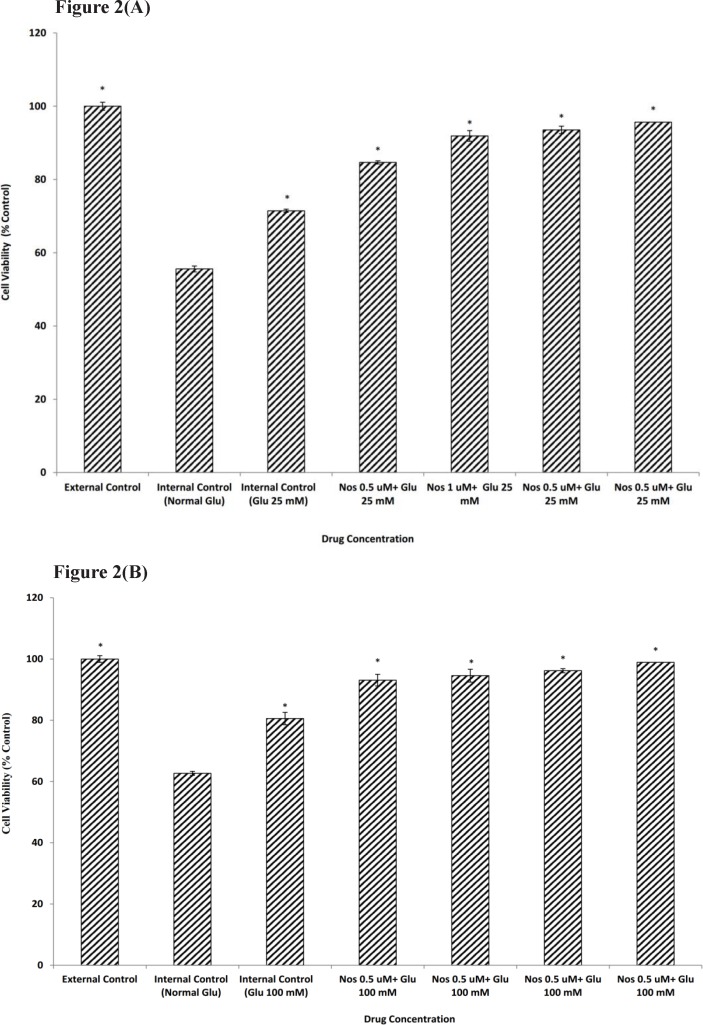
In order to determine if noscapine (0.5-2 µM) could affect neuronal cells viability in the presence of 25 and 100 mM D-glucose prior to 30 min OGD, cultures were pretreated with noscapine and HG. Our results showed that in the presence of noscapine protective effect of glucose was potentiated significantly compared to that of D-glucose alone (Figure2a-b). The increase in cell viability was 95.67±1.02 and 98.91±0.66 for 2 µM noscapine, the most effective concentration, in the presence of 25 and 100 mM D-glucose.
The effects of noscapine in high glucose conditions on NO production in primary cortical neurons exposed to 30 minutes OGD
We examined the effects of noscapine in HG conditions on NO production in primary cortical neurons exposed to 30 min OGD. As shown in Figure. 3a-b, under normal glycemic pre-treatment, neuronal cells exposed to 30 min OGD, produced significantly more nitrite compared to groups which were not exposed to OGD (P<0.001). Cortical neurons pre-treated with 25 or 100 mM D-glucose prior to exposure to 30 min OGD produced significantly less NO 1.42±0.09 µM and 1.27±0.08 µM respectively. Pre-treating neurons with noscapine (0.5-2µM) during HG condition (25 or 100 mM) induced a further decrease in NO production as compared with HG alone (P<0.001). The neuronal NO production induced by 30 min of OGD was attenuated by 100 µM L-NAME, a nitric oxide synthase inhibitor (Figure 3a-b).
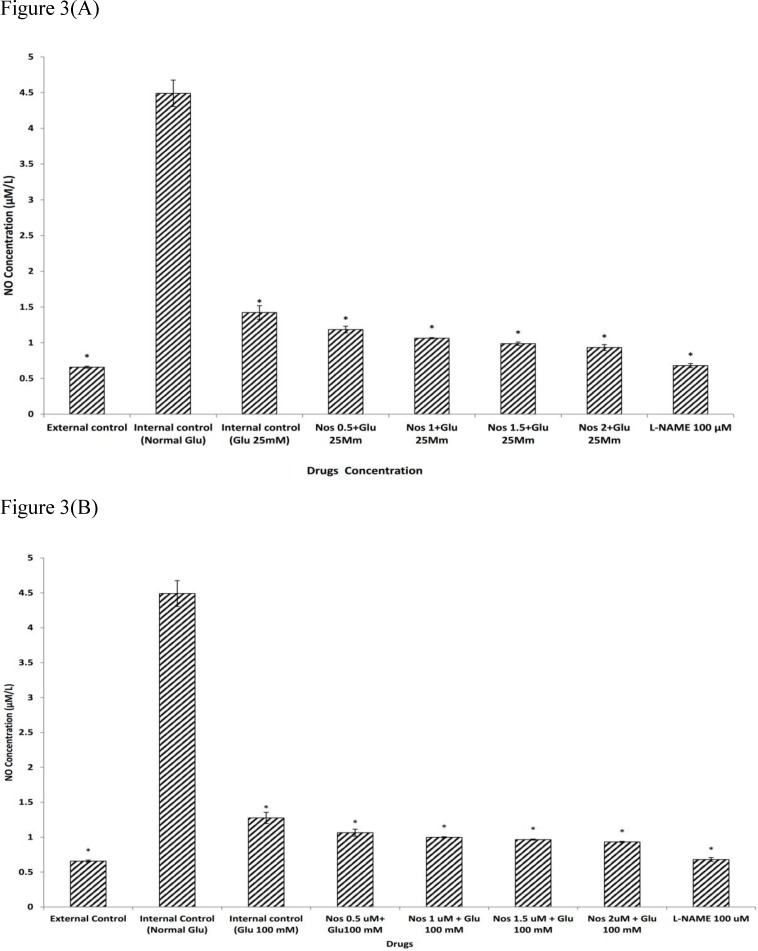
The effects of noscapine in high glucose conditions on intracellular calcium levels against chemical OGD
To determine the intracellular calcium levels during ischemia, the neuronal cells were exposed to 30 min sodium-azide (4 mM) as a model of chemical OGD in the absence of glucose. 4 mM NaN3 was able to induce a significant increase in (Ca2+)i levels in cortical neurons bathed in Ca2+-containing medium (169.87±4.73) (Figure.4-5). Exposure of cortical neurons to sodium azide and 25 mM or 100 mM D-glucose after 30 min of OGD, decreased (Ca2+)i levels by 161.23±4.29 and 143.33±2.23 respectively. Furthermore, the ischemia-evoked increase in (Ca2+)i levels was decreased by 150.82±2.7 and 124.12±1.87 in the presence of 2 µM noscapine with 25 or 100 mM D-glucose respectively (Figure 4-5).
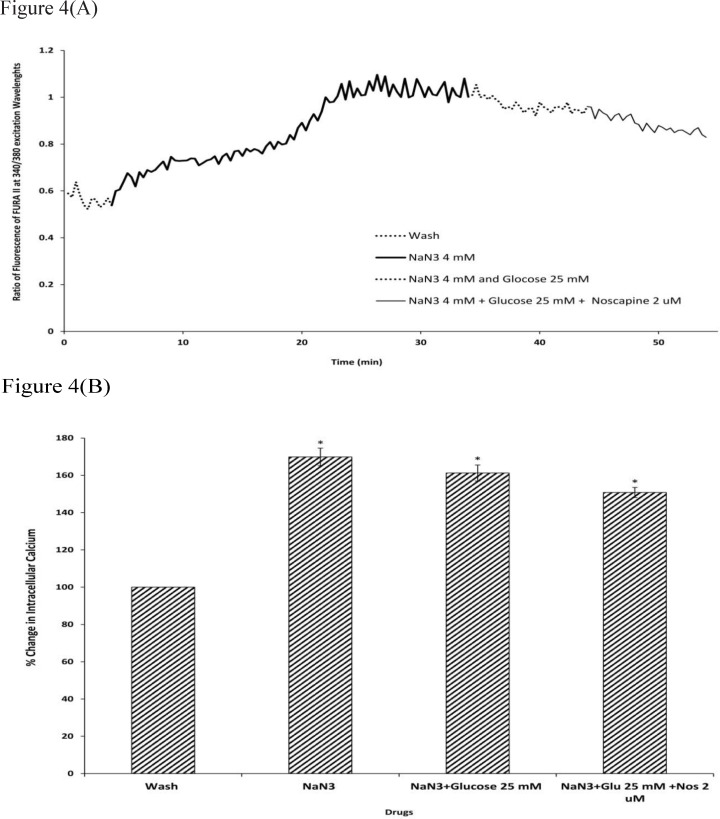
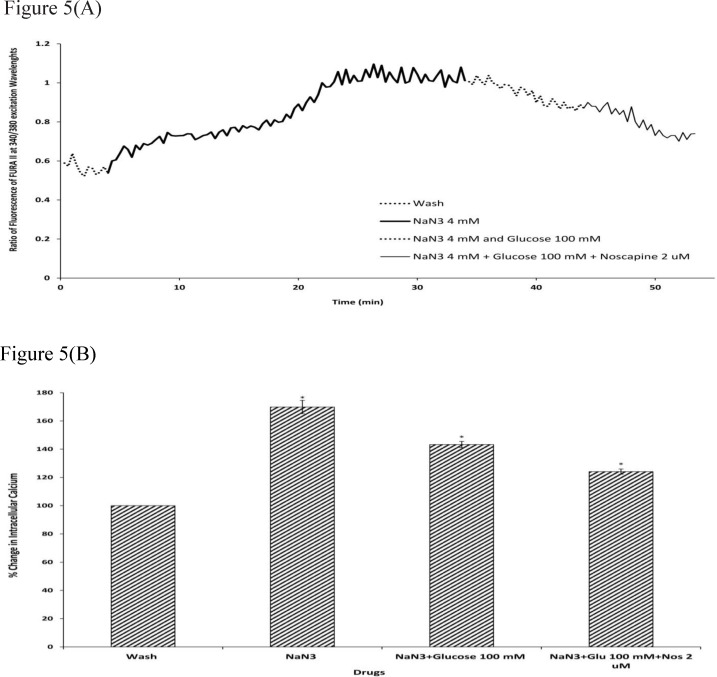
Discussion
Hyperglycemia appears to be an important factor in the development of diabetic neuropathy (2). It induces neuronal damage and dysfunction probably via the generation of reactive oxygen species (2, 39). As such, many studies have shown that HG does indeed cause neuronal cell death (-). However, there is conflicting evidence regarding the role of extracellular glucose during brain ischemic insults (9). It is generally held that glucose supplies the essential energy needs of the brain and alteration of glucose metabolism may result in dysfunction of the CNS (8, 14). A number of studies have obtained evidence for the beneficial effects of enhanced glucose entry into neurons during ischemia (14, 40, 41). These works suggest a protective role for glucose against excitotoxicity and hypoxic ischemia. In the current study, we aimed to show the effects of HG on intracellular Ca2+ levels, NO production, and general cell viability in the absence and presence of noscapine which we had previously shown to have neuroprotective effects of its own (42).
We examined the effect of D-glucose (25 or 100 mM) on neuronal cell death. Cortical cultures were exposed, for 24 h. to 4.5 mM (as normal glucose control), 25 and 100 mM glucose (as high glucose). Our results showed that 25 and 100 mM D-glucose could significantly reduce cell viability in a concentration dependent fashion as compared with control. In fact, neuronal cell viability treated with 100 mM glucose was markedly reduced as compared to 25 mM D-glucose. This was consistent with previous studies demonstrating that high concentrations of glucose induced neuronal injury in an in-vitro model (2, 4, 43). For example, Koshimura K, Tanaka J, Murakami Y and Kato Y () showed that HG increased NO production and induced cell death in differentiated PC12 cells. Also, Vincent AM, McLean LL, Backus C and Feldman EL () demonstrated that in neurons, HG caused oxidative stress, induced mitochondrial dysfunction and programmed cell death. Thus, the data obtained by us and other workers, confirmed exposure to HG for 24 h. or more, to be detrimental to neuronal cells.
However, there is data to suggest a neuroprotective role for short period exposure to HG after ischemia (40). Here the mechanism of protection is not completely delineated. Toping up of intracellular glucose stores has been proposed to be the reason for this effect (9, 44). Thus, to determine whether a short exposure to HG could protect cortical neuronal cells from OGD insult, cells were pre-incubated for 24 h. in RPMI-1640 containing 25 or 100 mM glucose prior to 30 min OGD. Exposure to HG reduced cell death due to OGD. This effect was more visible for 100 mM D-glucose than that of 25 mM D-glucose.
Other workers have shown HG to be protective against the effects of excitotoxins (8, 9, 41). Seo SY, Kim EY, Kim H and Gwag BJ () showed that although pretreatment with 25 mM glucose did not induce great protection against NMDA evoked neuronal death, 100 mM glucose pretreatment enhanced cell survival after treatment with NMDA. It has been demonstrated that OGD induces cell death by enhancing the release of extracellular glutamate which in turn increases activation of NMDA receptors leading to increased intracellular Ca2+ levels and augmented NO production (-). Thus the data obtained by us is in part agreement with the work of many other researchers (8, 9, 14, 40, 41).
All this suggests an important role for intracellular Ca2+ and NO in both excitotoxicity and OGD induced cell death.
In a previous study carried out in this lab, effects of noscapine on OGD induced death in primary cortical neurons had been studied (42). Noscapine appeared to impart protection against ischemia by attenuating OGD induced NO production. In this study we investigated the possibility that noscapine could potentiate the protective effects of HG from OGD in primary cortical neurons. When neuronal cells pre-treated with noscapine (0.5-2 µM) subsequent the addition of HG ( 25 or 100 mM) and then subjected to 30 min OGD, the cell viability increased as compared with the cell pre-treated with high-glucose alone. In order to find out the neuroprotective effect of noscapine in HG condition, we set out to measure the change in Ca2+ and NO after OGD in cells pretreated with HG.
Sodium azide/glucose deprivation (chemical OGD) model was used to create in-vitro ischemic conditions. This model has been shown to elicit electrophysiological and neurochemical changes that are similar to the OGD model of ischemia. While this model having the advantage of producing neurochemical changes more rapidly and reproducibly (49, 50), thus facilitating the recording of changes in (Ca2+)i (51). Our results showed that during chemical OGD the (Ca2+)i was significantly increased compared to control condition. This is consistent with previous studies indicating that NaN3 could increase neuronal (Ca2+)i, as a consequence of energy depletion (52).
We next examined the (Ca2+)i level in neuronal cells exposed to 25 or 100 mM D-glucose after 30 min of chemical OGD treatment. The results suggested that exposure to 25 or 100 mM D-glucose was able to reverse the effects of chemical OGD on intracellular Ca2+ levels in cultured cortical neurons. Also, 100 mM glucose was more effective in decreasing (Ca2+)i levels than 25 mM glucose. Chan J and Greenberg DA () showed that in PC12 cells, increasing glucose concentration in the culture medium by a factor of 4, attenuated ischemic injury by reducing the dihydropyridine-sensitive and depolarization-induced increase in Ca2+. These results suggested that HG might decrease neuronal cell death by reducing (Ca2+)i levels in neuronal cells.
Finally, our findings demonstrated that cells exposed to D-glucose together with 2 µM noscapine following chemical OGD had significantly lower (Ca2+)i compared to D-glucose treatment alone. This data suggests that noscapine reinforced the protective effect of HG via reduction in intracellular Ca2+ levels. Also, noscapine potentiated the effects of 100 mM glucose more than it decreased the effects of 25 mM glucose. However, because 100 mM D-glucose could considerably reduce cell death during ischemic insult, the neuroprotective effect of 2 µM noscapine in presence of 100 mM D-glucose also could better act on neuronal cells to reduce (Ca2+)i following chemical OGD.
Many studies have been shown that NO is involved in both physiological and pathological events, and is believed to play different roles in both lethal and sub-lethal events (47, 54). Several studies have found evidence indicating an involvement of NO synthase (NOS) in glutamate-induced excitotoxicity and there were some corroborating data that NO production increases during ischemia (35, 45, 47, -). For example, it has been shown that during OGD, calcium enters the neurons through NMDA receptor and/or L-type voltage-gated calcium channel activation and activates NOS which leads to NO production (45). Zhang M, Ning G-M, Hong D-H, Yang Y, Kutor J and Zheng X-X () had shown that Ca2+-free medium markedly inhibited the OGD-induced increase in NO, suggesting that the inhibition of Ca2+ might cause the decrease of NO synthesis.
Our results confirmed that in neuronal cells exposed to 30 min OGD, NO production was significantly increased, supporting a role for NO in the cascade of events leading to OGD-induced cell death (45). However, the exposure of neuronal cells to 25 or 100 mM D-glucose could decrease the NO production during 30 min OGD. After that neuronal cells were pre-treated with noscapine (0.5-2 µM) in the presence of the 25 or 100 mM D-glucose and then subjected to 30 min OGD. The results indicated that the NO production was significantly reduced as compared with 25 or 100 mM D-glucose alone. This suggested that noscapine also increased neuroprotective effect of HG by reducing NO production. In addition, when the cultures were pre-treated with the NOS inhibitor, L-NAME, NO production was significantly decreased and returned to the level of the external control. Suggesting that NOS inhibitors could markedly decrease the NO production after NMDA receptor activation, and decreased excitotoxic injury in cultured neurons (45, 58, 59)
In conclusion, noscapine potentiated the neuroprotective effect of HG (25 and 100 mM) treatment prior to OGD. The enhanced cell viability after treatment with both noscapine and HG was mirrored by a dampening of OGD induced intracellular Ca2+ and NO rise. These observations support the hypothesis that calcium homeostasis and NO production have crucial roles in excitotoxicity process.
Acknowledgements
References
-
1.
Tomlinson DR, Gardiner NJ. Glucose neurotoxicity. Nat. Rev. Neurosci. 2008;9:36-45. [PubMed ID: 18094705].
-
2.
Zhang WF, Xu YY, Xu KP, Wu WH, Tan GS, Li YJ, Hu CP. Inhibitory effect of selaginellin on high glucose-induced apoptosis in differentiated PC12 cells: role of NADPH oxidase and LOX-1. Eur. J. Pharmacol. 2012;694:60-8. [PubMed ID: 22964466].
-
3.
Lee HS, Son SM, Kim YK, Hong KW, Kim CD. NAD (P) H oxidase participates in the signaling events in high glucose-induced proliferation of vascular smooth muscle cells. Life Sci. 2003;72:2719-30. [PubMed ID: 12679189].
-
4.
Koshimura K, Tanaka J, Murakami Y, Kato Y. Involvement of nitric oxide in glucose toxicity on differentiated PC12 cells: prevention of glucose toxicity by tetrahydrobiopterin, a cofactor for nitric oxide synthase. Neurosci. Res. 2002;43:31-8. [PubMed ID: 12074839].
-
5.
Banasiak KJ, Xia Y, Haddad GG. Mechanisms underlying hypoxia-induced neuronal apoptosis. Prog Neurobiol. 2000;62:215-50. [PubMed ID: 10840148].
-
6.
Zipfel GJ, Babcock DJ, Lee JINMOO, Choi DW. Neuronal apoptosis after CNS injury: the roles of glutamate and calcium. J. Neurotrauma. 2000;17:857-69. [PubMed ID: 11063053].
-
7.
Cater HL, Chandratheva A, Benham CD, Morrison III B, Sundstrom LE. Lactate and glucose as energy substrates during, and after, oxygen deprivation in rat hippocampal acute and cultured slices. J. Neurochem. 2003;87:1381-90. [PubMed ID: 14713294].
-
8.
Seo SY, Kim EY, Kim H, Gwag BJ. Neuroprotective effect of high glucose against NMDA, free radical, and oxygen–glucose deprivation through enhanced mitochondrial potentials. J. Neurosci. 1999;19:8849-55. [PubMed ID: 10516304].
-
9.
Tian GF, Baker AJ. Protective effect of high glucose against ischemia-induced synaptic transmission damage in rat hippocampal slices. J. Neurophysiol. 2002;88:236-48. [PubMed ID: 12091549].
-
10.
Simon R, Swan J, Griffiths T, Meldrum B. Blockade of N-methyl-D-aspartate receptors may protect against ischemic damage in the brain. Science (New York, NY ). 1984;226:850-2.
-
11.
Wieloch T. Hypoglycemia-induced neuronal damage prevented by an N-methyl-D-aspartate antagonist. Science. 1985;230:681-3. [PubMed ID: 2996146].
-
12.
Monyer H, Goldberg MP, Choi DW. Glucose deprivation neuronal injury in cortical culture. Brain Res. 1989;483:347-54. [PubMed ID: 2565150].
-
13.
Cebers G, Cebere A, Liljequist S. Metabolic inhibition potentiates AMPA-induced Ca2+ fluxes and neurotoxicity in rat cerebellar granule cells. Brain Res. 1998;779:194-204. [PubMed ID: 9473670].
-
14.
Seo SY, Kim EY, Kim H, Jou I, Gwag BJ. Attenuation of Neuronal Death by NMDA and Oxygen‐Glucose Deprivation in Cortical Neurons Maintained in High Glucose. Ann. N.Y. Acad. Sci. 1999;893:396-9. [PubMed ID: 10672276].
-
15.
Guyot L, Diaz F, O'Regan M, Song D, Phillis J. Topical glucose and accumulation of excitotoxic and other amino acids in ischemic cerebral cortex. Horm Metab Res. 2000;32:6-9. [PubMed ID: 10727006].
-
16.
Gisselsson L, Smith ML, Siesjö BK. Hyperglycemia and focal brain ischemia. J. Cereb. Blood Flow Metab. 1999;19:288-97. [PubMed ID: 10078881].
-
17.
Katsura K, Kristian T, Smith ML, Siesjo BK. Acidosis induced by hypercapnia exaggerates ischemic brain damage. J. Cereb. Blood Flow Metab. 1994;14:243-50. [PubMed ID: 8113321].
-
18.
Kondo F, Kondo Y, Makino H, Ogawa N. Delayed neuronal death in hippocampal CA1 pyramidal neurons after forebrain ischemia in hyperglycemic gerbils: amelioration by indomethacin. Brain Res. 2000;853:93-8. [PubMed ID: 10627312].
-
19.
Li PA, Siesjö B. Role of hyperglycaemia‐related acidosis in ischaemic brain damage. Acta Physiol. Scand. 2003;161:567-80. [PubMed ID: 9429666].
-
20.
Li PA, Liu GJ, He QP, Floyd RA, Siesjö BK. Production of hydroxyl free radical by brain tissues in hyperglycemic rats subjected to transient forebrain ischemia. Free Radic. Biol. Med. 1999;27:1033-40. [PubMed ID: 10569636].
-
21.
Lundgren J, Smith ML, Siesjö BK. Effects of dimethylthiourea on ischemic brain damage in hyperglycemic rats. J. Neurol. Sci. 1992;113:187-97. [PubMed ID: 1487754].
-
22.
Rehncrona S, Rosén I, Siesjö BK. Brain lactic acidosis and ischemic cell damage: 1. Biochemistry and neurophysiology. J. Cereb. Blood Flow Metab. 1981;1:297-311. [PubMed ID: 7328145].
-
23.
Smith ML, Von Hanwehr R, Siesjö B. Changes in extra-and intracellular pH in the brain during and following ischemia in hyperglycemic and in moderately hypoglycemic rats. J. Cereb. Blood Flow Metab. 1986;6:574-83. [PubMed ID: 3760041].
-
24.
Ginsberg MD, Prado R, Dietrich WD, Busto R, Watson BD. Hyperglycemia reduces the extent of cerebral infarction in rats. Stroke. 1987;18:570-4. [PubMed ID: 3109078].
-
25.
Kraft SA, Larson C, Shuer LM, Steinberg GK, Benson GV, Pearl RG. Effect of hyperglycemia on neuronal changes in a rabbit model of focal cerebral ischemia. Stroke. 1990;21:447-50. [PubMed ID: 2309269].
-
26.
Roos MW. Effects of hyperglycemia on small cerebral infarctions in awake rabbits. Ups. J. Med. Sci. 1999;104:145-61. [PubMed ID: 10422217].
-
27.
Vannucci RC, Brucklacher RM, Vannucci SJ. The effect of hyperglycemia on cerebral metabolism during hypoxia-ischemia in the immature rat. J. Cereb. Blood Flow Metab. 1996;16:1026-33. [PubMed ID: 8784248].
-
28.
Zasslow MA, Pearl RG, Shuer LM, Steinberg G, Lieberson R, Larson Jr C. Hyperglycemia decreases acute neuronal ischemic changes after middle cerebral artery occlusion in cats. Stroke. 1989;20:519-23. [PubMed ID: 2929029].
-
29.
Choi KT, Illievich UM, Zornow MH, Scheller MS, Strnat MAP. Effect of hyperglycemia on peri-ischemic neurotransmitter levels in the rabbit hippocampus. Brain Res. 1994;642:104-10. [PubMed ID: 7913389].
-
30.
Kanthan R, Shuaib A, Griebel R, Miyashita H, Kalra J. Glucose-induced decrease in glutamate levels in ischemic human brain by in-vivo microdialysis. Neurosci. Lett. 1996;209:207-9. [PubMed ID: 8736647].
-
31.
Phillis J, Song D, O'regan M. Effect of hyperglycemia on extracellular levels of amino acids and free fatty acids in the ischemic/reperfused rat cerebral cortex. Brain Res. 1999;837:177-83. [PubMed ID: 10434000].
-
32.
Dave KR, Pileggi A, Raval AP. Recurrent hypoglycemia increases oxygen glucose deprivation-induced damage in hippocampal organotypic slices. Neurosci. Lett. 2011;496:25-9. [PubMed ID: 21466838].
-
33.
Mahmoudian M, Siadatpour Z, Ziai S, Mehrpour M, Benaissa F, Nobakht M. Reduction of the prenatal hypoxic-ischemic brain edema with noscapine. Acta Physiol Hung. 2003;90:313-8. [PubMed ID: 14708873].
-
34.
Mahmoudian M, Mehrpour M, Benaissa F, Siadatpour Z. A preliminary report on the application of noscapine in the treatment of stroke. Eur. J. Clin. Pharmacol. 2003;59:579-81. [PubMed ID: 14517705].
-
35.
Dawson VL, Dawson TM, London ED, Bredt DS, Snyder SH. Nitric oxide mediates glutamate neurotoxicity in primary cortical cultures. Proc. Natl. Acad. Sci. 1991;88:6368-71. [PubMed ID: 1648740].
-
36.
Dawson VL, Dawson T, Bartley D, Uhl G, Snyder S. Mechanisms of nitric oxide-mediated neurotoxicity in primary brain cultures. J. Neurosci. 1993;13:2651-61. [PubMed ID: 7684776].
-
37.
Frantseva MV, Carlen PL, El-Beheiry H. A submersion method to induce hypoxic damage in organotypic hippocampal cultures. J. Neurosci. Methods. 1999;89:25-31. [PubMed ID: 10476680].
-
38.
Mosmann T. Rapid colorimetric assay for cellular growth and survival: application to proliferation and cytotoxicity assays. J. Immunol. Methods. 1983;65:55-63. [PubMed ID: 6606682].
-
39.
Edwards JL, Vincent AM, Cheng HT, Feldman EL. Diabetic neuropathy: mechanisms to management. Pharmacol. Ther. 2008;120:1-34. [PubMed ID: 18616962].
-
40.
Malliopoulou V, Xinaris C, Mourouzis I, Cokkinos AD, Katsilambros N, Pantos C, Kardami E, Cokkinos DV. High glucose protects embryonic cardiac cells against simulated ischemia. Mol. Cell. Biochem. 2006;284:87-93. [PubMed ID: 16541202].
-
41.
Moon CH, Jung YS, Kim MH, Park RM, Lee SH, Baik EJ. Protein kinase C inhibitors attenuate protective effect of high glucose against hypoxic injury in H9c2 cardiac cells. Jpn. J.Physiol. 2000;50:645-9. [PubMed ID: 11173560].
-
42.
Vahabzadeh G, Rahbar-Roshandel N, Ebrahimi S-A, Mahmoudian M. Neuroprotective effect of noscapine on cerebral oxygen–glucose deprivation injury. Pharmacol Rep. 2015;67:281-8. [PubMed ID: 25712651].
-
43.
Vincent AM, McLean LL, Backus C, Feldman EL. Short-term hyperglycemia produces oxidative damage and apoptosis in neurons. FASEB J. 2005;19:638-40. [PubMed ID: 15677696].
-
44.
Siemkowicz E, Hansen AJ. Clinical restitution following cerebral ischemia in hypo, normo and hyperglycemic rats. Acta Neurol. Scand. 1978;58:1-8. [PubMed ID: 30250].
-
45.
(Tavakoli-Far B, Rahbar-Roshandel N, Rahimi-Moghaddam P, Mahmoudian M. Neuroprotective effects of mebudipine and dibudipine on cerebral oxygen–glucose deprivation/reperfusion injury. Eur. J. Pharmacol. 2009;610:12-7. [PubMed ID: 19285496].
-
46.
Kostandy BB. The role of glutamate in neuronal ischemic injury: the role of spark in fire. Neurol. Sci. 2012;33:223-37. [PubMed ID: 22044990].
-
47.
Zhang M, Ning G-M, Hong D-H, Yang Y, Kutor J, Zheng X-X. The Influence of Oxygen-Glucose Deprivation on Nitric Oxide and Intracellular Ca2+ in Cultured Hippocampal Neurons. Acta Biochim Biophys Sinica. 2003;35:561-6.
-
48.
Hardingham GE. Coupling of the NMDA receptor to neuroprotective and neurodestructive events. Biochem. Soc. Trans. 2009;37:1147-60. [PubMed ID: 19909238].
-
49.
Murai Y, Ishibashi H, Koyama S, Akaike N. Ca2+-activated K+ currents in rat locus coeruleus neurons induced by experimental ischemia, anoxia, and hypoglycemia. J. Neurophysiol. 1997;78:2674-81. [PubMed ID: 9356417].
-
50.
Finley M, Fairman D, Liu D, Li P, Wood A, Cho S. Functional validation of adult hippocampal organotypic cultures as an in vitro model of brain injury. Brain Res. 2004;1001:125-32. [PubMed ID: 14972661].
-
51.
Katnik C, Guerrero WR, Pennypacker KR, Herrera Y, Cuevas J. Sigma-1 receptor activation prevents intracellular calcium dysregulation in cortical neurons during in vitro ischemia. J. Pharmacol. Exp. Ther. 2006;319:1355-65. [PubMed ID: 16988055].
-
52.
Koschmieder Jørgensen N, Petersen SF, Damgaard I, Schousboe A, Hoffmann EK. Increases in [Ca2+] i and changes in intracellular pH during chemical anoxia in mouse neocortical neurons in primary culture. J. Neurosci. Res. 1999;56:358-70. [PubMed ID: 10340744].
-
53.
Chan J, Greenberg DA. Effects of glucose on calcium channels in neural cells. Neurosci. Lett. 1991;121:34-6. [PubMed ID: 1708485].
-
54.
Yamada T, Kawahara K, Kosugi T, Tanaka M. Nitric oxide produced during sublethal ischemia is crucial for the preconditioning-induced down-regulation of glutamate transporter GLT-1 in neuron/astrocyte co-cultures. Neurochem. Res. 2006;31:49-56. [PubMed ID: 16474996].
-
55.
Varathan S, Shibuta S, Varathan V, Takemura M, Yonehara N, Mashimo T. Effects of deep hypothermia on nitric oxide‐induced cytotoxicity in primary cultures of cortical neurons. J. Neurosci. Res. 2003;72:613-21. [PubMed ID: 12749026].
-
56.
Whiteman M, Armstrong JS, Cheung NS, Siau JL, Rose P, Schantz JT, Jones DP, Halliwell B. Peroxynitrite mediates calcium-dependent mitochondrial dysfunction and cell death via activation of calpains. FASEB J. 2004;18:1395-7. [PubMed ID: 15240564].
-
57.
Olesen SP, Møller A, Mordvintcev P, Busse R, Mülsch A. Regional measurements of NO formed in vivo during brain ischemia. Acta Neurol. Scand. 2009;95:219-24. [PubMed ID: 9150812].
-
58.
Kowara R, Moraleja KL, Chakravarthy B. Involvement of nitric oxide synthase and ROS-mediated activation of L-type voltage-gated Ca2+ channels in NMDA-induced DPYSL3 degradation. Brain Res. 2006;1119:40-9. [PubMed ID: 16987501].
-
59.
Moncada C, Lekieffre D, Arvin B, Meldrum B. Effect of NO synthase inhibition on NMDA-and ischaemia-induced hippocampal lesions. Neuroreport. 1992;3:530-2. [PubMed ID: 1382661].