Abstract
Keywords
Telmisartan Solid dispersions Organic Solvent-free In-vitro characterization Oral bioavailability
Introduction
Telmisartan (TEL), is a new angiotensin II type 1 receptor blocker (ARB) useful in the treatment of hypertension, heart diseases, heart attack and bladder diseases (1). However, as a BCS class II drug, the solubility of TEL is strongly pH-dependent, with maximum solubility observed at high and low pH, and it is poorly soluble in the range of pH 3–9 (2). Although TEL is rapidly absorbed in the stomach after oral administration, its poor solubility in the intestinal sites can make its absorption rate limited, and further results in low bioavailability and large individual variation. Therefore, a new type of formulation that can improve the dissolution rate and oral bioavailability of TEL in the gastrointestinal tract will be desired.
A possible way of overcoming the challenges of poor absorption rate caused by the low solubility of poorly soluble drug is to alter the physical properties of the drug by preparation of solid dispersions (SDs) (3). The enhanced dissolution rates of drugs by formulating them into SDs can be attributed to the reduction in particle size, reduction in the crystallinity and an increase in the surface area (4). In addition, no energy is required to break up the crystal lattice of a drug during dissolution process and drug solubility and wettability may be increased by surrounding hydrophilic carriers (5).
Various methods have been reported to prepare SDs, such as spray drying, co-grinding, hot melt extrusion and solvent wetting methods (6, 7). However, these methods have some limitations such as poor miscibility between the drug and its carriers, degradation of drug due to the requirement of relatively high preparation temperatures, and toxicity and ecological problems associated with the use of organic solvents (8). Lyophilization is an effective method which can keep the physicochemical stability of a drug during the manufacturing process. Although considerable efforts have been made for the preparation of SDs by lyophilization (9, 10), few studies have been conducted to prepare SDs without using any organic solvents.
In order to improve the low solubility of TEL, numerous attempts have been made by researchers (4, 11, 12, 13, 14). Tran et al. successfully prepared polyethylene glycol 6000 (PEG 6000)-based SDs loading TEL and alkalizers. They demonstrated that the alkalizers in PEG 6000-based SDs could synergistically enhance the dissolution of TEL not only by modulating pHM but also by changing drug crystallinity to an amorphous form (4). Patel et al. has recently developed a novel TEL-loaded surface solid dispersion (SSD) using poloxamer 407 and PEG 6000. The in-vitro study showed that the solubility and dissolution of TEL were significantly increased in form of SSD as compared to pure drug (11). More recently, Marasini et al. has also designed pH modulated SDs using polyvinylpyrrolidone K30 (PVP K30) and alkalizers by a spray-drying method. The developed SDs exhibited marked improvement of dissolution rate and oral bioavailability of TEL (12). However, it could be found in these studies that different organic solvents had been routinely used to dissolve the drug during the formulation and preparation process.
In this study, organic solvent-free solid dispersions (OSF-SDs) containing TEL were prepared with PVP K30 and PEG 6000 as hydrophilic polymers, NaOH as an alkalizer, and poloxamer 188 as a surfactant by a lyophilization method. In-vitro dissolution rate and physicochemical properties of OSF-SDs were investigated and in-vivo oral bioavailability of OSF-SDs was also evaluated by using TEL as a reference.
Experimental
Materials
Telmisartan (TEL) was obtained from Eurapharm, Inc. (Suwon, South Korea). Polyvinylpyrrolidone K30 (PVP K30, Povidone® K30) was supplied by BASF (Shanghai, China) and the polyethylene glycol 6000 (PEG 6000) was purchased from Shinyo (Osaka, Japan). Sodium hydroxide (NaOH) was purchased from Sinopharm Chemical Reagent Co. (Shanghai, China). Poloxamer 188 (Lutrol® F68) was kindly provided by Shanghai Chineway Pharm. Tech. Co. (Shanghai, China). All other reagents and chemicals were of analytical grade and used without further purification.
Preparation of organic solvent-free solid dispersions (OSF- SDs)
The OSF-SDs containing TEL were prepared by a lyophilization method as reported in the literature (15). The detailed formulation compositions are shown in Table 1. Briefly, NaOH was dissolved in distilled water and formed an alkaline solution. TEL and PVP K30 (or PEG 6000) were accurately weighed and dissolved in the alkaline solution with a continuous stirring for the preparation of OSF-SD1 and OSF-SD3. On the other hand, TEL, PVP K30 (or PEG 6000) and poloxamer 188 were also dissolved in the upper alkaline solution for the preparation of OSF-SD2 and OSF-SD4. The resulting solutions were then frozen at -70 °C and lyophilized using a lyophilizer (Christ Alpha 1-4, Germany). After the lyophilization, the solid masses were grinded and sieved to obtain a particle size fraction of 125–500µm.
Formulation compositions of OSF-SDs containing TEL
Formulation | TEL | PVP K30 (g) | PEG 6000 (g) | Poloxamer | NaOH | Distilled water |
---|---|---|---|---|---|---|
OSF-SD1 | 0.8 | 2.4 | - | - | 0.08 | 4 |
OSF-SD2 | 0.8 | 2.4 | - | 0.16 | 0.08 | 4 |
OSF-SD3 | 0.8 | - | 2.4 | - | 0.08 | 4 |
OSF-SD4 | 0.8 | - | 2.4 | 0.16 | 0.08 | 4 |
Analysis of drug content
The OSF-SDs equivalent to 80 mg of TEL loading were accurately weighted and dissolved in 100 mL of methanol. One milliliter of above solution was diluted into 10 mL, and then filtered through 0.45 µm Millipore filters. The TEL in filtrate was analyzed by a HPLC method described below.
Scanning electron microscopy (SEM)
The OSF-SDs were detected by S-4700 (Hitachi, Tokyo, Japan) scanning electron microscope in high vacuum mode. Samples were coated with gold for 180 s using a JEOL JFC-1100 sputter coater (Jeol, Tokyo, Japan) under argon atmosphere and then imaged at ambient temperature at 15 kV and observed at magnifications of 100×.
Differential scanning calorimetry (DSC)
DSC measurements were performed using a differential scanning calorimeter (Model 2010, TA Instruments, USA). Accurately weighed samples (20-50 mg) including raw material, excipients, OSF-SDs and their physical mixtures (PMs) were sealed in aluminum pans. An empty aluminum pan was used as reference. The samples were heated at a scanning rate of 10 °C/min from 50 to 300 °C under a dry nitrogen gas purge.
X-ray powder diffractometry (XRD)
The XRD patterns of the raw material, excipients, OSF-SDs and their PMs were recorded by using an X-ray diffractometer (MERCURY CCD, Japan) with tube anode Cu over the interval 5–60°/2θ. The scanning rate was adjusted to 2°/min.
Fourier transform-infrared (FT-IR) spectroscopy
The spectra of the samples including the raw material, excipients, OSF-SDs and their PMs were characterized using a FTIR spectrophotometer (Model Excaliber Series UMA-500, Bio-Rad, USA). KBr pellets were prepared by gently mixing 1 mg of the sample with 200 mg KBr. The wavelength ranged from 400 to 4,000 cm-1 with a resolution of 2 cm-1.
Dissolution studies
Dissolution studies were performed for both TEL bulk powder and OSF-SDs equivalent to 80 mg of TEL loading according to the USP dissolution I basket method at a rotation speed of 75 rpm in the 900 mL of pH 6.8 phosphate buffer solution at 37±0.5 °C (16). At predetermined intervals, 5 mL of the samples was withdrawn and filtered through a membrane filter (0.45μm). The concentration of TEL was assayed by a HPLC method described below. All experiments were performed in triplicate. In addition, dissolution studies were also carried out in the media with different pH values (pH1.2, pH4.5, pH7.4) and water.
High performance liquid chromatography (HPLC) analysis
TEL was analyzed using a HPLC system (Shimadzu, LC-20A, Japan) consisting of a LC-20AD pump and a SPD-M20A detector. Analysis was carried out on a Phenomenex C18 column (250 × 4.6 mm, 5 μm). The mobile phase consisted of a 60 : 40 (%v/v, pH3.7) mixture of acetonitrile and 10 mM potassium dihydrogen phosphate. The flow rate was 1.0 mL/min and the column temperature was maintained at 35 °C. The injection volume was 20 μL and the detection wavelength was 296 nm (4).
Bioavailability study
Animal study
Before beginning any experiment, we obtained approval for this study from the Institutional Review Board on Animal Research and Ethics Committees of Soochow University. Eighteen male SD rats weighing 260–320 g were randomly divided into three treatment groups, each consists of six rats. The rats were fasted over 12 h prior to the experiments. A polyethylene cannula (inner diameter, 0.58 mm; outer diameter 0.96 mm) was surgically introduced into the left femoral artery of rat under ether anesthesia to obtain blood samples at the various sampling times. The mini capsules containing TEL bulk powder, OSF-SD3 and OSF-SD4 were administered orally at a dose equivalent to 3.6mg (TEL)/kg body weight using an oral sonde to three groups respectively. Approximately 0.5 mL of blood sample was collected in a heparinized tube using an indwelling cannula at 0.15, 0.5, 1, 2, 4, 6, 8, 12 and 24 h, and then centrifuged at 1500 × g for 10 min to collect the plasma samples. The plasma samples were stored in a freezer at −40 °C until being analyzed by HPLC (17).
Preparation of plasma sample
Twenty microliters of itraconazole (4 μg/mL) as an internal standard were added to 200 μL of each plasma sample and voltexed for 5 s. Fifty microliters of 10 % phosphoric acid and 1 mL of diethyl ether were added to the mixture and then agitated for 5 min using a vortex mixer. Thereafter, the mixture was centrifuged at 1500 × g for 10 min. The organic phase was transferred into a tube and evaporated under a gentle stream of nitrogen. The residue was then reconstituted with 50 μL of methanol and 20 μL of the resulting solution was injected into the HPLC system.
HPLC analysis for plasma samples
Chromatographic separation was performed at a flow rate of 1.0 mL/min, at a wavelength of 254 nm, using a Phenomenex C18 (4.6 × 250 mm, 5 μm) column. The column temperature was maintained at 35 °C. The mobile phase was acetonitrile: 0.01mol/L phosphate buffer (60:40, v/v, pH 3.7) (4).
Data analysis
Pharmacokinetic parameters of TEL were calculated using non-compartmental methods. The maximum plasma concentration (Cmax) and the time to reach the Cmax (Tmax) were read directly from the plasma concentration–time profiles of TEL. The areas under the plasma concentration–time curve from zero to 24 h (AUC0–24h) were calculated using the classical trapezoidal method. All data are presented as mean ± standard deviation. The statistical significance of the differences was performed using an analysis of variance (ANOVA) test and a p value < 0.05 or 0.01 was considered significant.
Results and Discussion
Preparation and characterization of OSF-SDs
In this study, four different types of OSF-SDs containing TEL were prepared by using a lyophilization method similar to the method in the literature (15). PEG 6000 and PVP K30 were chosen because they have been demonstrated to have significant inhibition effects on the re-crystallization of amorphously dispersed drugs in SDs, and they can render an increase in solubilities and dissolution rates of the drugs (18, 19). The incorporation of poloxamer 188 in SDs has also proven to be an effective way to facilitate the solubilization and stabilization of poorly soluble drugs (20, 21).
In order to avoid using organic solvents during the preparation of SDs, sodium hydroxide (NaOH) was used as an alkalizer to increase the solubility of TEL in polymeric solution for a lyophilization. In our previous study, TEL was absolutely dissolved in a polymeric solution alkalized with NaOH. However, it could not be formed a clear solution when incorporated with other alkalizers such as MgO, NaHCO3, Na2CO3 and Na2HPO4 at a given concentration. Therefore, NaOH was routinely used as an alkalizer in this study.
The drug contents of prepared SDs were analyzed by a HPLC system. As shown in Table 2, the content of TEL in OSF-SD1, OSF-SD2, OSF-SD3 and OSF-SD4 approached almost 100%, indicating that the formulation composition and preparing process had almost no effect on the drug content of OSF-SDs. The SEM images of OSF-SDs are also shown in Figure 1. Both PVP K30-based SD2 (Figure 1A) and PEG 6000-based SD4 (Figure 1B) were formed as regular shapes after grinding.
Content analysis of TEL in OSF-SDs (mean ±SD, n=3
Formulation code | Drug content (%) |
---|---|
OSF-SD1 | 98.58 ± 1.18 |
OSF-SD2 | 99.98 ± 1.97 |
OSF-SD3 | 103.51 ± 2.84 |
OSF-SD4 | 97.39 ± 5.22 |
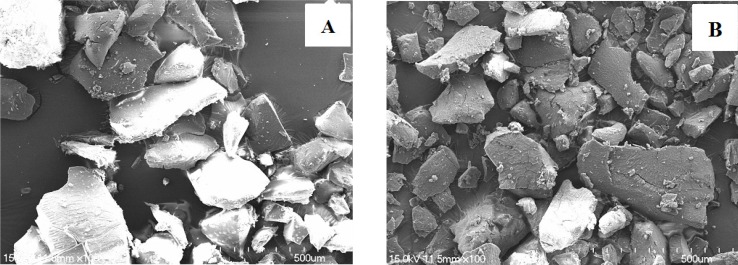
The solid state of the drugs in the OSF-SDs was characterized using DSC, XRD and FT-IR. The DSC thermograms of TEL, excipients, OSF-SDs and their PMs are shown in Figure 2. The DSC curve of TEL (Figure 2A) exhibited a distinct single endothermic peak at 267.22 °C, which corresponds to its intrinsic melting point. No melting drug peak was observed in both PVP K30 and PEG 6000-based OSF-SDs (Figure 2E-H), while a distinct peak was still remained in the thermograms of PVP K30-based PMs (Figure 2I and J). The reason why drug peak disappeared in PEG 6000-based PMs (Figure 2L and M) could be attributed to the low melting point of PEG 6000, resulting in dissolution of TEL in an eutectic mixture of drug and carriers during DSC analysis. The DSC results implied the distortion of the crystalline structure of the drug in the OSF-SDs (22). The transformation of crystalline structure of drug to amorphous or partially amorphous state may enhance drug dissolution rate from the OSF-SDs (23).
![DSC thermograms of TEL (A), PVP K30 (B), PEG 6000 (C), NaOH (D), poloxamer 188 (E), OSF-SDs [OSF-SD1 (F), OSF-SD2 (G), OSF-SD3 (H), OSF-SD4 (I)] and their physical mixtures [PM1 (J), PM2 (K), PM3 (L), PM4 (M)].](https://services.brieflands.com/cdn/serve//315db/0e9a8f9a182053467b144148d6f5b02f7cdca005/ijpr-15-385-g002.jpg)
The crystallinity of the drug in SDs was further investigated by using XRD. As shown in Figure 3A, the characteristic reflections of TEL can be detected at 2θ positions of 6.8°, 14.3° and 22.3°. The characteristic crystalline reflections of PEG 6000, NaOH and poloxamer 188 can also be observed at different 2θ positions as shown in Figure 3C-E, while PVP K30 (Figure 3B) was amorphous form lacking crystalline characteristics. Some crystalline signals of the drug were still detectable in the PMs of drug and excipients (Figure 3J-M). However, compared with those of the PMs, the distinctive crystalline peaks of the drug disappeared in OSF-SDs (Figure 3F-I). It is well known that the lack of distinctive XRD reflections of a drug in the SDs demonstrates the drug was highly dispersed in SDs. Moreover, a large reduction in crystallinity of the drug indicates the drug is in an amorphous state, which can result in improved dissolution rate of the drug (11, 12).
![XRD patterns of TEL (A), PVP K30 (B), PEG 6000 (C), NaOH (D), poloxamer 188 (E), OSF-SDs [OSF-SD1 (F), OSF-SD2 (G), OSF-SD3 (H), OSF-SD4 (I)] and their physical mixtures [PM1 (J), PM2 (K), PM3 (L), PM4 (M)].](https://services.brieflands.com/cdn/serve//315db/2354e06d1405c7778b18a18d056208179a88c71b/ijpr-15-385-g003.jpg)
FT-IR was carried out to further characterize possible interactions between the drug and the carriers in the OSF-SDs. The structural changes and the lack of crystal structure can lead to the changes in bonding between functional groups, which can be observed by FT-IR spectroscopy. The FT-IR spectrum of TEL (Figure 4A) showed a distinct absorption band for the C=C skeleton vibration of benzene at 1579 cm-1, the carbonyl group C=O at 1690 cm-1 and the O–H band at 3433 cm-1 (24, 25). Neither form changes nor shifts were observed for both C=C vibration band and O–H band of TEL in OSF-SDs (Figure 4F-I). Interestingly, the peak of carbonyl group of TEL in PEG 6000-based OSF-SD3 (Figure 4H) and OSF-SD4 (Figure 4I) disappeared. However, the changes of C=O band in PVP K30-based OSF-SD1 (Figure 4F) and OSF-SD2 (Figure 4G) could not be observed because the corresponding peak was overlapped by the peaks of carriers. It has been described that a lowering of the frequency of the carbonyl stretching band from carboxylic acid is typically indicative of strong hydrogen bonding interactions (26), indicating good miscibility between the drug and the carriers (27).
![FT-IR spectra of TEL (A), PVP K30 (B), PEG 6000 (C), NaOH (D), poloxamer 188 (E) and OSF-SDs [OSF-SD1 (F), OSF-SD2 (G), OSF-SD3 (H) and OSF-SD4 (I)].](https://services.brieflands.com/cdn/serve//315db/7f30b521744fb138c258eab7c4956ff41dfe7ad6/ijpr-15-385-g004.jpg)
Dissolution studies
In order to evaluate the drug release from OSF-SDs, the dissolution study was carried out under the sink condition using a basket method. As shown in Figure 5, in pH 7.4 buffer solution, both PEG 6000-based (OSF-SD3 and OSF-SD4) and PVP K30-based SDs (OSF-SD1 and OSF-SD2) showed remarkably higher dissolution rates than TEL bulk power. Especially, the dissolution rates of PEG 6000-based SDs (OSF-SD3 and OSF-SD4) were higher than those of PVP K30-based SDs (OSF-SD1 and OSF-SD2). Furthermore, the formulations containing poloxamer 188 (OSF-SD2 and OSF-SD4) exhibited higher initial drug release than OSF-SD1 and OSF-SD3. These could be attributed to the excellent hydrophilicity and dispersibility of hydrophilic carriers in water (especially for PEG 6000), and further the solubilization and pH modulation effects by poloxamer 188 and NaOH incorporated in OSF-SDs (28). As shown in Figure 6, the OSF-SD4 exhibited different dissolution profiles in different pH media, with the highest rate at pH 7.4 and the lowest rate at pH4.0. This is most likely due to the fact that the solubility of TEL is strongly pH-dependent as described in the literature (2).
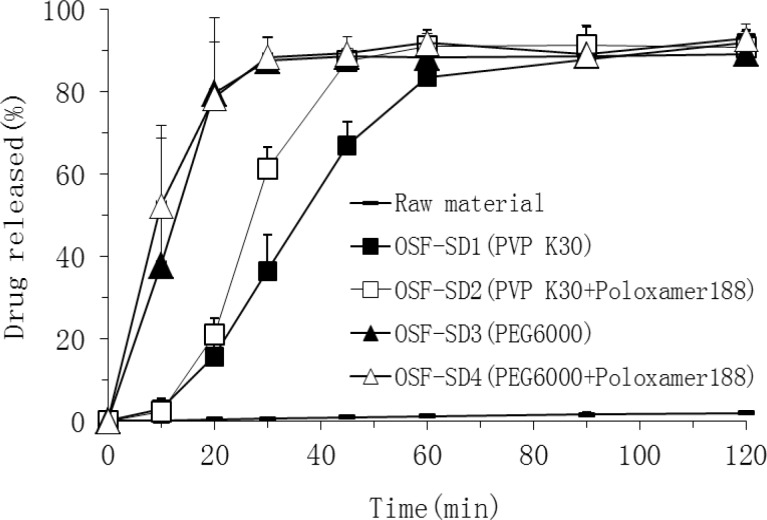
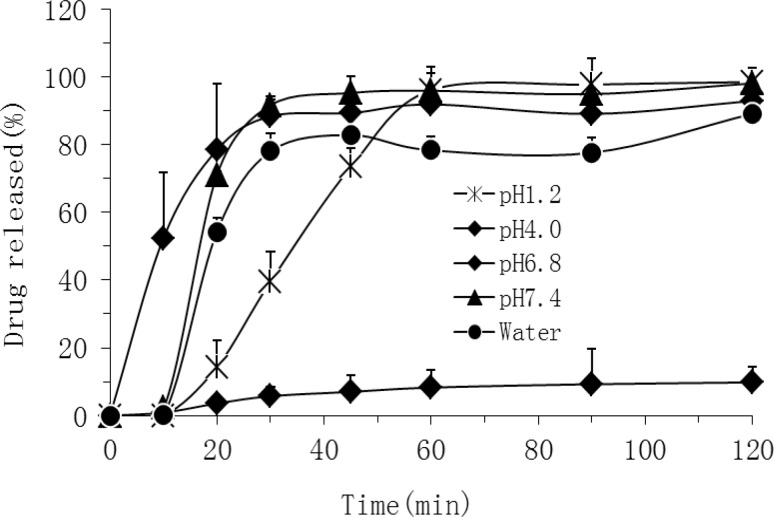
The mechanisms of enhanced dissolution rate of TEL from OSF-SDs can be ascribed to several factors such as the reduction of particle size, reduction in interfacial tension between hydrophobic drug and dissolution medium, improved wettability and micro-environmental solubility of the drug in SDs (29). Moreover, the lack of crystallinity of the drug, as discussed above, could be considered as an important factor in enhancement of the dissolution rate (30).
Bioavailability study
The oral bioavailability and pharmacokinetic parameters of PEG 6000-based SDs (OSF-SD3 and OSF-SD4) were evaluated by using TEL bulk powder as a reference after oral administration to rats. In this animal study, both OSF-SD3 and OSF-SD4 were chosen because they showed relatively higher dissolution rates than other formulations. Meanwhile, the effect of surfactant (poloxamer 188) on the absorption of TEL in SDs could also be investigated. As shown in Figure 7, the mean plasma concentrations of the drug from OSF-SDs were remarkably higher than that from the TEL bulk powder. Especially, the OSF-SD4 containing poloxamer 188 showed the highest plasma concentration-time curve among all formulations. The main pharmacokinetic parameters are also presented in Table 3. The Cmax of OSF-SD3 and OSF-SD4 increased about 2.2 and 2.5 folds compared with that of TEL bulk powder (584 and 670 ng/mL versus 268 ng/mL, p < 0.01), respectively. Similarly, the AUC0-24 of them increased about 3.2 and 4.7 folds compared with that of the reference (4417 and 6375 ng·h/mL versus 1360 ng·h/mL, p < 0.01), respectively. It could also found that the OSF-SD4 gave a significantly higher Cmax and AUC0-24 than OSF-SD3 (p < 0.05 and p < 0.01, respectively). This could be attributed to the positive effect of poloxamer 188 on the solubility and gastrointestinal permeability of the drug (10). Although OSF-SDs showed a relatively longer Tmax than the reference, there was no statistically significant difference for Tmax between each group. Overall, the OSF-SDs exhibited remarkably higher oral absorption than the TEL bulk powder. This might to be due to the improved solubility, wettabiliy and dipersibility produced by the hydrophilic polymer and alkalizer, as well as the absorption enhancement of drug molecule by the surfactant (4, 19, 20, 26).
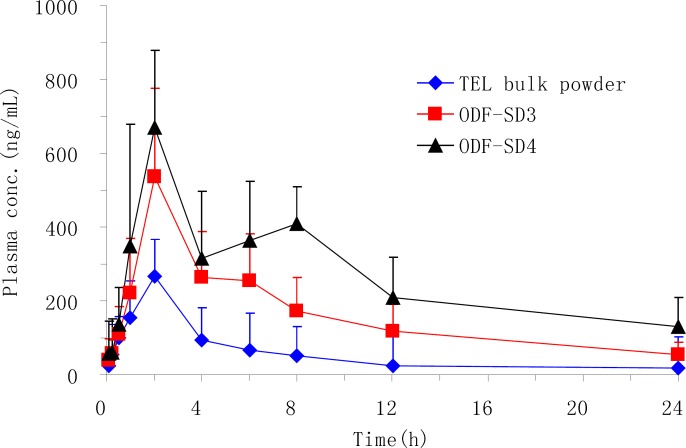
Pharmacokinetic parameters of TEL following a single oral administration of TEL bulk powder, OSF-SD3 and OSF-SD4 in rats (mean ±SD, n=6
Conclusions
In this study, OSF-SDs were successfully prepared with PVP K30 and PEG 6000 as hydrophilic carriers, NaOH as an alkalizer, and poloxamer 188 as a surfactant by a lyophilization method without using any organic solvents. A significant enhancement of the dissolution rate of TEL, a poorly water-soluble model drug, was achieved in OSF-SDs. The results of DSC, XRD and FT-IR suggest that TEL was molecularly dispersed in the carriers, and intermolecular hydrogen bonding between the drug and its carriers were formed in SDs. An enhanced oral bioavailability of TEL was achieved by formulating it into OSF-SD. This study demonstrates organic solvent free lyophilization method can be a promising method to formulate TEL into solid dispersion and improve its systemic uptake.
Acknowledgements
References
-
1.
Kumar AS, Ghosh S, Mehta GN. Efficient and improved synthesis of Telmisartan. Beilstein J Org Chem. 2010;25:1-5.
-
2.
Wienen W, Entzeroth M, van Meel JCA, Stangier J, Busch U, Ebner T, Schmid J, Lehmann H, Matzek K, Kempthorne-Rawson J, Gladigau V, Hauel NH. A review on telmisartan: A novel, long-acting angiotensin II-receptor antagonist. Cardiovasc Drug Rev. 2000;18:127-154.
-
3.
Castro SG, Bruni SS, Lanusse CE, Allemandi DA, Palma SD. Improved albendazole dissolution rate in pluronic 188 solid dispersions. AAPS PharmSciTech. 2010;11:1518-1524. [PubMed ID: 20945166].
-
4.
Tran PHL, Tran HTT, Lee BJ. Modulation of microenvironmental pH and crystallinity of ionizable TEL using alkalizers in solid dispersions for controlled release. J Control Rel. 2008;129:59-65.
-
5.
Van den Mooter G, Augustijns P, Blaton N, Kinget R. Physico-chemical characterization of solid dispersions of temazepam with polyethylene glycol 6000 and PVP K30. Int J Pharm. 1998;164:67-80.
-
6.
Janssens S, Roberts C, Smith EF, Van den Mooter G. Physical stability of ternary solid dispersions of itraconazole in polyethylene glycol 6000/ hydroxypropylmethylcellulose 2910 E5 blend. 2008 Int J Pharm;355:100-107.
-
7.
Xie YM, Xie P, Song X, Tang XH, Song H. Preparation of esomeprazole zinc solid dispersion and study on its pharmacokinetics. Int J Pharm. 2008;360:53-57. [PubMed ID: 18495389].
-
8.
Kim EJ, Chun MK, Jang JS, Lee IH, Lee KR, Choi HK. Preparation of a solid dispersion of felodipine using a solvent wetting method. Eur J Pharm Biopharm. 2006;64:200-205. [PubMed ID: 16750355].
-
9.
Lee J, Cheng Y. Critical freezing rate in freeze drying nanocrystal dispersions. J Control Rel. 2006;111:185-192.
-
10.
He XQ, Pei LX, Tong HHY, Zheng Y. Comparison of spray freeze drying and the solvent evaporation method for preparing solid dispersions of baicalein with Pluronic F68 to improve dissolution and oral bioavailability. AAPS PharmSciTech. 2011;12:104-113. [PubMed ID: 21181514].
-
11.
Patel B, Parikh RH, Swarnkar D. Enhancement of dissolution of telmisartan through use of solid dispersion technique-surface solid dispersion. J Pharm Bioallied Sci. 2012;4:S64-68. [PubMed ID: 23066211].
-
12.
Marasini N, Tran TH, Poudel BK, Cho HJ, Choi YK, Chi SC, Choi HG, Yong CS, Kim JO. Fabrication and evaluation of pH-modulated solid dispersion for telmisartan by spray-drying technique. Int. J. Pharm. 2013;441:424-432. [PubMed ID: 23174408].
-
13.
Zhong L, Zhu XY, Luo XF, Su WK. Dissolution properties and physical characterization of telmisartan–chitosan solid dispersions prepared by mechanochemical activation. AAPS PharmSciTech. 2013;14:541-550. [PubMed ID: 23430728].
-
14.
Park J, Cho W, Cha KH, Ahn J, Han K, Hwang SJ. Solubilization of the poorly water soluble drug, telmisartan, using supercritical anti-solvent (SAS) process. Int J Pharm. 2013;441:50-55. [PubMed ID: 23262425].
-
15.
García-Rodriguez JJ, de la Torre-Iglesias PM, Vegas-Sánchez MC, Torrado-Durán S, Bolás-Fernández F, Torrado-Santiago S. Changed crystallinity of mebendazole solid dispersion: improved anthelmintic activity. Int J Pharm. 2011;403:23-28. [PubMed ID: 20934497].
-
16.
Varghese SJ, Johny SK, David Paul D, Ravi TK. In-vitro interaction study of retinoic acid isomers with telmisartan and amlodipine by equilibrium dialysis method using UV spectroscopy Spectrochim. Acta: Part A. 2011;79:384-387.
-
17.
Cao QR, Kim TW, Choi JS, Lee BJ. Circadian variations in the pharmacokinetics, tissue distribution and urinary excretion of nifedipine after a single oral administration to rats. Biopharm. Drug Dispos. 2005;26:427-437. [PubMed ID: 16217814].
-
18.
Heo MY, Piao ZZ, Kim TW, Cao QR, Kim AR, Lee BJ. Effect of solubilizing and microemulsifying excipients in polyethylene glycol 6000 solid dispersion on enhanced dissolution and bioavailability of ketoconazole. Arch Pharm Res. 2005;28:604-611. [PubMed ID: 15974450].
-
19.
Karavas E, Georgarakis E, Sigalas MP, Avgoustakis K, Bikiaris D. Investigation of the release mechanism of a sparingly water-soluble drug from solid dispersions in hydrophilic carriers based on physical state of drug, particle size distribution and drug–polymer interactions. Eur J Pharm Biopharm. 2007;66:334-347. [PubMed ID: 17267194].
-
20.
Newa M, Bhandari KH, Li DX, Kwon TH, Kim JA, Yoo BK, Woo JS, Lyoo WS, Yong CS, Choi HG. Preparation, characterization and in-vivo evaluation of ibuprofen binary solid dispersions with poloxamer 188. Int J Pharm. 2007;243:228-237. [PubMed ID: 17597315].
-
21.
Mehanna MM, Motawaa AM, Samaha MW. In sight into tadalafil-block copolymer binary solid dispersion: Mechanistic investigation of dissolution enhancement. Int J Pharm. 2010;402:78-88. [PubMed ID: 20933589].
-
22.
Srinarong P, Faber JH, Visser MR, Hinrichs WLJ, Frijlink HW. Strongly enhanced dissolution rate of fenofibrate solid dispersion tablets by incorporation of superdisintegrants. Eur J Pharm Biopharm. 2009;73:154-161. [PubMed ID: 19465121].
-
23.
Yang J, Grey K, Doney J. An improved kinetics approach to describe the physical stability of amorphous solid dispersions. Int J Pharm. 2010;384:24-31. [PubMed ID: 19786081].
-
24.
Zhang YZ, Zhi ZZ, Jiang TY, Zhang JH, Wang ZY, Wang SL. Spherical mesoporous silica nanoparticles for loading and release of the poorly water-soluble drug telmisartan. J Control Rel. 2010;145:257-263.
-
25.
Zhang YZ, Jiang TY, Zhang Q, Wang SL. Inclusion of telmisartan in mesocellular foam nanoparticles: Drug loading and release property. Eur J Pharm Bopharm. 2010;76:17-23.
-
26.
Ha NS, Tran TTD, Tran PHL, Park JB, Lee BJ. Dissolution-enhancing mechanism of alkalizers in poloxamer-based solid dispersions and physical mixtures containing poorly water-soluble valsartan. Chem Pharm Bull. 2011;59:844-850. [PubMed ID: 21720034].
-
27.
Papadimitriou SA, Barmpalexis P, Karavas E, Bikiaris DN. Optimizing the ability of PVP/PEG mixtures to be used as appropriate carriers for the preparation of drug solid dispersions by melt mixing technique using artificial neural networks: I. Eur J Pharm Biopharm. 2012;82:175-186. [PubMed ID: 22732266].
-
28.
Cao QR, Liu Y, Xu WJ, Lee BJ, Yang MS, Cui JH. Enhanced oral bioavailability of novel mucoadhesive pellets containing valsartan prepared by a dry powder-coating technique. Int J Pharm. 2012;434:325-333. [PubMed ID: 22688251].
-
29.
Bley H, Fussnegger B, Bodmeier R. Characterization and stability of solid dispersions based on PEG/polymer blends. Int J Pharm. 2010;390:165-173. [PubMed ID: 20132875].
-
30.
Savolainen M, Kogermann K, Heinz A, Aaltonen J, Peltonen L, Strachan C, Yliruusi J. Better understanding of dissolution behaviour of amorphous drugs by in situ solid-state analysis using Raman spectroscopy. Eur J Pharm Biopharm. 2009;71:71-79. [PubMed ID: 18590816].