Abstract
Keywords
Anticancer activity Thymoquinone Nanothymoquinone MTT assay MCF7 cells
Introduction
In recent years, The morbidity and mortality of cancer still reach a high plateau and is a major public health problem world wide (1,2).
Cancer is the major cause of human’s death because of high incidence and mortality. The identification of new cytotoxic drug with low side effects on immune system has developed as important area in new studies of pharmacology (3).
Many studies report that a heavy diet in fruits and vegetables lowers the incidence of cancer (4,5). Some fruits and vegetables are considered as the main anticancer foods, because of their abundant antioxidants such as phenols, vitamin C, vitamin E, beta-carotene and lipotene (6). It has been reported that various fruit and vegetable extracts are capable of inhibiting the protoasome activity and this inhibition is associated with tumor cell apoptosis (7). Thymoquinone (TQ) is the bioactive compound derived from black seed (Nigella sativa) oil. It is a spice that grows in the Mediterranean region and in Western Asian countries. In folklore medicine, the seed is reportedly associated with diverse therapeutic benefits to bronchial asthma, dysentery, headache, gastrointestinal problems, eczema, hypertension, and obesity. The therapeutic and chemopreventive potential of black seeds have been reported (8). TQ has been reported to exhibit antiproliferative effects on cell lines derived from breast, colon, ovary, larynx, lung, myeloblastic leukemia, and osteosarcoma. Chemosensitization by TQ is mostly limited to in-vitro studies, and it has potential in therapeutic strategy for cancer. The results favor efficacy and enhancement of therapeutic benefit against tumor cells resistant to therapy based on cellular targets that are molecular determinants for cancer cell survival and progression (9).
Drug overdose remains a common clinical problem across the globe, occurring both inadvertently and intentionally. Consequently, a broad range of non-specific therapies have been developed to approach this problem, ranging from cathartics to more invasive and sophisticated approaches. Nanoparticles have been developed for scavenging various drugs; indeed (10). Nanogels, sub-micron hydrogel nanoparticles, have highly desirable properties that may make them particularly suitable for such applications. Like hydrogels, nanogels have a three dimensional, internally cross-linked microstructure, swell in aqueous solvents to provide free volume for non-specific sorption, and can shrink and swell according to changes in the gel environment. Like nanoparticles, nanogels are injectable, have extremely high specific surface areas available for interaction with chemicals in the gel environment, and respond much faster to environmental stimuli. Based on these properties, nanogels have already attracted significant interest as sensors, rheological modifiers, optical devices, mechanical actuators, diagnostics supports, and drugdelivery vehicles, among other applications (11). The aims of the current study were: 1) the preparation of MA-chitosan nanogels loaded with Thymoquinone and 2) Comparison the effects of Thymoquinone and nanothymoquinone on the viability of human breast adenocarcinoma cell line. Cell cytotoxicity and viability was examined by the 3-(4,5-dimethylthiazol-2-yl)-2,5-diphenyltetrazolium bromide (MTT) and dye exclusion assay.
Experimental
Chemicals
Thymoquinone (2-isopropyl-5-methyl-1,4-benzoquinone), Myristic acid (Sigma Aldrich ≥ 99%, Mw = 228.37 g/mol), Dimethyl sulfoxide (DMSO, Sigma Aldrich, ≥ 99.9%, Mw = 78.13 g/mol), Chitosan(Sigma Aldrich, medium molecular weight of 340 g/mol) and EDC (1-ethyl-3-(3-dimethylaminopropyl)-carbodiimide Sigma Aldrich, Mw = 155.24 g/mol, d = 0.814 g/mL) were purchased from Sigma Chemical Co. (St. Louis, MO. USA).
Preparation of the nanogels
Nanogels were prepared by the technique of self-assembly with the method of Chen, et al. (12) with some modifications. Myristic acid (MA) solution was prepared by dissolving 228 mg MA in 23 mL Dimethyl sulfoxide. Chitosan (0.5 g) was dissolved in 1% acetic acid solution (100 mL). Polymer complexes of MA and chitosan was prepared by adding EDC cross linker. EDC was dissolved in methanol (126 µL/15 mL); then added to the MA solution. Subsequently, chitosan solution was added drop wisely to the MA solution while stirring under the hood at room temperature. MA was coupled to chitosan by the formation of amid linkages through the EDC-mediated reaction. The weight ratio of chitosan to MA and EDC were 3:1:1. The molecule oriented itself to exposed hydrophilic regions to the water and the hydrophobic regions assembled in the core; by this process micelle like nanogels are prepared. Dried nanogels were dissolved in 1% acetic acid solution and kept in refrigerator at -4 ˚C until required for the tests. Dionized water was applied for preparing all solutions.
Loading thymoquinone
Thymoquinone was loaded into the MA-chitosan nanogels. A variable ratio of Thymoquinone (0, 5, 50, 100, 200 and 300 µg/mL) was added to the nanogels while sonicating in water bath (Powersonic S10) with high-power ultrasound at room temperature for 30 min. Thymoquinone -loaded nanogels (TLNs) were characterized by Malvern Zetasizer (Malvern Instruments Ltd., United Kingdom) to determine median size of nanogels and size distribution when loaded with different amount of the Thymoquinone.
TEM and SEM study
Scanning and Transmission Electron Microscopy (SEM and TEM) was preformed to observe TLNs morphology, shape, size and core and shell structures. For preparing SEM photographs, one drop of TLN was dropped onto studs and gold sputtered using an auto sputter coater (BIORAD Polaron Division), then analyzed with Hitachi Field Emission Scanning Electron Microscope (FE-SEM) S-4160 (Japan) at 15 kV acceleration voltage. TEM was performed with the method of Liu et al. (13). By using Transmission Electron Microscope (Zeiss EM 10C TEM-GmbH) at an accelerating voltage of 80 Kv. The increasing amount of the Thymoquinone led to an increase in the particles sizes with irregular shape (Figure 1).
Cell culture and Thymoquinone treatment
MCF7 cell lines were obtained from the National Cell Bank of Iran (NCBI). The cells were cultured in RPMI-1640 containing 10% FBS, 2 mM glutamine, antibiotics (penicillin G, 60 mg/L; streptomycin, 100 mg/L; amphotericin B, 50 μL/L) under a humid atmosphere (37 °C, 5% CO2, 95% air).
Upon reaching appropriate confluence, the cells were passaged and incubated with 0(Control group), 5, 50, 100, 200 and 300 µg/mL concentrations of Thymoquinone and nanothymoquinone for defined times. The cells were treated with MA-chitosan nanogels at the same dose of that used in the maximum dose (300 microgram/mL) group too.
Determination of cell viability
The assay detects the reduction of MTT [3-(4,5-dimethylthiazolyl)-2,5-diphenyl-tetrazolium bromide] (Sigma) (a colorimetric technique) by mitochondrial dehydrogenase to blue formazan product, which reflects the normal function of mitochondria and hence the measurement of cytotoxicity and cell viability.
Briefly, l04 cells/well were treated with various concentrations of Thymoquinone and nanothymoquinone. After 24 hour incubation the cells were washed twice with phosphate buffered saline (PBS) and MTT (0.5 mg/mL PBS) was added to each well and incubated at 37 °C for 3 h. The formazan crystals that formed were dissolved by adding DMSO (100 μL/well), and the absorbance was read at 570 nm using a microplate scanning spectrophotometer (ELISA reader, Organon Teknika, Netherlands). Toxicity level was calculated by the following formula:
Viability % = 100 - Cytotoxicity %
To diminish test error level, MTT strain was added to some wells without cells and along with other wells, absorbance level was read and ultimately subtracted from whole the absorbance.
Statistical analysis
Statistical significances of difference throughout this study were calculated using a Student,s t-test and by one-way variance analysis. P<0.05 was considered statistically significant.
p-values (Statistically significant if<0.05) were determined using the Graph Pad Prism V.5. software.
Results
Surface morphology
The shape and surface texture of the nanoparticles could be detected using a number of sophisticated techniques such as TEM or SEM, respectively. Nanoparticles showed a round and smooth surface in TEM. The morphology of TQ-loaded MA-chitosan nanogels as prepared is shown in Figure 1(A and B). Nanoparticle size was determined by TEM, which proved its sphericity. The particle size ranged between 150 and 200 nm (Figure 1A). The SEM of Nanoparticles proved their smooth surface texture (Figure 1B).
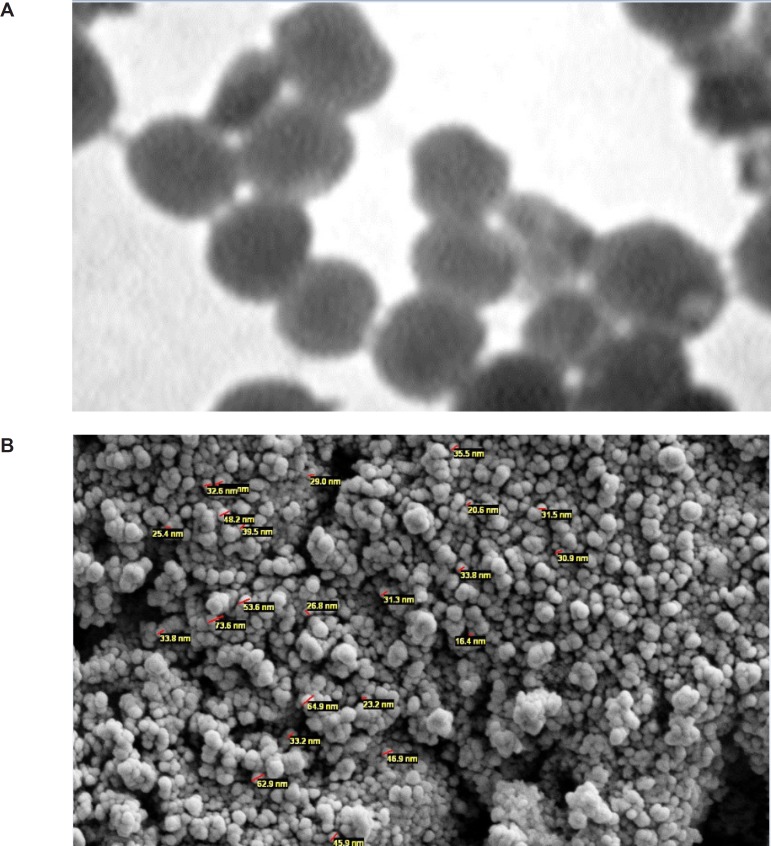
The effects of Thymoquinone and nanothymoquinone on inhibition and proliferation of MCF7 cell line
The effect of Thymoquinone and nanothymoquinone were studied as a dose-response experiment. Proliferation of MCF7 cells was significantly inhibited by Thymoquinone and nanothymoquinone in a concentration-dependent manner in 24 h. (P<0.05) Different concentrations of Thymoquinone and nanothymoquinone at 24 h had different cytotoxicity effects on MCF7 cell line ().
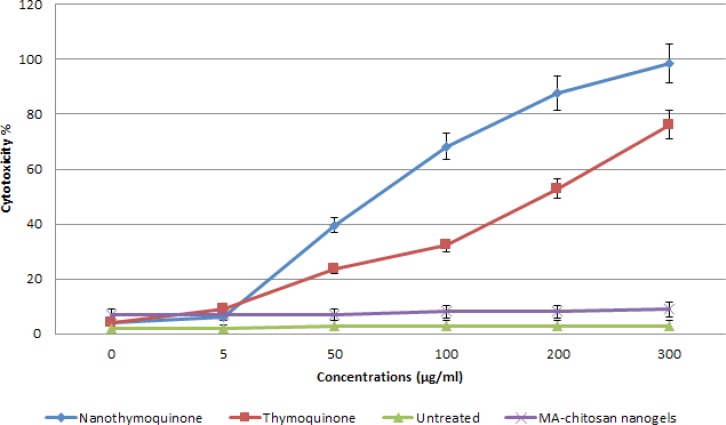
The 50% inhibition concentration (IC50) values of Thymoquinone and nanothymoquinone on MCF7 cells were determined (Figure 3). IC50 was determined by probit analysis using the Pharm PCS (Pharmacologic Calculation System) statistical package (Springer- Verlag, USA). There were significant differences in IC50 Thymoquinone and nanothymoquinone (P<0.05).
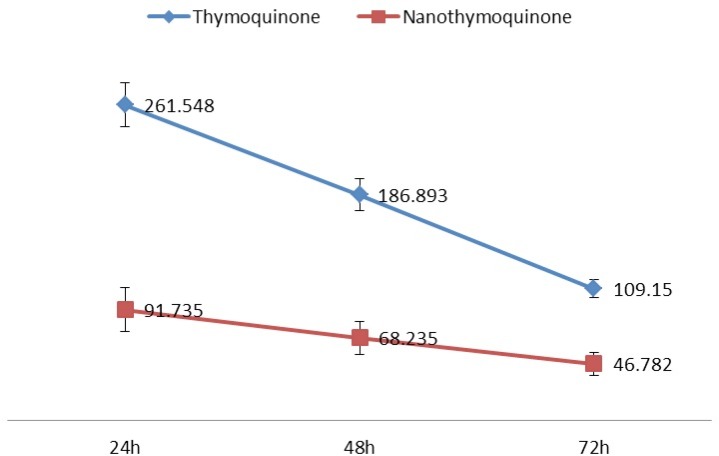
Discussion
Cancer drug resistance has been reported to develop following treatment. Therefore, there is a need to explore into new anti-cancer drugs. There has been increasing literature on medicinal properties of thymoquinone over the years. Preclinical studies reveal the potential of TQ in improving the therapeutic effect of anticancer drugs and also protection of nontumor tissues against chemotherapy-induced damages. For example, TQ ameliorated nephrotoxicity and cardiotoxicity by cisplatin, ifosfamide, and doxorubicin (14-16). However, no Phase I pilot study has so far been reported evaluating its promising anticancer effect in human subjects. Of interest, Gali-Muhtasib et al. (17) noted that TQ induces a sharp increase in p16 protein levels within 2 h of treatment; this observation is of interest, since as mentioned by Gali-Muhtasib et al. (18) and Hochhauser (19), modulation of p16 protein expression increases tumor sensitivity to chemotherapeutic drugs.
Bioavailability of a drug to the cells, whether in-vitro or in-vivo, is critical for its optimal efficacy. Physical entrapment of bioactive molecules such as drugs, proteins, carbohydrates, and DNA in the polymeric network, as well as their in-vitro release behavior, have been extensively investigated as targeted drug delivery carriers for biomedical applications (20-23). To enhance the solubility of drugs in aqueous solvents, increase their bioavailability, enhance serum half-life, for tumor cell targeting and bio imaging, nanotechnology has recently emerged as a new technology of choice. Conjugations of drugs with lipids, carbohydrates, and proteins have been used to make nanoparticles. Recent studies from our laboratory have shown that enhanced uptake of cur cumin nanoparticles correlates with enhanced antitumor effects (20). TQ is the bioactive compound, but its poor solubility in aqueous medium and relative toxicity affect its bio-availability and administration in human.
Of particular relevance to pathophysiology of tumor growth is proliferation of tumor cells. Thus far, TQ has been shown to inhibit cell proliferation in cultured cells derived from human breast and ovarian adenocarcinoma, myeloblastic leukemia cells, HL-60, squamous carcinoma [SCCVII;], fibro sarcoma [FSSaR;)], laryngeal neoplastic cells-Hep-2, and prostate and pancreatic cancer (PC) cell lines. Interestingly, human pancreatic ductal epithelial cells (HPDE) cells, noncancerous (BPH-1) prostate epithelial cells, normal kidney cells, and normal human osteoblasts are relatively resistant to inhibitory effect of TQ as reported by us and others (9).
In the present investigation, TQ-encapsulated Chitosan nanoparticles were prepared successfully. We prepared Thymoquinone -loaded nanogels to enhance solubility and investigate their ability to inhibit the proliferation of breast cancer cells in-vitro. When examined for its ability to suppress the growth of cancer cells, we found that Thymoquinone -loaded nanogels was at least twice as potent as free TQ, possibly due to enhanced uptake. It comprised investigation into extended treatment duration and observation on molecular processes. The initial stage involved study on breast cancer cell proliferation and exposure to wide range of Thymoquinone and nanothymoquinone concentrations. The long-term treatment with thymoquinone was studied for 72 h in breast cancer cell line MCF-7. Further analysis on cell cycle was previously carried out for prolonged treatment of 72 h (21). Thymoquinone was found to inhibit molecules which are involved in the S phase with 25 μM thymoquinone concentration. Furthermore, sub-G1 phase arrest occurred with either 25 μM when treated for 12 h. It was previously reported more than 60 μM thymoquinone led to sub-G1 arrest within 12–48 h. This indicated cell growth inhibition could be sustained with minimal dose 25 μM thymoquinone in an extended duration of treatment (22). Cells were found to be more sensitized following 72 h. The proliferation of MCF-7 cell line was regulated by dose of thymoquinone and in overall 50% cell death occured with 186.89 μg/mL and 68.24 μg/mL with 48 h treatment for Thymoquinone and nanothymoquinone respectively. The design and preparation of nanogels have attracted a great deal of interest in biomedical engineering, pharmaceutical applications, and biomaterials science because of their tunable chemical and three-dimensional (3D) physical structure, good mechanical properties, high water content, and biocompatibility. These unique properties offer great potential for the utilization of nanogels in tissue engineering, biomedical implants, drug delivery, and bionanotechnology (23).
A variety of polysaccharide–based nanogels have been subjected to intensive research in order to address the concerns related to the spontaneous self assembly behaviours of the nanogels in aqueous media. All the tested nanogels have clearly presented adequate cell viability and non-toxicity (23). These nanogels have high content of functional group, compatibility, adjustable size, low immunogenicity and large surface area for multivalent bio-conjugation. Furthermore, their abundance in nature, renewability, nontoxicity and low manufacturing cost make them unique candidates for biotechnology and pharmaceutics applications. Chitosan myristic acid nanogel is used as a support for the organophosphorus hydrolase which degrade organophosphorus pesticides. Acting as an inhibitor of acetylcholinestrase, organophosphorus compounds pose important hazards to the human and environment while they are used as effective pesticides and insecticides. Growing usage of organophosphorus compounds in agriculture and manufacture of nerve agents has stimulated introduction of stabilization methods for degradative enzymes such as organophosphorus hydrolase (24). Gupta et al. recently reported chitosan polymers blended non-covalently to encapsulate curcumin using the capillary-microdot technique (25). These curcumin nanoparticles showed higher efficacy against breast cancer cells and had the potential to treat breast tumors by local, sustained, and long-term therapeutic delivery as a biodegradable system. Curcumin can block TNF-action and production in in-vitro models (26). (TNF)-α stimulates the production of bcl-2 family member protein from the cytoplasm to the outer mitochondrial membrane (27,28).
This is the first study that describes the encapsulation of TQ in chitosan myristic acid nanogel. It could be concluded that these nanogels are effective at low dose rates, with controlled and effective release and could be applied as an anticancer strategy.
Acknowledgements
References
-
1.
Nicholson DW. Caspase structure, proteolytic substrates, and function during apoptotic cell death. Cell Death Differ. 1999;6:1028-1042. [PubMed ID: 10578171].
-
2.
Mao TK, Van De Water J, Keen CL, Schmitz HH, Gershwin ME. Cocoa flavonols and procyanidins promote transforming growth factor-beta1 homeostasis in peripheral blood mononuclear cells. Exp. Biol. Med. 2003;228:93-99.
-
3.
Cragg GM, Newman DJ. Plants as a source of anticancer agents. J. Ethnopharmacol. 2005;100:72-79. [PubMed ID: 16009521].
-
4.
Chen MS, Chen D, Dou QP. Inhibition of proteasome activity by various fruits and vegetables is associated with cancer cell death. In-vivo. 2004;1:73-80. [PubMed ID: 15011755].
-
5.
Harborne JB, Williams CA. Anthocyanins and other flavonoids. Nat. Prod. Rep. 2001;18:310-333. [PubMed ID: 11476484].
-
6.
Ghasemian A, Mehrabian S, Majd A. Peel extracts of two Iranian cultivars of Pomegranate(punica granatum) have antioxidant and antimutagenic activities. Pakistan J. Biol. Sci. 2006;7:1402-1405.
-
7.
Traub ML, De Butte-Smith M, Zukin RS, Etgen AM. Oestradiol and insulin-like growth factor-1 reduce cell loss after global ischaemia in middle-aged female rats. J. Neuroendocrinol. 2009;21:1038-1044. [PubMed ID: 19840235].
-
8.
Padhye S, Banerjee S, Ahmad A, Mohammad R, Sarkar FH. From here to eternity the secret of pharaohs: therapeutic potential of black cumin seeds and beyond. Cancer Ther. 2008;6:495-510. [PubMed ID: 19018291].
-
9.
Banerjee S, Padhye S, Azmi A, Wang Z, Philip PA, Kucuk O, Sarkar FH, Mohammad RM. Review on molecular and therapeutic potential of thymoquinone in cancer. Nutr.Cancer. 2010;62:938-946. [PubMed ID: 20924969].
-
10.
Weinberg GL, Vade Boncouer T, Ramaraju GA, Garcia-Amaro MF, Cwik MJ. Pretreatment or resuscitation with a lipid infusion shifts the dose response to bupivacaine induced asystole in rats. Anesthesiol. 1998;88:1071-1075.
-
11.
Hoare T, Sivakumaran D, Stefanescu CF, Lawlor MW and Kohane DS. Nanogel scavengers for drugs: local anesthetic uptake by thermoresponsive nanogels. Acta Biomater. 2012;8:1450-1458. [PubMed ID: 22244983].
-
12.
Chen XG, Lee CM, Park HJ. O/W emulsification for the selfaggregation and nanoparticle formation of linoleic acid-modified chitosan in the aqueous system. J. Agric. Food Chem. 2003;51:3135-3139. [PubMed ID: 12720404].
-
13.
Liu CG, Desai KG, Chen XG, Park HJ. Linolenic acid-modified chitosan for formation of self-assembled nanoparticles. J. Agric. Food Chem. 2005;53:437-441. [PubMed ID: 15656685].
-
14.
Badary OA, Nagi MN, al-Shabanah OA, al-Sawaf HA, al-Sohaibani MO, al-Bekairi AM. Thymoquinone ameliorates the nephrotoxicity induced by cisplatin in rodents and potentiates its antitumor activity. Can. J. Physiol Pharmacol. 1997;75:1356-1361. [PubMed ID: 9534946].
-
15.
al-Shabanah OA, Badary OA, Nagi MN, al-Gharably NM, al-Rikabi AC, al-Bekairi AM. Thymoquinone protects against doxorubicin-induced cardiotoxicity without compromising its antitumor activity. J. Exp. Clin. Cancer Res. 1998;17:193-198. [PubMed ID: 9700580].
-
16.
Badary OA, Abdel-Naim AB, Abdel-Wahab MH, Hamada FM. The influence of thymoquinone on doxorubicin-induced hyperlipidemic nephropathy in rats. Toxicol. 2000;143:219-226.
-
17.
Gali-Muhtasib HU, Abou Kheir WG, Kheir LA, Darwiche N, Crooks PA. Molecular pathway for thymoquinone-induced cell-cycle arrest and apoptosis in neoplastic keratinocytes. Anticancer Drugs. 2004;15:389-399. [PubMed ID: 15057144].
-
18.
Gali-Muhtasib H, Roessner A, Schneider-Stock R. Thymoquinone: a promising anti-cancer drug from natural sources. Int. J. Biochem. Cell Biol. 2006;38:1249-1253. [PubMed ID: 16314136].
-
19.
Hochhauser D. Modulation of chemosensitivity through altered expression of cell cycle regulatory genes in cancer. Anticancer Drugs. 1997;8:903-910. [PubMed ID: 9436633].
-
20.
Ravindran J, Nair HB, Sung B, Prasad S, Tekmal RR, Aggarwal BB. Thymoquinone poly (lactide-co-glycolide) nanoparticles exhibit enhanced anti-proliferative, anti-inflammatory, and chemosensitization potential. Biochem. Pharmacol. (2010;79:1640-1647. [PubMed ID: 20105430].
-
21.
Shoieb AM, Elgayyar M, Dudrick PS, Bell JL, Tithof PK. In-vitro inhibition of growth and induction of apoptosis in cancer cell lines by thymoquinone. Int. J. Oncol. 2003;22:107-113. [PubMed ID: 12469192].
-
22.
Motaghed M, Al-Hassan FM, Hamid SS. Cellular responses with thymoquinone treatment in human breast cancer cell line MCF-7. Pharmaco. Res. 2013;5:200-206.
-
23.
Oh JK, Drumright R, Siegwart DJ, Matyjaszewski K. The development of microgels/ nanogels for drug delivery applications. Prog. Polym. Sci. 2008;33:448-477.
-
24.
Oh JK, Lee DI, Park JM. Biopolymer- based microgels/ nanogels for drug delivery applications. Progress in Polymer Sci. 2009;34:1261-1282.
-
25.
Gupta V, Aseh A, Rios CN, Aggarwal BB, Mathur AB. Fabrication and characterization of silk fibroin-derived curcumin nanoparticles for cancer therapy. Int. J. Nanomed. 2009;4:115-122.
-
26.
Aggarwal BB, Gupta SC, Sung B. Curcumin: an orally bioavailable blocker of TNF and other pro-inflammatory biomarkers. Br. J. Pharmacol. 2013;169:1672-1692. [PubMed ID: 23425071].
-
27.
Hashemi M. The study of pentoxifylline drug effects on renal apoptosis and BCL-2 gene expression changes following ischemic reperfusion injury in rat. Iran. J. Pharm. Res. 2014;13:181-189. [PubMed ID: 24734070].
-
28.
Khazaei Koohpar Z, Hashemi M, Mahdian R, Parivar K. The effect of pentoxifylline on bcl-2 gene expression changes in hippocampus after long-term use of ecstasy in wistar rats. Iran. J. Pharm. Res. 2013;12:521-527. [PubMed ID: 24250658].