Abstract
Keywords
1 3 4-Oxadiazole-2-thione β-Pyranosyl Thioglycoside Nucleoside in-vitro Acinetobacter calcoaceticus Antiproliferative activity
Introduction
The resistance of infective bacteria to present antibiotics demands research assigned to the discovery of new drugsin the antibacterial drug field. The majority of carbohydrates found in nature or biological systems exist as glycoconjugates in which the monosaccharide units are joined via O-, N-, or S-glycosidic bonds. Thioglycosides have received considerable attention, because they are widely employed as biological inhibitors, inducers and ligandsfor affinity chromatography of carbohydrate-processing enzymes and proteins (1-8). They have excellent chemoselectivity in glycosylation processes as both donors and acceptorsparticularly via reaction processesthat involve active and latent glycosylation protocols (9). The thioglycosyl heterocycles are sufficiently stable under a variety of reaction conditions and have the ability to be readilyconverted into a variety of other functionalities (10, 11). Multivalent display of carbohydrates is frequently used as a method to increase affinities in various contexts such as the binding of bacteria, bacterial toxins, galectins and other lectins (12-24). These properties may affect medicinal effect of antibiotic agents.
On the other hand, oxadiazole derivatives, which belong to an important group of heterocyclic compounds, have been thesubject of extensive study in the recent past. Numerous reports have highlighted their chemistry anduse. Diverse biological activities, such as antiinflamatory, antitumor, antimicrobial and anticonvulsant, have been found to be associated with oxadiazole derivatives (25-28). Moreover, sulfur-containing heterocycles represent an important group of sulfur compounds that are promising for use in practical applications.
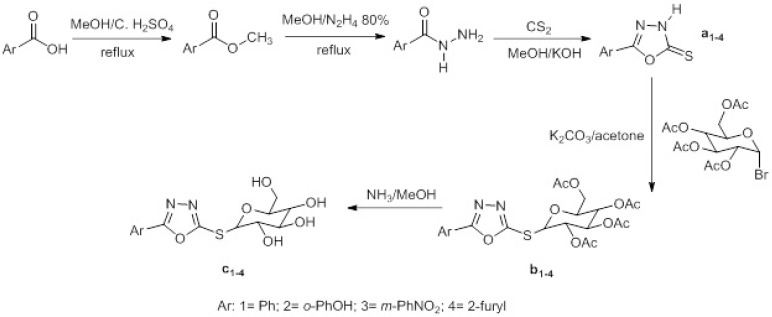
In-vitro antibacterial activity of synthetic compounds against Acinetobacter Calcoaceticus ATCC 23055 (concentration = 10 µg/µL
Compound | Diameter of inhib. zone (mm) | Compound | Diameter of inhib. zone (mm) |
---|---|---|---|
a4 | 22 | c4 | 31 |
a1 | 19 | c1 | 28 |
a2 | 18 | c2 | 29 |
a3 | 16 | c3 | 25 |
Therefore, it is interesting to report the synthesis of a new series of compounds in which the glycosyl moieties have been used as carriers for the heterocycles having the oxadiazole ring.
In our previous work (29), we reported the synthesis antibacterial properties of new series of thioglycoside derivatives of 1,2,4-triazole-5-thiones, whereas in the present work, we report the synthesisof new groups of anti-Acinetobacter calcoaceticus agents in which 1,3,4-oxadiazole-2-thiones moiety is coupled to monosaccharide unit.
Experimental
1H and 13C NMR spectra were recorded on a Bruker AVANCE-300 spectrometer at 300 and 75 MHz, respectively in CDCl3 using TMS as the internal standard. High-resolutionmass spectra were obtained with a HPLC-Q-TOF system equipped with Q-TOF micromass spectrometer (dual ESI). Melting points were measured on a Philip Harris C4954718apparatus without calibration. Optically active samples were analyzed by EHARTNACKapparatus (Paris, France) at 20 °C in dichloromethane. Thin layer chromatography (TLC) analyses were carried out on silica gel plates. All chemicals were purchased from Merckand used as received.
5-phenyl-1,3,4-oxadiazole-2(3H)-thione (a1)
Yield: 74%; mp 158-160 °C; 1H NMR spectrum, (300 MHz, CDCl3), δ, ppm, (J, Hz): ): 7.52 (m, 3H, ArH), 7.96 (d, J = 6.9, 2H, ArH) 10.75 (bs, 1H, N-H); 13C NMR spectrum, (75 MHz, CDCl3), δ, ppm: 122.90, 126.47, 129.85, 132.67, 160.90 (Ar), 177.88 (C=S).
5-(2-hydroxyphenyl)-1,3,4-oxadiazole-2(3H)-thione (a2)
Yield: 84%; mp 162-164 °C; 1H NMR spectrum, (300 MHz, CDCl3), δ, ppm, (J, Hz): ): 7.10 (m, 2H, ArH), 7.49 (t, 1H, J = 7.1, ArH), 7.72 (2, 1H, J = 8.1, ArH), 8.39 (bs, 1H, OH), 11.34 (bs, 1H, N-H); 13C NMR spectrum, (75 MHz, CDCl3), δ, ppm: 109.79, 111.17, 119.84, 129.50, 133.87, 156.78, 160.27 (Ar), 177.47 (C=S).
5-(3-nitrophenyl)-1,3,4-oxadiazole-2(3H)-thione (a3)
Yield: 78%; mp 167-168 °C; 1H NMR spectrum, (300 MHz, CDCl3), δ, ppm, (J, Hz): ): 7.40-7.60 (m, 3H, ArH), 7.94 (d, J = 7.8, 1H, ArH), 11.10 (bs, 1H, OH); 13C NMR spectrum, (75 MHz, CDCl3), δ, ppm: 120.93, 124.43, 126.85, 131.76, 132.44, 148.53, 159.15 (Ar), 178.04 (C=S)
5-(2-furyl)-1,3,4-oxadiazole-2(3H)-thione (a4)
Yield: 69%; mp 152-155 °C; 1H NMR spectrum, (300 MHz, CDCl3), δ, ppm, (J, Hz): ): 6.62 (bs, 1H, Furyl), 7.18 (bs, 1H, Furyl), 7.66 (bs, 1H, Furyl), 11.38 (bs, 1H, N-H); 13C NMR spectrum, (75 MHz, CDCl3), δ, ppm: 113.05, 115.37, 137.99, 147.61, 153.90 (Ar), 177.10 (C=S).
2-phenyl-5-(2,3,4,6-tetra-O-acetyl-β-D-1-thio-glucopyranose)-1,3,4-oxadiazole (b1)
Yield: 64%; mp 102-105 °C; 1H NMR spectrum, (300 MHz, CDCl3), δ, ppm, (J, Hz): ): 1.95 (s, 3H, OAc), 2.04 (s, 3H, OAc), 2.09 (s, 3H, OAc), 2.18 (s, 3H, OAc), 3.88-3.99 (m, 1H, H-6a), 4.12-4.20 (m, 1H, H-6b), 4.27-4.30 (m, 1H, H-5), 5.14-5.71 (m, 3H, H-2, -3, -4), 5.96 (d, 1H, J1,2 = 9.3, H-1), 7.52 (m, 3H, ArH), 7.94-8.04 (m, 2H, ArH); 13C NMR spectrum, (75 MHz, CDCl3), δ, ppm: 20.56 (2C), 20.72 (2C) (4 × OCOCH3), 61.55 (C-6), 67.56 (C-4), 69.76 (C-2), 73.52 (C-3), 74.71 (C-5), 83.42 (C-1), 121.93, 126.80, 129.18, 132.03, 132.79 (Ar), 166.52 (C−S), 168.93, 168.37, 170.06, 170.58 (4 × OCOCH3).
2-(2-hydroxyphenyl)-5-(2,3,4,6-tetra-O-acetyl-β-D-1-thio-glucopyranose)-1,3,4-oxadiazole (b2)
Yield: 66%; mp 99-101 °C; 1H NMR spectrum, (300 MHz, CDCl3), δ, ppm, (J, Hz): ): 2.03 (s, 3H, OAc), 2.04 (s, 3H, OAc), 2.06 (s, 3H, OAc), 2.10 (s, 3H, OAc), 3.88-3.92 (m, 1H, H-6a), 4.13-4.17 (m, 1H, H-6b), 4.27-4.32 (m, 1H, H-5), 5.14-5.37 (m, 3H, H-2, -3, -4), 5.50 (d, 1H, J1,2 = 9.6, H-1), 7.02 (t, 1H, J = 7.8, ArH), 7.13 (d, 1H, J = 8.4, ArH), 7.47 (t, 1H, J = 6.9, ArH), 7.72 (d, 1H, J = 7.8, ArH), 9.87 (bs, 1H, OH); 13C NMR spectrum, (75 MHz, CDCl3), δ, ppm: 20.54 (2C), 20.60 (2C) (4 × OCOCH3), 61.56 (C-6), 67.72 (C-4), 69.74 (C-2), 73.46 (C-3), 76.62 (C-5), 83.33 (C-1), 107.58, 117.66, 120.11, 126.54, 134.04, 157.34, 160.02 (Ar), 165.92 (C−S), 169.34, 169.43, 169.966, 170.54 (4 × OCOCH3).
2-(3-nitrophenyl)-5-(2,3,4,6-tetra-O-acetyl-β-D-1-thio-glucopyranose)-1,3,4-oxadiazole (b3)
Yield: 58%; mp 112-113 °C; 1H NMR spectrum, (300 MHz, CDCl3), δ, ppm, (J, Hz): ): 2.04 (s, 3H, OAc), 2.06 (s, 3H, OAc), 2.09 (s, 3H, OAc), 2.10 (s, 3H, OAc), 3.88-4.05 (m, 1H, H-6a), 4.14-4.21 (m, 1H, H-6b), 4.28-4.33 (m, 1H, H-5), 5.16-5.67 (m, 3H, H-2, -3, -4), 5.97 (d, 1H, J1,2 = 9.3, H-1), 7.75 (t, J = 7.8, 1H, ArH), 8.27-8.43 (m, 2H, ArH), 8.84 (d, J = 8.1, 1H, ArH); 13C NMR spectrum, (75 MHz, CDCl3), δ, ppm: 20.53 (2C), 20.65 (2C) (4 × OCOCH3), 61.50 (C-6), 67.70 (C-4), 69.71 (C-2), 73.49 (C-3), 74.83 (C-5), 83.32 (C-1), 121.80, 124.93, 126.36, 127.03, 130.52, 132.21, 148.67 (Ar), 164.50 (C-S), 169.33, 169.44, 169.97, 170.53 (4 × OCOCH3).
2-(2-furyl)-5-(2,3,4,6-tetra-O-acetyl-β-D-1-thio-glucopyranose)-1,3,4-oxadiazole (b4)
Yield: 72%; mp 107-109 °C; 1H NMR spectrum, (300 MHz, CDCl3), δ, ppm, (J, Hz): ): 1.97 (s, 3H, OAc), 2.04 (s, 3H, OAc), 2.07 (s, 3H, OAc), 2.10 (s, 3H, OAc), 3.85-4.00 (m, 1H, H-6a), 4.13-4.21 (m, 1H, H-6b), 4.27-4.32 (m, 1H, H-5), 5.24 (t, 1H, J1,2=J2,3 = 9.3, H-2), 5.42 (t, 1H, J2,3=J3,4 = 9.3, H-4), 5.61 (t, 1H, J2,3=J3,4 = 9.3, H-3), 6.34 (d, 1H, J1,2 = 9.3, H-1), 6.61 (bs, 1H, Furyl), 7.17 (bs, 1H, Furyl), 7.66 (bs, 1H, Furyl); 13C NMR spectrum, (75 MHz, CDCl3), δ, ppm: 20.49 (2C), 20.70 (2C) (4 × OCOCH3), 61.54 (C-6), 67.51 (C-4), 69.40 (C-2), 73.04 (C-3), 74.75 (C-5), 83.17 (C-1), 112.36, 116.17, 137.41, 146.80, 152.37 (Ar), 159.98 (C-S), 168.97, 169.28, 170.04, 170.56 (4 × OCOCH3).
2-phenyl-5-(β-D-1-thio-glucopyranose)-1,3,4-oxadiazole (c1)
Yield: 33%; mp 108-110 °C; [α]D20= 3° (c = 1.0, CH2Cl2). 1H NMR spectrum, (300 MHz, CDCl3), δ, ppm, (J, Hz): ): 3.92-4.03 (m, 1H, H-6a), 4.12-4.22 (m, 1H, H-6b), 4.27-4.30 (m, 1H, H-5), 4.35-4.85 (m, 4H, OH), 5.12-5.70 (m, 3H, H-2, -3, -4), 5.99 (d, 1H, J1,2 = 9.3, H-1), 7.50 (m, 3H, ArH), 7.94-8.02 (m, 2H, ArH); 13C NMR spectrum, (75 MHz, CDCl3), δ, ppm: 61.76 (C-6), 67.64 (C-4), 69.26 (C-2), 73.59 (C-3), 74.78 (C-5), 83.37 (C-1), 122.23, 126.88, 129.40, 132.09, 132.99 (Ar), 166.45 (C−S); HRMS spectrum (ESI), m/z: Calculated, 340.0729. C14H16N2O6S [M+H]+. Found, 341.0817.
2-(2-hydroxyphenyl)-5-(β-D-1-thio-glucopyranose)-1,3,4-oxadiazole (c2)
Yield: 46%; mp 144-145 °C; [α]D20= 4° (c = 1.0, CH2Cl2). 1H NMR spectrum, (300 MHz, CDCl3), δ, ppm, (J, Hz): ): 3.86-3.97 (m, 1H, H-6a), 4.11-4.16 (m, 1H, H-6b), 4.26-4.32 (m, 1H, H-5), 4.37-4.83 (m, 4H, OH), 5.13-5.37 (m, 3H, H-2, -3, -4), 5.57 (d, 1H, J1,2 = 9.6, H-1), 7.03 (t, 1H, J = 7.8, ArH), 7.12 (d, 1H, J = 8.4, ArH), 7.49 (t, 1H, J = 6.9, ArH), 7.74 (d, 1H, J = 7.8, ArH), 9.97 (bs, 1H, OH); 13C NMR spectrum, (75 MHz, CDCl3), δ, ppm: 62.46 (C-6), 68.02 (C-4), 69.86 (C-2), 73.65 (C-3), 76.69 (C-5), 83.04 (C-1), 109.18, 117.36, 121.01, 126.94, 133.84, 157.86, 160.23 (Ar), 165.57 (C−S); HRMS spectrum (ESI), m/z: Calculated, 356.0678. C14H16N2O7S [M+H]+. Found, 357.0762.
2-(3-nitrophenyl)-5-(β-D-1-thio-glucopyranose)-1,3,4-oxadiazole (c3)
Yield: 39%; mp 122-124 °C; [α]D20= -2° (c = 1.0, CH2Cl2). 1H NMR spectrum, (300 MHz, CDCl3), δ, ppm, (J, Hz): ): 3.84-4.01 (m, 1H, H-6a), 4.17-4.25 (m, 1H, H-6b), 4.24-4.33 (m, 1H, H-5), 4.36-4.85 (m, 4H, OH), 5.16-5.68 (m, 3H, H-2, -3, -4), 6.07 (d, 1H, J1,2 = 9.3, H-1), 7.78 (t, J = 7.8, 1H, ArH), 8.27-8.46 (m, 2H, ArH), 8.81 (d, J = 8.4, 1H, ArH); 13C NMR spectrum, (75 MHz, CDCl3), δ, ppm: 62.20 (C-6), 67.74 (C-4), 69.79 (C-2), 73.67 (C-3), 74.35 (C-5), 83.44 (C-1), 121.89, 123.90, 126.86, 127.43, 131.82, 132.28, 149.63 (Ar), 165.22 (C-S); HRMS spectrum (ESI), m/z: Calculated, 385.0580. C14H15N3O8S [M+H]+. Found, 386.0665.
2-(2-furyl)-5-(β-D-1-thio-glucopyranose)-1,3,4-oxadiazole (c4)
Yield: 52%; mp 111-113 °C; [α]D20= -4° (c = 1.0, CH2Cl2). 1H NMR spectrum, (300 MHz, CDCl3), δ, ppm, (J, Hz): ): 3.82-4.08 (m, 1H, H-6a), 4.17-4.23 (m, 1H, H-6b), 4.24-4.39 (m, 1H, H-5), 4.35-4.89 (m, 4H, OH), 5.27 (t, 1H, J1,2=J2,3 = 9.3, H-2), 5.45 (t, 1H, J2,3=J3,4 = 9.3, H-4), 5.69 (t, 1H, J2,3=J3,4 = 9.3, H-3), 6.31 (d, 1H, J1,2 = 9.3, H-1), 6.66 (bs, 1H, Furyl), 7.17 (bs, 1H, Furyl), 7.58 (bs, 1H, Furyl); 13C NMR spectrum, (75 MHz, CDCl3), δ, ppm: 60.94 (C-6), 66.98 (C-4), 69.87 (C-2), 73.54 (C-3), 74.85 (C-5), 83.63 (C-1), 112.30, 117.20, 137.83, 146.88, 152.52 (Ar), 162.07 (C-S); HRMS spectrum (ESI), m/z: Calculated, 330.0522. C12H14N2O7S [M+H]+. Found, 331.0609.
Bacterial Strain
The antibacterial activity of compounds was assayed with our previous published
method (30). The antibacterial activity of the compounds was tested against Gram-negative strain of Acinetobacter calcoaceticus ATCC 23055.
Resultsand Discussion
Thioglycosilation was performed according our previous published method (29). 1-bromide sugar and 1,3,4-oxadiazole-2-thione nuclei a1-4 were synthesized to the literature procedures (29,31). Deprotection of acetylated nucleosides was performed to the literature procedure (32). The synthesis of the final nucleosides is depicted in Figure 1.
The structure of thioglycosides was confirmed by appropriate spectroscopic methods such as1HNMR,13C NMR, and high resolution mass spectroscopy (HRMS). The anomeric protons of nucleosides c1–4 were assigned to the doublet at 5.57–6.31 ppm with J1,2=9.3–9.6 Hz, confirming theβ-configuration.
In our recent published research (29), there was not any significant change in the antibacterial effect of the acetylated final thioglycosides in comparison with the parent heterocyclic nuclei. Therefore, in the present work, the deacetylated nucleosides c1-4 have been compared to the parent heterocyclic nuclei a1-4 against Acinetobacter calcoaceticus.
The in vitro antibacterial activity of the synthesized compounds in DMSO against Acinetobacter calcoaceticus is shown in Tables 1. Diameter of inhibition zone for ampicillin as reference drug is 18 mm for 10 µg/µL concentration of drug in the same test conditions.
As shown in the Table 1. these compounds showed higher antibacterial effects in comparison with Ampicillin (18 mm) which is normally used for treating such infections.
In general, compounds from c set (nucleosides) showed more antimicrobial activity than the other set. Thioglycoside derivatives of 1,3,4-oxadiazole-2-thiones (c set) were more active against Acinetobacter calcoaceticus ATCC 23055 than “parent” 1,3,4-oxadiazole-2-thiones (a set), confirming the relation between glyco-conjugation and increasing of antiproliferative activity of antibiotic agents. The best results in the tables belonged to c4that showing high activity against A. calcoaceticus (31 mm).
Going over the structure of these synthetic compounds confirmed that the existence of 2-furyl instead of m-PhNO2 group as Ar increased their antibacterial activity against A. calcoaceticus. The existence of m-PhNO2 group as Ar in structures of a set and their corresponding sugar derivatives decreased the antibacterial activity of them in comparison with the rest of synthetic compounds.
References
-
1.
Marino C, Marino K, Miletti L, Manso Alves MJ, Colli W, de Lederkremer RM. 1-Thio-β-D-galactofuranosides: synthesis and evaluation as β-D-galactofuranosidase inhibitors. Glycobiology. 1998;8:901-4. [PubMed ID: 9675223].
-
2.
Awad OME, Attia WE, El Ashry ESH. Comparative evaluation of D-glucosylthiouronium, glucosylthioheterocycles, Daonil and insulin as inhibitors for hepatic glycosidases. Carbohydr. Res. 2004;339:469-76. [PubMed ID: 15013383].
-
3.
El Ashry ESH, Awad LF, Atta IA. Synthesis and role of glycosylthioheterocycles in carbohydrate chemistry. Tetrahedron. 2006;62:2943-98.
-
4.
Apparu C, Driguez H, Williamson G, Svensson B. Chemoenzymatic synthesis of 6ω-S-α-d-glucopyranosyl-6ω-thiomaltooligosaccharides: their binding to Aspergillus nigerglucoamylase G1 and its starch-binding domain. Carbohydr. Res. 1995;277:313-20. [PubMed ID: 8556738].
-
5.
Birk R, Ikan A, Bravdo B, Braun S, Shoseyov O. Synthesis of lsopropyl-1-thio-Β-D-glucopyranoside (IPTGlc), an inducer of aspergillus niger b1 Β-glucosidase production. Appl. Biochem. Biotechnol. 1997;66:25-30. [PubMed ID: 9204516].
-
6.
Defaye J, Guillot JM, Biely P, Vrsanska M. Positional isomers of thioxylobiose, their synthesis and inducing ability for D-xylan-degrading enzymes in the yeast Cryptococcus albidus. Carbohydr. Res. 1992;228:47-64. [PubMed ID: 1516094].
-
7.
Schnaar RL, Lee YC. Polyacrylamide gels copolymerized with active esters New medium for affinity systems. Biochemistry. 1975;14:1535-41. [PubMed ID: 235956].
-
8.
Orgeret C, Scillier E, Gautier C, Defaye J, Driguez H. 4-Thiocellooligosaccharides Their synthesis and use as ligands for the separation of cellobiohydrolases of Trichodermareesei by affinity chromatography. Carbohydr. Res. 1992;224:29-40. [PubMed ID: 1591767].
-
9.
Mereyala HB, Gurijala VR. Use of 2-pyridyl 2-acetamido-3,4,6-tri-O-acetyl-2-deoxy-1-thio-beta-D-glucopyranoside as a glycosyl donor and methyl iodide as an activator for the synthesis of 1,2-trans-linked saccharides. Carbohydr. Res. 1993;242:277-80. [PubMed ID: 8495443].
-
10.
Chen Q, Kong F. Stereoselective glycosylation using fully benzylated pyrimidin-2-yl 1-thio-β-d-glycopyranosides. Carbohydr. Res. 1995;272:149-57.
-
11.
Ding X, Yang G, Kong F. Synthesis and glycosylation of pyrimidin-2-yl 1-thio-α-d-manno- and -α-l-rhamnopyranoside. Carbohydr. Res. 1998;310:135-9.
-
12.
Mammen M, Choi SK, Whitesides GM. Polyvalent interactions in biological systems: implications for design and use of multivalent ligands and inhibitors. Angew. Chem. Int. Ed. 1998;37:2754-94.
-
13.
Lee RT, Lee YC. Affinity enhancement by multivalent lectin-carbohydrate interaction. Glycoconjugate J. 2000;17:543-51.
-
14.
Lindhorst TK, Dubber M, Krallmann-Wenzel U, Ehlers S. Cluster mannosides as inhibitors of type 1 fimbriae-mediated adhesion of Escherichia coli: pentaerythritol derivatives as scaffolds. Eur. J. Org. Chem. 2000;11:2027-34.
-
15.
Nagahori N, Lee RT, Nishimura SI, Pag´ e D, Roy R, Lee YC. Inhibition of adhesion of type 1 fimbriated Escherichia coli to highly mannosylated ligands. Chem. Bio. Chem. 2002;3:836-44.
-
16.
Autar R, Khan AS, Schad M, Hacker J, Liskamp RMJ, Pieters RJ. Adhesion inhibition of F1C-fimbriated Escherichia coli and Pseudomonas aeruginosa PAK and PAO by multivalent carbohydrate ligands. Chem. Bio. Chem. 2003;4:1317-25.
-
17.
Joosten JAF, Loimaranta V, Appeldoorn CCM, Haataja S, El Maate FA, Liskamp RMJ, Finne J, Pieters RJ. Inhibition of Streptococcus suis adhesion by dendritic galabiose compounds at low nanomolar concentration. J. Med. Chem. 2004;47:6499-508. [PubMed ID: 15588085].
-
18.
Fan E, Zhang Z, Minke WE, Hou Z, Verlinde CLMJ, Hol WGJ. High-affinity pentavalent ligands of Escherichia coli heat-labile enterotoxin by modular structure-based design. J. Am. Chem. Soc. 2000;122:2663-4.
-
19.
Kitov PI, Sadowska JM, Mulvey G, Armstrong GD, Ling H, Pannu NS, Read RJ, Bundle DR. Shiga-like toxins are neutralized by tailored multivalent carbohydrate ligands. Nature. 2000;403:669-72. [PubMed ID: 10688205].
-
20.
Arosio D, Vrasidas I, Valentini P, Liskamp RMJ, Pieters RJ, Bernardi A. Synthesis and cholera toxin binding properties of multivalent GM1 mimics. Org. Biomol. Chem. 2004;2:2113-24. [PubMed ID: 15254640].
-
21.
Andr´e S, Pieters RJ, Vrasidas I, Kaltner H, Kuwabara I, Liu FT, Liskamp RMJ, Gabius HJ. Wedge like Glycodendrimers as inhibitors of binding of mammalian galectins to glycoproteins, lactose maxiclusters, and cell surface glycol conjugates. Chem. Bio. Chem. 2001;2:822-30.
-
22.
Vrasidas I, Andr´e S, Valentini P, B¨ock C, Lensch M, Kaltner H, Liskamp RMJ, Gabius HJ, Pieters RJ. Rigidified multivalent lactose molecules and their interactions with mammalian galectins: a route to selective inhibitors. Org. Biomol. Chem. 2003;1:803-10. [PubMed ID: 12929363].
-
23.
Wittmann V, Seeberger S. Spatial screening of cyclic neoglycopeptides: identification of polyvalent wheat-germ agglutinin ligands. Angew. Chem. Int. Ed. 2004;43:900-3.
-
24.
Woller EK, Walter ED, Morgan JR, Singel DJ, Cloninger MJ. Altering the strength of lectin binding interactions and controlling the amount of lectin clustering using mannose/hydroxyl-functionalized dendrimers. J. Am. Chem. Soc. 2003;125:8820-6. [PubMed ID: 12862477].
-
25.
Tabatabai SA, Rezaee Zavareh E, Reyhanfard H, Alinezhad B, Shafaghi B, Sheikhha M, Shafiee A, Faizi M. Evaluation of anxiolytic, sedative-hypnotic and amnesic effects of novel 2-phenoxy phenyl-1,3,4-oxadizole derivatives using experimental models. Iran. J. Pharm. Res. 2015;14:51-7.
-
26.
Obi K, Kojima A, Fukuda H, Hirai K. Synthesis and biological activity of a novel class of cephalosporins with a oxadiazolyl hydroxypyridone moiety at C-7. Bioorg. Med. Chem. Lett. 1995;5:2777-82.
-
27.
Dilmaghani KA, Jazani NH, Nasuhi Pur F, Shokoufeh N, Ghadiri F, Mirfakhraee F. Synthesis of 5-(4-aminophenyl)-2-(arylamino)-1, 3, 4-thiadiazoles and their schiff base derivatives as antimycobacterial agents. Chem. Heterocycl. Compd. 2012;48:362-7.
-
28.
Tabatabai SA, Lashkari SB, Zarrindast MR, Gholibeikian M, Shafiee A. Design, synthesis and anticonvulsant activity of 2-(2-phenoxy) phenyl-1,3,4-oxadiazole derivatives. Iran. J. Pharm. Res. 2013;12:105-11. [PubMed ID: 24250678].
-
29.
Dilmaghani KA, Nasuhi Pur F, Jazani NH, Alavi A, Niknam Z, Mirfakhraee F. Synthesis of new 1,2,4-triazole-5-thiones and their thioglycoside derivatives as potential antibacterial agents. Phosphorus, Sulfur, and Silicon. 2014;189:81-7.
-
30.
Dilmaghani KA, Nasuhi Pur F, Hatami Nezhad M. Synthesis and antibacterial evaluation of new thionesubstituted 1,2,4-triazole Schiff bases as novel antimicrobial agents. Iran. J. Pharm. Res. 2015;14:693-699. [PubMed ID: 26330857].
-
31.
Aydogan F, Turgut Z, Ocal N. Synthesis and electronic structure of new aryl- and alkyl-substituted 1,3,4-oxadiazole-2-thionederivatives. Turk. J. Chem. 2002;26:159-69.
-
32.
Ahmad A, Iqbal R, Akhtar H, Hag ZU, Duddeck H, Stefaniak L, Sitkowski J. Synthesis and structure determination of some oxadiazole-2-thione and triazole-3-thione galactosides. Nucleos. Nucleot. Nucl. 2001;20:1671-82.