Abstract
Keywords
Rosemary leaf hydro-alcoholic extract Cerebral ischemia reperfusion Infarct volume Brain edema Blood–brain barrier permeability Neuro-protective
Introduction
Acute ischemic stroke is a major cause of both death and disability (1). Nearly 85% of strokes are acute ischemic strokes which result from a sudden loss of blood supply to the cerebral regions (2). This, deprives the brain of oxygen and nutrients and initiates a dynamic sequence of patho-physiological events (3). Oxidative stress and inflammatory events play an integral role in the pathogenesis of cerebral ischemia (4).
Disruption of blood–brain barrier (BBB), subsequent edema is major contributors to the pathogenesis of ischemic stroke, the details for pathogenesis of ischemic stroke remains unelucidated (5). Edema formation after ischemia can cause an increase in brain mass (1), which in turn leads to cell death and deterioration of stroke (6). Thus, protection of BBB breakdown by natural products may be a promotional strategy for treatment of ischemic tolerance (5).
The current strategies of therapeutic management such as tPA have the potential to aggravate ischemic brain injuries by increasing infarct size, cerebral edema, and intracranial hemorrhage (7). Normally, the infarct core will grow and progressively replace the penumbra (8). Therefore, rescuing the ischemic penumbra from infarction by potentiating of ischemic tolerance in this tissue is the main goal of ischemic tolerance (9).
There is a significant increase in the research and development of herbal medicine based on scientific studies as a supplement (10). Rosemary (Rosmarinus officinalis L.) is an important medicinal herb from Lamiaceae family originated from Mediterranean region and has been cultivated for long time in Iran (11).
Phytochemical studies have shown that rosemary (Rosmarinus officinalis L.) extracts (REs) contain essential oils, terpenoids, flavonoids, and alkaloids (12), including carnosic acid (CA), rosmarinic acid, carnosol (CS), caffeic acid, and ursolic acid, which contribute to the biological activity of rosemary and could provide effective synergy (13).
It is well known that the aqueous and alcoholic extracts of the leaves of R. officinalis L. possess a variety of pharmacological properties, including anti-aging (14), hepatoprotective, anti-bacterial, anti-thrombotic, anti-ulceogenic, diuretic, anti-diabetic, antioxidant, anti-noceptive, anti-inflammatory, and anti-depressant activities (15). On the other hand, the bioactive components of REs exhibit potent antioxidant activities (16), reduce lipid peroxidation in heart and brain (cortex and hippocampus) (17), inhibit the production of reactive oxygen species, and suppress inflammatory response (18).
It has been reported that REs can be useful in the prevention of disorders due to angiogenesis (19) and atherosclerosis (20).
The effects of Rosmarinus officinalis leaf hydro-alcoholic extract (RHE) on ischemic–reperfusion injury of the rat brain still remain to be explored. We hypothesized whether RHE can induce ischemic tolerance for protecting the brain from focal ischemic stroke. Therefore, the aim of this study was to examine the effects of various doses of hydro-alcoholic extract from rosemary (Rosmarinus officinalis L.) leaf on BBB permeability, brain edema, brain injuries and neurological dysfunctions during the acute phase of injury following focal cerebral ischemia reperfusion in rats.
Materials and methods
Preparation of extract
The Arial parts of the cultivated Rosmarinus officinalis were collected in August 2011 from the Herb Farm of Shahid Beheshti University. Voucher specimen of the collected plants (voucher no; 1922 ) was confirmed at Medicinal Plant Herbarium (MPH), Department of Phytochemistry, Medicinal Plants and Drugs Research Institute, Shahid Beheshti University.
Dried and powdered rosemary leaves (500 g) were extracted using enough volume of ethanol/water (70:30) kept at dark room with temperature (23 °C) for 48 h. The resulting solution was filtered through filter paper. After filtration, the solvent was removed by rotary evaporator to produce an waxy semi- solid materials. The rosemary extract was dissolved in 0.2% Tween 80 and distilled water added as vehicle for oral (p.o.) grouping.
High performance liquid chromatography (HPLC)
A reverse phase HPLC analysis (RP-HPLC) was used to quantify the major active components of the extract. The HPLC system consisted of a K-1001 pump, a K-2800 photodiode array (PDA) detector Knauer (Berlin, Germany). The chromatographic separations were performed on Eurosphere C18 (5 µM, 250 mm × 4.6 mm), Knauer (Berlin, Germany). Data were acquired and processed using a Chromgate software. The mobile phase composed of 0.01% formic acid solution and methanol from 75:25 to 20:80 %v/v gradient over 30 min at a flow rate of 0.5 mL min−1 and column temperature of 25 °C. Detector response was set at 330 nm. RHE contains 1.3% caffeic acid (Sigma, London, UK), and 4% rosmarinic acid (Merck, Darmstadt, Germany).
Animals
All the experimental and animal handling procedures were approved by the Animal and Ethics Review Committee of the University of Kharazmi of Iran. Adult male Wistar rats (Razi Institute, Tehran, Iran) weighing 280-300 g were housed in standard cages in a temperature (22 ± 2 °C) with humidity (40 ± 5%), under a 12 h light/dark cycle (lights on at 07:00), and received standard diet and water freely.
Experimental
Animals were randomly divided into four groups (n = 18 in each group).The first group served as control and vehicle was given orally (p.o.); the other three groups were pretreated orally (p.o.) once daily (10-11.00 h), for 30 days with RHE (50, 75 and 100 mg/Kg body weight): doses identified using previously published data. 2 h after the last pretreatment, the rats subjected to 60 min of middle cerebral artery occlusion followed by 24 h of reperfusion. After 24 h reperfusion, neuro-behavioral studies, infarct volume (n = 6), water content (n = 6), and blood brain barrier permeability (n = 6) were measured. Additionally, 12 sham-operated animals underwent the same surgical procedures (without blocking the arteries).
Lipid profiles
The rats were anesthetized with chloral hydrate (400 mg/Kg i.p.) at 30 days after the initiation of pretreatment, just before surgery. Then, blood samples were taken by tail vein. The blood samples were centrifuged at 7000 × g for 10 min, room temperature to obtain plasma.
The serum samples were stored at -20 °C until biochemical analysis. Levels of HDL, triglyceride, and cholesterol content (Pars Azmun, Iran according to manufacturer’s instructions) were measured using an autoanalyzer (Liasys, Roma, Italy).
Middle cerebral artery occlusion
The right middle cerebral artery (MCA) occlusion (MCAO) was induced using an intraluminal filament model (21). The rats were anesthetized with chloral hydrate (Merck, Germany; 400 mg/kg i.p.). Briefly stating, neck vessels were exposed through a midline incision. Under an operating microscope, the right common carotid artery (CCA), the right external carotid artery (ECA), and the right internal carotid artery (ICA) were isolated. Then, a 3-0 silicone-coated nylon was carefully inserted from the external carotid artery into the internal carotid artery (ICA) until light resistance was felt. The filament was inserted approximately 20 mm from the carotid bifurcation to effectively block the middle cerebral artery (MCA). After 60 min of transient MCA occlusion (tMCAO), blood flow was restored by withdrawing the nylon filament and the ECA was permanently tied. During these procedures, body temperature was monitored with a rectal probe, and was maintained at 37±0.3 °C (Citizen-513w) using a heating pad. Animals were then recovered from anesthesia and were returned to their cages for 24 h. Sham animals were subjected to surgery with sutures of the same size but the suture was not advanced into the middle cerebral artery. The animals were kept at ambient temperature until sampling, with free access to water and food.
Neurological examination
Neurological function was evaluated using a 0-5 point scale neurological score (21) after 24 h of reperfusion: 0 = no neurological dysfunction; 1 = failure to extend opposite forepaw; 2 = circling to the contralateral side, when held by tail with feet on floor; 3 = falling to the left; 4 = unable to bear weight on affected side; 4 = no spontaneous walking and a depressed level of consciousness; 5 = death.
Measurement of infarct volume
The rats were decapitated under chloral hydrate anesthesia (800 mg/Kg), 24 h post-stroke and the brains were rapidly removed. The brains were cooled in ice-cold saline for 10 min and sectioned coronally into eight 2 mm-thick slices starting from the forebrain area (i.e. from the olfactory bulbs to the cortical-cerebellar junction),by using a Brain Matrix Slicer. Brain slices were incubated for 20 min in a 2% solution of 2, 3, 5-triphenyl tetrazolium chloride (Merk, Germany) and kept at 37 °C in a water bath for 15 min. The stained brains were then photographed using a digital camera connected to a computer (Cannon, Japan). Damaged regions were defined as areas that were completely white. The infarct area in each section was measured using image analysis software (UTHSCSA Image Tool). The infarct volume was calculated by taking the average of infarct area on both sides of the slice and multiplying it by section thickness. The total infarct volumes of each brain were calculated as the sum of the infarct volumes of each slice (22): corrected infarct volume = left hemisphere volume-(right hemisphere volume - infarct volume).
Water content of the brain
The rats were decapitated at 25 h after reperfusion and the brains were removed. Then, the pons and olfactory bulb were removed and the brain tissues were weighted immediately to obtain their wet weight (WW) (23). Subsequently, brains were dried at 110 °C for 24 h in an oven to determine their dry weight (DW). Brain water content (BWC), was calculated according to the formula: (WW−DW)/WW×100.
Evaluation of BBB permeability disruption
Measurement of Evans blue extravasations BBB permeability was assessed by measuring EB extravasations. Briefly stating, 4 mL/Kg of 2% Evans blue solution (EB, Sigma Chemicals, USA) in saline was injected into the tail vein, 30 min before reperfusion. Twenty-four h after, rats were deeply anesthetized, chest walls were opened, and trans-cardially perfused with 250 mL saline solution. After decapitation, the brain tissue was removed and hemispheres were separated and weighed. Each hemisphere was homogenized, in the 2.5 mL phosphate buffer saline, and then 2.5 mL of 60% trichloroacetic acid (Merck, Germany) was added to precipitate protein. The mixture was then vortexed for 3 min, cooled for 30 min and centrifuged for 30 min at 1000 ×g. The supernatants were measured at 610 nm for absorbance of EB using a spectrophotometer (UV-visible, USA). The results were expressed as microgram per gram brain tissue calculated according a standard curve (23).
Statistical analysis
All data are presented as the mean± S.E.M. Statistical analysis of neurological score was assessed using analysis of variance followed by a post hoc Fisher’s indicated least significant difference . For neurological score, we have applied Mann-Whitney U test. A p-value of less than 0.05 was considered to be statistically significant.
Results
Effects of RHE on body weight
The pretreatment with RHE at doses of 50, 75 mg/Kg/day for 30 days did not decrease body weight as compared with control and same pretreatment groups (Table 1.), but 100 mg/Kg/day of RHE significantly decreased body weight one day before the end of study (day 30).
Effects of the RHE pre-treatment on serum lipid levels
The total cholesterol (TC), triglyceride (TG), LDL-c levels were significantly reduced in the serum of rats supplemented with RHE at the doses of 50, 75, and 100 mg/Kg/day for 30 days as compared to the vehicle-treated rats. RHE pretreatment increased the level of serum HDL-c when compared with the vehicle-treated group. A significant reduction of the LDL/HDL-c and TG/HDL-c ratios was also seen in all consuming RHE groups as compared to control group at the end of thirtieth day (Figure 1.).
Effect of RHE on neurologic outcome
Median neurologic deficit scores (NDS) were reduced by RHE, being 1 (range: 0-2), 1 (range: 0-2), 1 (range: 0-1), and 2.5 (range: 0-4) in the doses of 50, 75 and 100 mg/Kg/day and control (0) for 30 days, respectively (Table 2.). The putative beneficial effects of RHE were confirmed by a reduction in infarct volume (Figure 2.).Therefore, the neurologic deficit scores (NDS) in the MCAO group pretreated with RHE at the doses of 50, 75, and 100 mg/Kg/day, for 30 days were reduced in comparison with the vehicle-pretreated MCAO group.
Dose-dependent effect of RHE on Infarct volume
In the control group, 1h-ischemia/24 h-reperfusion caused severe infarct in the cerebral cortex and subcortex areas. RHE pretreatment at doses of 50, 75 and 100 mg/Kg/day for 30 days reduced infarct volumes markedly with maximal protection at the doses of 75 and 100 mg/Kg/day as compared to the controls (Figure 2.). The neuro-protection, exerted by RHE was mainly seen in the cortex, while it was slightly observed in the infarct sub-cortex.
Effect of RHE on brain edema at 24 h post-tMCAO
Brain edema formation following induction of cerebral ischemia/reperfusion was determined by the measurement of the brain water content. Pretreatment of RHE at the doses of 50, 75, and 100 mg/Kg/day, reduced edema formation significantly as compared with control rats (the dose of 50 mg/Kg/day: left hemisphere = 78.95 ± 0.2%, right hemisphere = 79.92 ± 0.49%; the dose of 75 mg/Kg/day: left hemisphere = 78.81 ± 0.1%, right hemisphere = 79.45 ± 0.26%, dose of 100 mg/Kg/day: left hemisphere =78.68 ± 0.1%, right hemisphere = 79.3 ± 0.19% and in the control group: left hemisphere = 79.82±0.19%, right hemisphere = 82.34 ± 0.42%)(Figure 3.).
Effect of RHE on BBB dysfunction in stroke
The permeability of BBB followed by ischemia/reperfusion was evaluated by the amount of Evans Blue (EB) extravasations in the ischemic right hemisphere. In the MCAO-operated (control) group, EB content in the right cerebral hemisphere (ischemic) was 7.73 ± 0.4 and 4.25 ± 0.35 μg/g brain tissues in the left cerebral non-ischemic hemisphere. In contrast, RHE pretreatment at doses of 50, 75 and 100 mg/Kg/day for 30 days was significantly reduced extravasations of EB dye into the ischemic brain (The dose of 50 mg/Kg/day: non-ischemic hemisphere = 3.02 ± 0.25 μg/g tissue, ischemic hemisphere = 4.1 ± 0.23 μg/g tissue; the dose of 75 mg/Kg/day: non-ischemic hemisphere= 2.65 ± 0.18 μg/g tissue, ischemic hemisphere = 3.58 ± 0.3 μg/g tissue and dose of 100 mg/Kg/day: non-ischemic hemisphere = 2.63 ± 0.17 μg/g tissue, ischemic hemisphere= 3.38 ± 0.25 μg/g tissue)(Figure 4.).
Discussion
In the first part of our study, we evaluated for the first time that the effects of supplementation with RHE in the tMCAO model. The results of this evaluation revealed that pretreatment with RHE significantly reduced the BBB permeability disruption and cerebral edema, and more importantly, relieved brain infarction and neurological deficit scores after focal cerebral ischemia reperfusion.
Brain inflammation has recently emerged as a key player in the pathogenesis of I/R injury (1). It has been shown that RHEs is important for the prevention of phosphorylation of MAPKs, thereby blocking NF-kB activation, which in turn leads to decreased expression of iNOS and Cyclooxygenase-2 (COX-2) (2). Similarly, leukocytes activities, pro-inflammatory enzymes and mediators such as NO, IL-1β, and TNF-α were suppressed by REs during inflammation (3).
Oxidative stress is one of the most important mechanisms involved in pathogenesis of ischemic injuries and results in BBB disruption and neuronal death (4). It has also been reported that RHEs decrease lipid peroxidation and hydroxyl anion radical and hydrogen peroxide activities in rat serum, liver, kidney, heart, and brain tissues (5). Based on the mentioned evidences, it can be concluded that RHEs possesse strong antioxidant properties (6), even more than the phenol compounds (7).
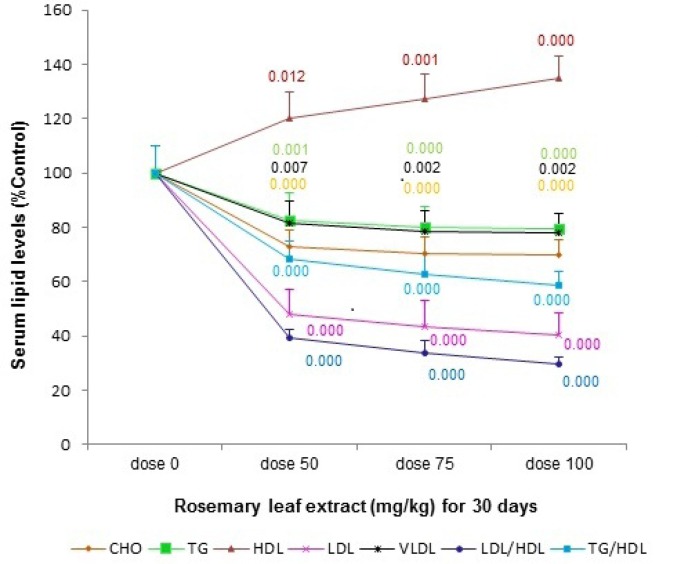
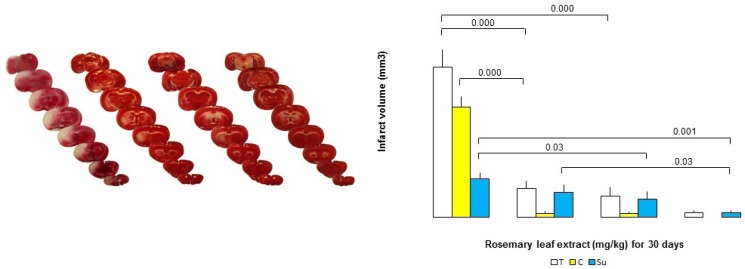
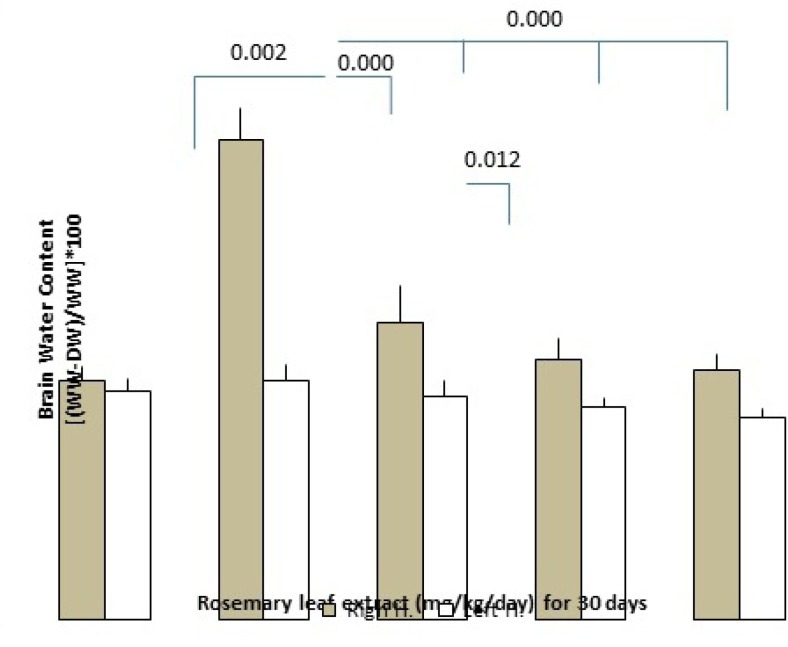
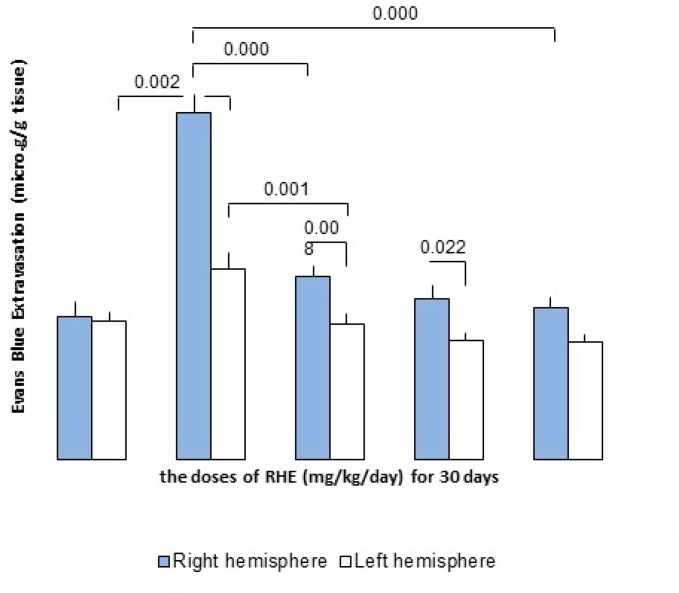
the body weight changes of animals on the 1st and 30thday 0, 50, 75, and 100 mg/Kg/day of RHE administration.
1th day of pretreatment | 30th pretreatment | |||||||
---|---|---|---|---|---|---|---|---|
0 | 50 | 75 | 100 | 0 | 50 | 75 | 100 | |
Body weigh | 296.6±2.4 | 289.3±3.4 | 297.6±4.0 | 295.9±2.5 | 331.6±3.1 | 323.3±3.6 | 327.5±3.3 | 315±2.8 |
Weight gain | 0 | 0 | 0 | 0 | 35±0.7 | 33.9±0.2 | 29.8±0.6 | 1 9 . 1 ± 0 . 3 (p=0.009) |
The distribution of neurologic deficit score in each group
No. | Experimental groups | NDS in each groups (N) | Total(N) | Median | Premature death | Statistical results | ||||||
---|---|---|---|---|---|---|---|---|---|---|---|---|
0 | 1 | 2 | 3 | 4 | 5 | |||||||
1 | Dose 0 | 1 | 2 | 6 | 8 | 1 | 0 | 18 | 2.5 | 2 | 1:2 sig. (0.000) | |
2 | Dose 50 | 7 | 8 | 3 | 0 | 0 | 0 | 18 | 1 | 0 | 1:3 sig. (0.000) | |
3 | Dose 75 | 7 | 9 | 2 | 0 | 0 | 0 | 18 | 1 | 0 | 1:4 sig. (0.000) | |
4 | Dose 100 | 8 | 10 | 0 | 0 | 0 | 0 | 18 | 1 | 1 | 3:4 nonsig. | |
- | Total (N) | 23 | 29 | 11 | 8 | 1 | 0 | 72 | - | 3 | 2:3, 2:4nonsig. |
The results showed that the administration of RHE resulted in an effective improvement of cerebral infarction, and neurological scores, also it is able to successfully attenuate BBB permeability which in turn lead to the reduction of brain edema. Thus, RHE protects against BBB disruption induced by acute ischemic injury through reducing brain edema, intracranial pressure, and restoring cerebral blood flow and energy.
Recent studies have reported that the phenolic compounds from Rosemary (Rosmarinus officinalis L.) not only attenuate oxidative stress (8,9) but also reduce cholesterol and triacyl-glycerols levels (10) in serum. It has been suggested that a biological active plasma level can be achieved by oral consumption of the concentration of 5% in the extract (11). Consistent with these studies, the second point of our study exhibited that the RHE supplementation significantly improved the TG/HDL-c and LDL/HDL-c ratios in a dose-dependent manner.
In conclusion, the present study demonstrated that cerebral ischemic tolerance induced by RHE pretreatment leads to a significant reduction of acute ischemic stroke lesion even in “ischemic penumbra” tissue that is extremely sensitive to hypoxic-ischemic damage. Therefore, RHE could markedly improve stroke outcome. Based on the mentioned evidences, although further works are required to clarify this issue, ischemic tolerance induced by RHE supplementation can be useful as a practical therapeutic in related diseases.
References
-
1.
Liu F, Akella P, Benashski SE, Xu Y, Mccullough LD. Expression of Na–K–Cl cotransporter and edema formation are age dependent after ischemic stroke. Exp. Neurol. 2010;224:356-61. [PubMed ID: 20406636].
-
2.
Liu ZH, Liu Q, Cai H, Xu CH, Liu G, Li ZH. Calcitonin gene-related peptide prevents blood–brain barrier injury and brain edema induced by focal cerebral ischemia reperfusion. Regul. Peptides. 2011;171:19-25.
-
3.
Rekik I, Allassonniere S, Carpenter TK, Wardlaw JM. Medical image analysis methods in MR/CT-imaged acute-subacute ischemic stroke lesion: Segmentation, prediction and insights into dynamic evolution simulation models. A critical appraisal. Neuroimage Clin. 2012;1:164-78. [PubMed ID: 24179749].
-
4.
Raza SS, Khan MM, Ahmad A, Ashafaq M, Islam F, Wagner AP, Safhi MM, Islam F. Neuroprotective effect of naringenin is mediated through suppression of NF-κB signaling pathway in experimental stroke. Neuroscience. 2013;230:157-71. [PubMed ID: 23103795].
-
5.
Huang P, Zhou CM, Hu Q, Liu YY, Hu BH, Chang X, Zhao XR, Xu XS, Li Q, Wei XH, Mao XW, Wang CS, Fun JY, Han JY. Cerebral care Granule attenuates blood–brain barrier disruption after middle cerebral artery occlusion in rats. Exp. Neurol. 2012;237:453-63. [PubMed ID: 22868201].
-
6.
Heo J, Han SW, Lee SK. Free radicals as triggers of brain edema formation after stroke. Free Radical Bio. Med. 2005;39:51-70. [PubMed ID: 15925278].
-
7.
Fanne RA, Nassar T, Yaraovoi S, Rayan A, Lamensdorf I, Karakoveski M, Vadim P, Jammal M, Cines DB, Higazi AR. Blood-brain barrier permeability and tPA–mediated neurotoxicity. Neuropharmacol. 2010;58:972-80.
-
8.
Kumar G, Goyal MK, Sahota PK, Jain R. Penumbra, the basis of neuroimaging in acute stroke treatment: Current evidence. J. Neurol. Sci. 2010;288:13-24. [PubMed ID: 19875134].
-
9.
Hughes JL, Beech JS, Jones PS, Wang D, Menon DK, Baron JC. Mapping selective neuronal loss and microglial activation in the salvaged neocortical penumbra in the rat. Neuroimage. 2010;49:19-31. [PubMed ID: 19716426].
-
10.
Sarris J, Panossian A, Schweitzer I, Stough C, Scholey A. Herbal medicine for depression, anxiety and insomnia: A review of psychopharmacology and clinical evidence. Eur. Neuropsychopharm. 2011;21:841-60.
-
11.
Minaiyan M, Ghannadi AR, Afsharipour M, Mahzouni P. Effects of extract and essential oil of Rosmarinus officinalis L on TNBS-induced colitis in rats. Res. Pharm. Sci. 2011;6:13-21. [PubMed ID: 22049274].
-
12.
Martinez AL, Gonzalez-Trujano ME, Chavez M, Pellicer F. Antinociceptive effectiveness of triterpenes from rosemary in visceral nociception. J. Ethnopharmacol. 2012;142:28-34. [PubMed ID: 22543174].
-
13.
Einbond LS, Wu HA, Kashiwazaki R, He K, Roller M, Su T, Wang X, Glodsberry S. Carnosic acid inhibits the growth of ER-negative human breast cancer cells and synergizes with curcumin. Fitoterapia. 2012;83:1160-8. [PubMed ID: 22828666].
-
14.
Abu-Al-Basal MA. Healing potential of Rosmarinus officinalis L on full-thickness excision cutaneous wounds in alloxan-induced-diabetic BALB/c mice. J. Ethnopharmacol. 2010;131:443-50. [PubMed ID: 20633625].
-
15.
Amaral GM, De-Carvalho NR, Barcelos RP, Dobrachinski F, De-Lima-Portella R, Da-Silva MH, Lugokenski TH, Mundstock-Dias GR, Da-Luz SC, Boligon AA, Athayde ML, Villetti MA, Soares FA, Fachinetto R. Protective action of ethanolic extract of Rosmarinus officinalis L in gastric ulcer prevention induced by ethanol in rats. Food Chem. Toxicol. 2013;55:48-55. [PubMed ID: 23279841].
-
16.
Cheng AC, Lee MF, Tsai ML, Lai CS, Lee JH, Ho CT, Pan MH. Rosmanol potently induces apoptosis through both the mitochondrial apoptotic pathway and death receptor pathway in human colon adenocarcinoma COLO 205 cells. Food Chem. Toxicol. 2011;49:485-93. [PubMed ID: 21112365].
-
17.
Posadas SJ, Cas V, Largo C, De-La-Candara B, Matallanas B, Reglero G, De Miguel E. Protective effect of supercritical fluid rosemary extract, Rosmarinus officinalis, on antioxidants of major organs of aged rats. Exp. Gerontol. 2009;44:383-9. [PubMed ID: 19289162].
-
18.
Lian KC, Chuang JJ, Hsieh CW, Wung BS, Huang GD, Jian TY, Sun YW. Dual Mechanisms of NF-κB inhibition in carnosol-treated endothelial cells. Toxicol. Appl. Pharm. 2010;245:21-35.
-
19.
Kayashima T, Matsubara K. Antiangiogenic effect of carnosic acid and carnosol, neuroprotective compounds in rosemary leaves. Biosci.Biotech. Bioch. 2012;76(1):115-9.
-
20.
Sinkovic A, Suran D, Lokar L, Fliser E, Skerget M, Novak Z, Knez Z. Rosemary extracts improve flow-mediated dilatation of the brachial artery and plasma pai-1 activity in healthy young volunteers. Phytother. Res. 2011;25:402-7. [PubMed ID: 20734322].
-
21.
Longa EZ, Weinstein PR, Carlson S, Cummins R. Reversible middle cere- bral artery occlusion without craniectomy in rats. Stroke. 1989;20:84-91. [PubMed ID: 2643202].
-
22.
Swanson RA, Morton MT, Tsao-Wu G, Savalos RA, Davidson C, Sharp FR. A semiautomated method for measuring brain infarct volume. J. Cereber. Blood F. Met. 1990;10(2):290-3.
-
23.
Bigdeli MR, Hajizadeh S, Froozandeh M, Rasulian B, Heidarianpour A, Khoshbaten A. Prolonged and intermittent normobaric hyperoxia induce different degrees of ischemic tolerance in rat brain tissue. Brain Res. 2007;1152:228-33. [PubMed ID: 17475225].
-
24.
Wei Z, Chigurupati S, Arumugam TV, Jo DG, Li H, Chan SL. Notch activation enhances the microglia-mediated inflammatory response associated with focal cerebral ischemia. Stroke. 2011;42:2589-94. [PubMed ID: 21737799].
-
25.
Yu MH, Choi JH, Chae IG, Im HG, Yang SA, More K, Lee IS, Lee J. Suppression of LPS-induced inflammatory activities by Rosmarinus officinalis L. Food Chem. 2013;136:1047-54. [PubMed ID: 23122161].
-
26.
Beninca JP, Dalmarco JB, Pizzolatti MG, Frode TS. Analysis of the anti-inflammatory properties of Rosmarinus officinalis L in mice. Food Chem. 2011;124:468-75.
-
27.
Sugawara T, Chan PH. Reactive oxygen radicals and pathogenesis of neuronal death after cerebral ischemia. Antioxid. Redox Sign. 2003;5:597-607.
-
28.
Botsoglou N, Taitzoglou I, Zervos I, Botsoglou E, Tsantarliotou M, Chatzopoulou PS. Potential of long-term dietary administration of rosemary in improving the antioxidant status of rat tissues following carbon tetrachloride intoxication. Food Chem. Toxicol. 2010;48:944-50. [PubMed ID: 20060873].
-
29.
Shiga A, Saito Y, Furuhashi T, Hoshizaki JI, Yoshida Y, Niki E. Antioxidative effects of volatile constituents of a rosemary extract on the lipid oxidation and cell damage. Antioxidants, Nutrition & Health. 2004:51-103.
-
30.
Hernandez-Hernandez E, Ponce-Alquicira E, Jaramillo-Flores ME, Legarreta G. Antioxidant effect rosemary (Rosmarinus officinalis L) and oregano (Origanum vulgare L) extracts on TBARS and colour of model raw pork batters. Meat Sci. 2009;81:410-7. [PubMed ID: 22064182].
-
31.
Park S, Kim S, Sapkota K, Kim S. Neuroprotective effect of Rosmarinus officinalis extract on human dopaminergic cell line, SH-SY5Y. Cell. Mol. Neurobiol. 2010;30:759-67. [PubMed ID: 20563702].
-
32.
Zegura B, Dobnik D, Niderl MH, Filipi M. Antioxidant and antigenotoxic effects of rosemary (Rosmarinus officinalisL) extracts in Salmonella typhimurium TA98 and HepG2 cells. Environ. Toxicol. phar. 2011;32:296-305.
-
33.
Ibarra A, Cases J, Roller M, Chiralt-Boix A, Coussaert A, Ripoll Ch. Carnosic acid-rich rosemary (Rosmarinus officinalis L) leaf extract weight gain and improves cholesterol levels and glycaemia in mice a high-fat diet. Brit. J. Nutr. 2011;106:1182-9. [PubMed ID: 21676274].
-
34.
Tai J, Cheung S, Wu M, Hasman D. Antiproliferation effect of Rosemary (Rosmarinus officinalis) on ovarian cancer cells in vitro human. Phytomedicine. 2012;19:436-43. [PubMed ID: 22325591].