Abstract
Keywords
Matrine HCC cell proliferation Aapoptosis P53 Myosin light chain kinase
Introduction
Hepatocellular carcinoma (HCC), the most common type of liver cancer, is the fifth most common malignant tumor type worldwide and the second leading cause of cancer-related deaths (1). The incidence and mortality of HCC are increasing rapidly every year (2). The proliferation of HCC cells is the primary cause of death. However, the mechanism of this proliferation is complex and poorly understood. Tumor cells continuously integrate external stress signals and determine if the cell should die or survive. These determinations are made by a wide range of signaling events that are controlled by kinases. Myosin II activation is essential for stress fiber and focal adhesion formation, and is implicated in integrin-mediated signaling events. Integrin ligation by the extracellular matrix (ECM) plays a crucial role in organizing the cytoskeleton (3), and the loss of substrate attachment is known to induce a type of apoptosis called anoikis (apoptosis upon loss of anchorage detachment from ECM) (4).Therefore, interactions between cells and the ECM play an important role in cell survival (5).The actin-myosin II interaction in smooth muscle and nonmuscle cells is regulated by the phosphorylation of serine 19 of the 20-kDa light chain of myosin II (MLC II )(6). This reaction is catalyzed by myosin light chain kinase (MLCK). MLCK, also known as calcium (Ca2+)/calmodulin-dependent protein kinase, plays a major role in the formation and stability of a cell’s cytoskeleton. This phosphorylation activates myosin, causing it to interact with actin (7). The disintegration and reformation of the cytoskeleton is very important for many cell reactions. The proliferation of tumor cells is partially influenced by the cytoskeleton (8). MLCK, when activated or overexpressed, has been found to contribute to the proliferation of tumor cells (9, 10). Hyper phosphorylated MLC II induces stress fiber formation and integrin clustering that link cell surface cytoskeletal proteins such as focal adhesion kinase (FAK) to actin (11, 12). FAK is a member of a focal adhesion family that mediates integrin-mediated signal transduction associated with a variety of cellular functions, including cellular adhesion, proliferation and migration (13). Integrins contribute to signal transduction from the extracellular environment to the intracellular network mediated by integrin-activated signaling molecules, such as FAK. The tumor suppressor p53 is a downstream signaling pathway to FAK, which regulates cell death pathway (14). There is some evidence suggesting that Bax, a proapoptotic member of the Bcl-2 family of cell death-regulating genes, is involved in p53- induced apoptosis. One set of molecules that appear to be modulated by the presence of Bax are the caspases (15). Caspase-3, one of a family of caspase proteases implicated in the commitment and execution of apoptotic cell death, exists as a proenzymes(35kDa)until cleaved in response to active apoptotic stimuli (19kDa and 17kDa) (16).
Matrine, a natural alkaloid component derived from a species of Leguminosae plant (Sophora flavescens Ait.), has been found to significantly inhibit the proliferation and migration of various types of tumor cells (17-22). In-vitro studies have demonstrated that matrine can promote the apoptosis of liver tumor cells and inhibit their proliferation (23-26). In-vivo, matrine has been found to prevent and treat the occurrence of diethyl nitrosamine (DENA)-induced HCC as well as the proliferation of liver tumor cells (27, 28). However, the mechanism by which matrine inhibits the proliferation of primary HCC cells and promotes the apoptosis of HCC cells, both in-vivo and in-vitro, remains unclear. In this study, we used a rat model of HCC to investigate the inhibitory effects of matrine on the proliferation of HCC cells. We also examined if matrine induced apoptosis in HCC cells via the down-regulation of MLCK overexpression and the activation of the P53 pathway and if matrine can inhibit the proliferation of HCC cells in-vivo.
Experimental
Animals and chemicals
Seventy Sprague-Dawley rats, weighing 70.3 ± 6.2 g, were purchased from the Animal Center of Changlinhe Pharmaceutic Co., Ltd. (Anhui, China). All of the rats were housed in cages under a 12 h light-dark cycle and fed a standard laboratory rat diet with water ad libitum. All procedures were conducted in accordance with the P. R. China legislation under No. 8910M047 on the use and care of laboratory animals and with the guidelines established by the Institute for Experimental Animals of Anhui Science and Technology University and were approved by the university committee for animal experiments. Matrine (concentration ≥ 99%) was obtained from the National Institutes for Food and Drug Control of Beijing, China. DENA was purchased from Sigma Chemical Co. (Beijing, China). A quantitative determination kit for α-fetoprotein (AFP) was purchased from Y-Y Chemical Reagents Co., Ltd. (Shanghai, China). Apoptosis detection and immunohistochemistry kits were purchased from Wuhan Boster Biological Technology, Ltd. (Hubei, China). A bicinchoninic acid (BCA) protein assay kit was purchased from Sangon Biotech Co., Ltd (Shanghai, China). Anti-procaspase-3 and anti-cleaved-caspase-3 were purchased from Biosynthesis Biotechnology Co., Ltd (Beijing, China). Rabbit anti-rat monoclonal MLCK antibody IgG and horseradish peroxidase-labeled antibody IgG were purchased from Hangzhou Hua’an Biotechnology Co., Ltd. (Zhejiang, China). Beta-actin rabbit polyclonal antibody IgG was purchased from Shanghai Kexing Biotechnology Co., Ltd. (Shanghai, China). Chemiluminescent substrates were purchased from Cell Signaling Technology (Beverly, MA, USA).
Experimental design
The rats were acclimatized for one week and then randomly assigned to either a control (n=12) or HCC group (n=58). The rats in the control group were given access to tap water for the duration of the experiment, while the rats in the HCC group were given access to a 0.01% DENA solution for the first 10 weeks of the experiment and then tap water for the remainder (29). After 20 weeks, blood samples were collected, and the AFP concentration in the serum was determined. Rats with an AFP concentration of more than 200 µg/L were used for the HCC model. The rats in the control group all had an AFP concentration of less than 20 µg/L. Forty-eight HCC model rats were further randomized into four groups: one model group (n=12) and three matrine-treated groups (n=12 for each group). The rats in the matrine-treated groups—labeled as group-low (L), group-medium (M) and group-high (H) according to the dosage administered—were treated by intraperitoneal injections of matrine. The dosage of matrine for each group was as follows: group-L received 12.5 mg/kg body weight, group-M received 25 mg/kg body weight and group-H received 50 mg/kg body weight. The rats of the three matrine-treated groups were treated by intraperitoneal injections of matrine for six consecutive weeks from the twentieth week. For the 12 h before they were sacrificed, the rats were restricted from food but not water.
Tissue collection and ELISA analysis
After 26 weeks, the rats were weighed, sacrificed under anesthesia induced by 3% pentobarbital sodium, and blood samples were collected. The blood samples were centrifuged, and the serum was separated. The AFP concentration in the serum was quantitated using enzyme-linked immunosorbent assay (ELISA) methods and a rat AFP assay kit (Y-Y Chemical Reagents Co., Ltd. Shanghai, China) according to the manufacturer’s instructions. The experiment was repeated in triplicate. According to the method previously published by Bishayee et al. (30), the rats’ livers were excised and weighed, and the number of visible hepatocyte nodules was recorded. A portion of the liver tissue was fixed, dehydrated in graded ethanol and embedded in paraffin. Some liver tissue was then sectioned into thicknesses of 5 µm, and used for cell apoptosis and immunohistochemical analyses. The remaining liver tissue was either used for Western blot analysis or stored at -80 °C for further use.
TUNEL staining
The TUNEL (terminal transferase dUTP nick end labeling) staining was performed in order to determine the effect of matrine on apoptosis by using an in situ cell death detection kit (Boster, Wuhan, China) according to the manufacturer’s instructions. The detection was performed using peroxidase (POD) and diaminobenzidine (DAB) substrate (Boster, Wuhan, China). Positive control slides were included in the kit. Sections were then counterstained with hematoxylin for 3 min and rinsed under running water. The number of apoptotic cells in the tissues of control and matrine-treated rats was determined by counting the stained cells in 12 fields, each of which contained at least 50 cells under the light microscope with 400×magnification for each section. The cell count was performed by an investigator who was blinded to the experimental protocol. The apoptotic index (AI) was calculated as follows: AI = (number of apoptotic cells/total number of cells) × 100%.
Western blot analysis
The rat liver tissues without nodule were homogenized in cold RIPA lysis buffer (Sangon, Shanghai, China) and the protein concentrations were determined by BCA assay kits (Sangon, Shanghai, China). Equal amounts of protein were separated using SDS-PAGE gels for p53, Bax, and cleaved-caspase-3, respectively. Then the protein was transferred onto a polyvinylidene fluoride (PVDF) membrane. The PVDF membranes were then incubated with rabbit monoclonal antibodies. The membranes were blocked with 5% fat-free milk in PBS with 0.1% Tween 20 (PBST) for one hour at room temperature and then incubated with different primary antibodies including anti-β-actin (1:1000),anti-p53,anti-Bax and anti-cleaved-caspase-3 (1:1000) overnight at 4 °C. After washing the membrane 3 times with PBST, secondary antibody HRP-labeled goat-anti-rabbit IgG was added to the membrane according to the vendor’s recommendation (1:2000) and incubated for 1h at room temperature. The membrane was then washed again as described previously. The bound antibodies were detected by using an ECL western blotting kit (Sangon, Shanghai, China). The relative protein level of p53, Bax, and cleaved-caspase-3 was calculated relative to that of β-actin.
Protein levels of MLCK were analyzed by western blot as described above. SDS-PAGE gels and PVDF membrane were used for MLCK. The primary antibodies used were anti-MLCK (1:1000) and anti-β-actin(1:1000). The relative protein level of MLCK was calculated relative to that of β-actin.
Immunohistochemistry
Liver tissue sections were deparaffinized and hydrated according to the methods detailed in the immunohistochemistry kit. The sections were incubated with the rabbit anti-rat monoclonal MLCK antibody IgG (primary antibody, 1:600 dilution) and then with the immunohistochemistry kit (secondary antibody). Phosphate buffered saline (PBS) was used as a negative control, replacing the primary antibody. The sections were then treated with 3,3’-diaminobenzidine (DAB) and examined under a microscope for positive signals. In our analysis, positive signals were yellowish brown. For each rat, three different images (at a magnification of 400×) were taken using an image analysis system. The integral absorbance of immunostaining for MLCK-positive foci signals was measured.
Data analysis
All of the data are presented as the mean ± the standard deviation (mean ± S.D.) and was analyzed statistically using SPSS 17.0 software. The average of each sample was then compared using variance analysis and a multiple comparison method. The enhanced chemiluminescence (ECL) Western blot images were semi-quantitatively analyzed using Image-Pro Plus 6.0 software.
Results
The effect of matrine on the body weights, liver weights and liver weight to body weight ratios of rats
Table 1 shows that the rats in the model group has significantly lower body weights (P < 0.01) and higher liver weight-to-body weight ratios (P < 0.01) than the control group, indicating that substantial HCC cell proliferation occurs in the model group. The rats of groups-L, -M and -H had significantly higher body weights (P < 0.01), lower liver weights (P < 0.01) and lower liver weight-to-body weight ratios (P < 0.01) than the model group, potentially indicating matrine inhibits the proliferation of HCC cells.
The effects of matrine on the body weights, liver weights and liver weight to body weight ratios in rats (mean ± S.D.)
Group | n | Body weight(g) | Liver weight(g) | Liver(g)/Body(g) weight×100) |
---|---|---|---|---|
control | 12 | 393.41 ± 24.63 | 12.36 ± 1.26 | 3.21 ± 0.30 |
model | 12 | 272.50 ± 14.26 | 21.19 ± 2.37 | 7.78 ± 0.81 |
group-L | 12 | 302.75 ± 18.87* | 18.11 ± 2.07* | 6.01 ± 0.80* |
group-M | 12 | 319.17 ± 29.49* | 16.30 ± 1.73* | 5.16 ± 0.83* |
group-H | 12 | 324.67 ± 33.96* | 15.25 ± 1.52* | 4.75 ± 0.73* |
The effect of matrine on the number of hepatocyte nodules and the serum concentration of AFP in rats
No natural occurrence of HCC was found in the rats of the control group, as indicated by the lack of visible hepatocyte nodules in their livers (Table 2). The liver size of the rats in the model group was increased dramatically, all of the hepatic lobes were greyish white in color due to the presence of hepatocyte nodules. Some of the lobes had a spherical structure. From the tissue sections, we found that the hepatocyte nodules were HCC and cholangiocarcinoma of different grades. Compared with the rats in the model group, the rats in groups-L, -M and -H had relatively smooth liver surfaces and significantly fewer hepatocyte nodules (P < 0.01). This result corroborated the suggested inhibition effect of matrine on HCC cell proliferation in rats. Additionally, the AFP concentrations in the rats of the matrine-treated and model groups were significantly increased when compared with the control group, but the AFP concentrations in all three matrine-treated groups were significantly lower (P < 0.01) than in the model group.
The effects of matrine on the numbers of hepatocyte nodules and the serum AFP concentrations in rats (mean±S.D.)
The effectof matrine on HCC cellapoptosis
The cells of the positive control (a slice given in the apoptosis kit) were almost entirely apoptotic (Figure 1A). HCC cell apoptosis in the liver of the model rats (Figure 1C) was greatly inhibited compared to the normal control group (Figure 1B), though the other liver tissue cells continued to undergo programmed cell death. A larger number of apoptotic HCC cells were found in the liver of the matrine-treated rats (Figure 1D; 1E; 1F) than in those of the model group (Figure 1C). The plot in Figure 1 shows the calculated apoptotic indexes (AIs). The AI of the model liver tumor cells (14.3 ± 5.2) was significantly smaller (P < 0.05) than that of the normal tissue cells (31.7 ± 7.6). The AIs of the tumor cells in the livers of the matrine-treated rats (group-L: 36.7 ± 12.5) (P < 0.05) (group-M: 68.2 ± 18.6; group-H: 78.9 ± 15.6) (P < 0.01) were significantly greater than those of the model group.
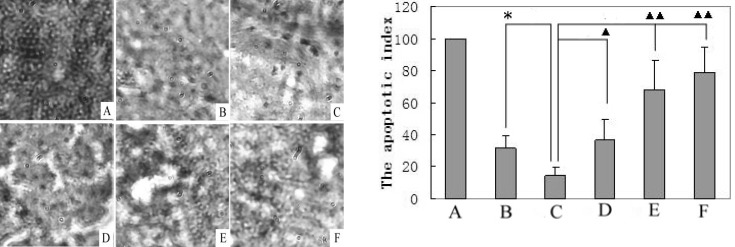
Matrine effected on activation of cleaved caspase-3 via modulation of p53 and Bax expression. We then investigated if the mechanism of matrine-induced liver tumor cell apoptosis through activation of cleaved capase-3 expression depends on modulation of the p53 and Bax pathway. Western blot analysis was used to test the effect of matrine on p53 and Bax proteins expression. Figure 2 indicated shows that matrine can significantly up-regulate p53 and Bax expression. At the same time, protein levels of β-actin, and cleaved caspase-3 were detected in the livers of HCC model and matrine-treated rats (Figure 3). As can be seen in Figure 3, treatment with matrine increased the level of cleaved caspase-3 relative to β-actin in the HCC rats. Quantitative analysis by densitometry scan showed that levels of cleaved caspase-3 relative to β-actin were increased in dose-dependent manner.

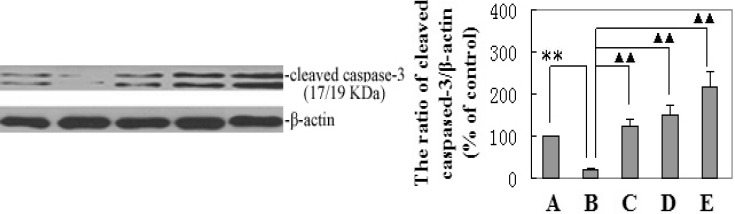
Semi-quantitative analysis of MLCK expression levels by Western blot
The ratio of MLCK to ß-actin in the rats of the normal group was set to 100 and used as the basis for comparison with the other groups. The ratio of MLCK to ß-actin in the model rats (242 ± 45) was significantly higher (P < 0.01) than that of the rats in the normal control group. The MLCK/ß-actin ratios of the rats in the matrine-treated groups (group-L: 205 ± 23, P < 0.05; group-M: 171 ± 16; group-H: 153 ± 18, P < 0.01)were significantly lower than that of the rats in the model group. This result suggests that matrine inhibits the MLCK overexpression caused by HCC (Figure 4).
The effect of matrine on MLCK immunochemistry in HCC tissues
Figure 5 shows the MLCK expression levels in the livers of the control, model, and matrine-treated rats. MLCK expression in the livers of the model rats was higher than the control group’s levels and expression in matrine-treated rats. Matrine-treated rats was lower than that of the model group. The MLCK integral absorbance in the livers of the model rats (285 ± 75) was significantly higher (P < 0.01) than that of the control group (81 ± 12). The MLCK integral absorbances of the treated rats (group-L: 243 ± 47, P < 0.05; group-M: 192 ± 38; group-H: 163 ± 29, P < 0.01) were all significantly lower than that of the model group. This result demonstrates the normalizing effect of matrine on MLCK overexpression in HCC tissues.
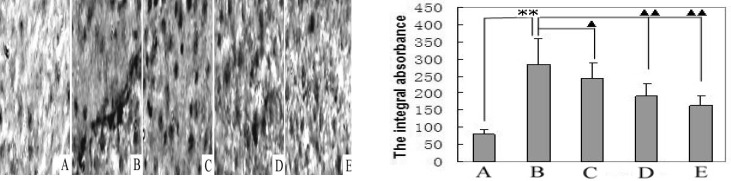
Discussion
Apoptosis plays an important role in the matrine-induced inhibition of tumor cell proliferation. In this study, we found that this apoptosis is mediated by the action of caspases, a group of cysteine proteases that can be activated through the p53 pathway. The results verified that p53 is a major player in the apoptosis process that is activated when HCC cells are exposed to matrine (31). Our results demonstrated the ability of matrine to significantly induce p53 expression, which is essential for the therapeutic effect of some clinical cancer drugs (32-34). The main function of the tumor suppressor p53 is to limit cellular proliferation by inducing apoptosis in response to matrine. Our results also demonstated that p53 mediates the apoptosis induced by matrine through a linear pathway involving Bax transactivation and the activation of caspase-3. MLCK hyperphosphorylated MLC II induces stress fiber formation and integrin clustering that link cell surface cytoskeletal proteins such as focal adhesion kinase (FAK) to actin (11, 12). Our immunohistochemistry results showed that MLCK expression increased dramatically at the tumor site in the rats of the model group, while MLCK expression in the matrine-treated rats was much lower. Western blot analysis further showed that in the rats of the model group, the MLCK expression level increased significantly. We confirmed that matrine could induce apoptosis in HCC cells and ultimately inhibit the proliferation of HCC cells by reversing MLCK overexpression. Matrine’s ability to inhibit the proliferation of HCC cells by regulating MLCK expression has not been reported previously, either in China or elsewhere. These results may indicate that matrine can be used as a component of tumor treatment and that MLCK can be a novel target for treating HCC. MLCK is closely associated with tumors and plays an important role in tumor cell proliferation, its specific inhibitor ML-7 has been reported to directly block tumor cell proliferation (9, 10). This study revealed that the inhibitory effect of matrine on HCC cell proliferation is associated with down-regulation of MLCK overexpression via the p53 apoptosis pathway. Matrine may thus be used as a potentially promising reagent to inhibit HCC cell proliferation and MLCK may be a novel target for the treatment of HCC.
Conclusion
In conclusion, we revealed that the MLCK expression was higher in the livers of HCC rats than in normal and matrine-treated rats. High level of MLCK expression was associated with inducing liver tumor cell proliferation and anti-apoptotic effects. Matrine inhibits HCC cell proliferation in rats through inducing apoptosis via p53, Bax-dependent caspase-3 activation pathway and down-regulating MLCK overexpression.
Acknowledgements
References
-
1.
Jemal A, Bray F, Center MM, Ferlay J, Ward E, Forman D. Global cancer statistics. Cancer J. Clin. 2011;61:69-90.
-
2.
Forner A, Llovet JM, Bruix J. Hepatocellular carcinoma. Lancet. 2012;379:1245-1255. [PubMed ID: 22353262].
-
3.
Klemke RL, Cai AL, Giannini PJ, Gallagher P, de Lanerolle, Cheresh DA. Regulation of cell motility by mitogen-activated protein kinase. J. Cell. Biol. 1997;137:481-492. [PubMed ID: 9128257].
-
4.
Frisch SM, Francis H. Disruption of epithelial cell-matrix interactions induces apoptosis. J. Cell. Biol. 1994;124:619-626. [PubMed ID: 8106557].
-
5.
Jacks T, Weinberg RA. Taking the study of cancer cell survival to a new dimension. Cell. 2002;111:923-925. [PubMed ID: 12507419].
-
6.
Wilson AK, Pollenz RS, Chisholm RL, de Lanerolle P. The role of myosin I and II in cell motility. Cancer Metastasis. Rev. 1992;11:79-91. [PubMed ID: 1511499].
-
7.
Elson EL. Cellular mechanics as an indicator of cytoskeletal structure and function. Annu. Rev. Biophys. Biophys. Chem. 1988;17:397-430. [PubMed ID: 3293593].
-
8.
Barkan D, Kleinman H, Simmons JL. Inhibition of metastatic outgrowth from single dormant tumor cells by targeting the cytoskeleton. Cancer Res. 2008;68:6241-6250. [PubMed ID: 18676848].
-
9.
Zhou X, Liu Y, You J. Myosin light-chain kinase contributes to the proliferation and migration of breast cancer cells through cross-talk with activated ERK1/2. CancerLett. 2008;270:312-327.
-
10.
Cui WJ, Liu Y, Zhou XL. Myosin light chain kinase is responsible for high proliferative ability of breast cancer cells via anti-apoptosis involving p38 pathway. Acta. Pharmacol. Sin. 2010;31:725-732. [PubMed ID: 20453870].
-
11.
Swant JD, Rendon BE, Symons M, Mitchell RA. Rho GTPase-dependent signaling is required for macrophage migration inhibitory factor-mediated expression of cyclin D1. J. Biol. Chem. 2005;280:23066-23072. [PubMed ID: 15840582].
-
12.
Sawhney RS, Cookson MM, Omar Y, Hauser J, Brattain MG. Integrin alpha-mediated ERK and calpain activation play a critical role in cell adhesion and motility via focal adhesion kinase signaling: identification of a novel signaling pathway. J. Biol. Chem. 2006;281:8497-8510. [PubMed ID: 16461767].
-
13.
Gilmore AP, Romer LH. Inhibition of focal adhesion kinase (FAK) signaling in focal adhesions decreases cell motility and proliferation. Mol. Biol. Cell. 1996;7:1209-1224. [PubMed ID: 8856665].
-
14.
Ilić D, Almeida EA, Schlaepfer DD, Dazin P, Aizawa S, Damsky CH. Extracellular matrix survival signals transduced by focal adhesion kinase suppress p53-mediated apoptosis. J. Cell. Biol. 1998;143:547-560. [PubMed ID: 9786962].
-
15.
Miller TM, Moulder KL, Knudson CM, Creedon DJ, Deshmukh M, Korsmeyer SJ, Johnson EM. Bax deletion further orders the cell death pathway in cerebellar granule cells and suggests a caspase independent pathway to cell death. J. Cell. Biol. 1997;139:205-217. [PubMed ID: 9314540].
-
16.
Martinou I, Missotten M, Fernandez PA, Sadoul R, Martinou JC. Bax and Bak proteins require caspase activity to trigger apoptosis in sympathetic neurons. Neuro. Rep. 1998;9:15-19.
-
17.
Li H, Tan G, Jiang X, Qiao H, Pan S, Jiang H, Kanwar JR, Sun X. Therapeutic effects of matrine on primary and metastatic breast cancer. Am. J. Chin. Med. 2010;38:115-1130.
-
18.
Tan C, Qian X, Jia R, Wu M, Liang Z. Matrine induction of reactive oxygen species activates p38 leading to caspase-dependent cell apoptosis in non-small cell lung cancer cells. Oncol. Rep. 2013;30:2529-2535. [PubMed ID: 24026034].
-
19.
Liu T, Song Y, Chen H, Pan S, Sun X. Matrine inhibits proliferation and induces apoptosis of pancreatic cancer cells in-vitro and in-vivo. Biol. Pharm. Bull. 2010;33:1740-1745. [PubMed ID: 20930385].
-
20.
Liu XY, Fang H, Yang ZG, Wang XY, Ruan LM, Fang DR, Ding YG, Wang YN, Zhang Y, Jiang XL, Chen HC. Matrine inhibits invasiveness and metastasis of human malignant melanoma cell line A375 in-vitro. Int. J. Dermatol. 2008;47:448-456. [PubMed ID: 18412860].
-
21.
Luo C, Zhong HJ, Zhu LM, Wu XG, Ying JE, Wang XH, Lü WX, Xu Q, Zhu YL, Huang J. Inhibition of matrine against gastric cancer cell line MNK45 growth and its anti-tumor mechanism. Mol. Biol. Rep. 2012;39:5459-5464. [PubMed ID: 22207169].
-
22.
Hui J, Yu S, Shuiying W, Xiaodong C. Matrine Activates PTEN to Induce Growth Inhibition and Apoptosis in V600E BRAF Harboring Melanoma Cells. Int.J. Mol. Sci. 2013;14:16040-16057. [PubMed ID: 23912239].
-
23.
Qin XG, Hua Z, Shuang W, Wang YH, Cui YD. Effects of matrine on HepG2 cell proliferation and expression of tumor relevant proteins in-vitro. Pharm. Biol. 2010;48:275-281. [PubMed ID: 20645813].
-
24.
Ma L, Wen S, Zhan Y, He Y, Liu X, Jiang J. Anticancer effects of the Chinese medicine matrine on murine hepatocellular carcinoma cells. Planta. Med. 2008;74:245-251. [PubMed ID: 18283616].
-
25.
Gao H, He S, Tang WX, Wang J. Inhibition of NF-kappa B activity enhanced apoptosis induced by matrine in hepatocellular carcinoma cells. Zhong. Hua. Gan. Zang. Bing. Za. Zhi. 2007;15:914-917.
-
26.
Zhang JQ, Li YM, Liu T, He WT, Chen YT, Chen XH, Li X, Zhou WC, Yi JF, Ren ZJ. Antitumor effect of matrine in human hepatoma G2 cells by inducing apoptosis and autophagy. World J. Gastroentero. 2010;14:4281-4290.
-
27.
Luo M, He P, Wu MC, Cui ZF, Li LF, Guo YJ. The Preventive Effect of Matrine and Oxymatrine on Rat Hapatoma Induced by Diethylnitrosamine. China. J. Cancer. Prev. Treat. 2000;7:561-563.
-
28.
Luo M, He P, Wu MC, Li LF, Guo YJ. Effects of matrine and oxymatrine on rat hepatoma induced by diethylnitrosamine. Chinese. Pharm. Bull. 2000;16:416-417.
-
29.
Ge SR, Xu HS, Shi WK, Li SK, Yao X. α-Fetoprotein in rat during diethylnitrosamine carcinogenesis. Acta Biologiac Experimentalis Sinica. 1978;11:105-108.
-
30.
Bishayee A, Dhir N. Resveratrol-Mediated chemoprevention of diethylnitrosamine- initiated hepatocarcinogenesis: Inhibition of cell proliferation and induction of apoptosis. Chem. Biol. Interact. 2009;179:131-144. [PubMed ID: 19073162].
-
31.
Horn HF, Vousden KH. Coping with stress: Multiple ways to activate p53. Oncogene. 2007;26:1306-1316. [PubMed ID: 17322916].
-
32.
Lin WC, Chuang YC, Chang YS, Lai MD, Teng YN, Su IJ, Wang CC, Lee KH, Hung JH. Endoplasmic reticulum stress stimulates p53 expression through NF-κB activation. PLoS. One. 2012;7:e39120. [PubMed ID: 22859938].
-
33.
Wu H, Lozano G. NF-kappa B activation of p53. A potential mechanism for suppressing cell growth in response to stress. J. Biol. Chem. 1994;269:20067-20074. [PubMed ID: 8051093].
-
34.
Ryan KM, Ernst MK, Rice NR, Vousden KH. Role of NF-κB in p53-mediated programmed cell death. Nature. 2000;404:892-897. [PubMed ID: 10786798].