Abstract
Keywords
Acetaminophen Paracetamol Antidepressant drugs Drug-drug interaction Cyclic voltammetric
Introduction
As pharmaceutical technology continues to expand at a phenomenal rate, so does the incidence of drug interactions. A clinically relevant drug-drug interaction (DDI) occurs when the effectiveness or toxicity of one medication is altered by the administration of another medicine or a substance that is administered for medical purposes. Adverse consequences of DDIs may result from either diminished therapeutic effect or toxicity. The two drugs need not physically interact with each other to produce the effect. When the drug combination results in an undesired effect, the drug interaction becomes an adverse drug interaction. Drug interactions are much more common than adverse drug interactions (1-10). In one of the commonest treatment cases, acetaminophen (paracetamol) (1) is used with antidepressant drugs (2a-c) (Figure 1), so the study of the interaction between these drugs can be important both pharmaceutically and therapeutically.
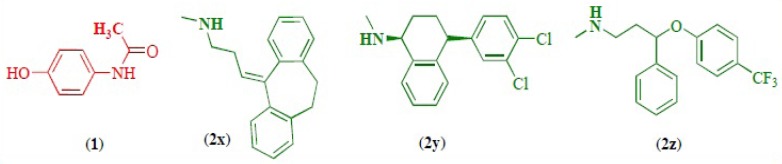
It has been shown that N-acetyl-p-benzoquinone-imine (NAPQI) is the main in-vivo and in-vitro oxidation product of acetaminophen (11). Our previous studies show that the electrochemically generated NAPQI is a reactive intermediate and as a Michael acceptor, participates in different types of reactions (11-16). On the other hand, a literature survey shows that acetaminophen is metabolized by cytochrome P450 2E1 to the reactive metabolite NAPQI (11). Since the electrochemical oxidation parallels the cytochrome P450-catalysed oxidation in liver microsomes, it was interesting to study the anodic oxidation of acetaminophen in the presence of antidepressant drugs (2x-z). In this work we would like to report a simple electrochemical procedure on the drug-drug interaction between acetaminophen and antidepressant drugs (Figure 1) and synthesis a new product of electro oxidation of acetaminophen in the presence of nortryptline (2x) at biological pH. Moreover the observed homogeneous rate constants
Experimental
Apparatus
Cyclic voltammetry was performed using a SAMA500 potentiostat/galvanostat. Controlled-potential coulometry was performed using a potentiostat/galvanostat system model BHP 2061-C. The working electrode used in the voltammetry experiments was a glassy carbon disc (1.8 mm2 area) and a platinum wire was used as the counter electrode. The working electrode used in controlled-potential coulometry and macroscale electrolysis was an assembly of four carbon rods (31 cm2) and large platinum gauze constituted the counter electrode. The working electrode potentials were measured versus SCE (all electrodes from AZAR Electrode Co. Iran). Experimental conditions were as reported in our earlier paper (17). The homogeneous rate constants were estimated by analysing the cyclic voltammetric responses using cyclic voltammogram digital simulation software (DigiElch SB) version 2.0 (18).
Reagents
Acetaminophen (1) was reagent grade material from Aldrich. nortriptyline (2x) and serteralin (2y) were obtained from Rouz Darou Pharmaceutical Co. and fluxetine (2z) was obtained from Jalinous Pharmaceutical CO. All of solvents were of pro-analysis grade from E. Merck. These chemicals were used without further purification.
Electroorganic Synthesis of 6
In a typical procedure, 60 mL of phosphate buffer solution (0.2 M, pH 7.0) in water/acetonitrile (70/30), containing acetaminophen (1) (0.5 mmol) and nortriptyline (2a) (0.5 mmol) was electrolyzed at 0.30 V versus the SCE in a two-compartment cell. The electrolysis was terminated when the current decayed to 5% of its original value. At the end of electrolysis the precipitated solid was collected by filtration and was washed several times with water. After washing, product was characterized by IR, and MS.
Characterization data
Mp. 250-252 ◦C (Dec.). IR (KBr): 3435.8, 3016.7, 2769.6, 2426.9, 1613.8, 1485.5, 1384.4, 1161.72, 1029.5, 777.56, 756.4, 594.9 cm-1 MS: m/z (relative intensity) = 495 (M, 3), 494 (6), 369 (6), 319 (5), 263 (21), 219 (64), 202 (100), 178 (40), 152 (61), 91 (50), 57 (74).
Results and Discussion
Voltammetric studies
Cyclic voltammogram of 1.0 mM of acetaminophen (1) in aqueous solution containing 0.2 M phosphate buffer at pH 7 shows one anodic (A1) and corresponding cathodic peak (C1), corresponding to the transformation of acetaminophen to N-acetyl-p-benzoquinone-imine (NAPQI) and vice versa within a quasi-reversible tow-electron process (Figure 2, curve a). (13-15) A peak current ratio
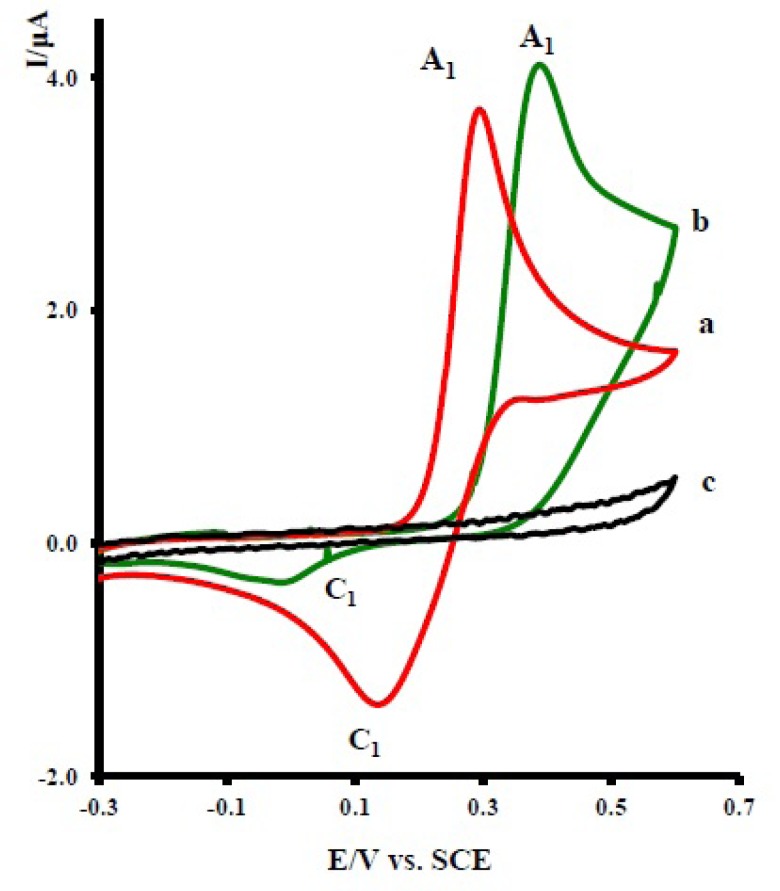
The oxidation of 1 in the presence of 10 mM of nortriptyline (2x) was studied in some detail. Figure 2 curve b, shows the cyclic voltammogram of 1 in the presence of 2x. The voltammogram exhibits one anodic peak (A1) and its cathodic counterpart (C1). The comparison of peak C1 in the absence and presence of 2x shows a decrease in the current for the later. The observed shift of the A1 peak in curve b, relative to curve a, is probably due to the formation of a thin film of product at the surface of the electrode, inhibiting to a certain extent the performance of the electrode process. In this figure, curve c is the voltammogram of 2x in the same condition and in the absence of 1.
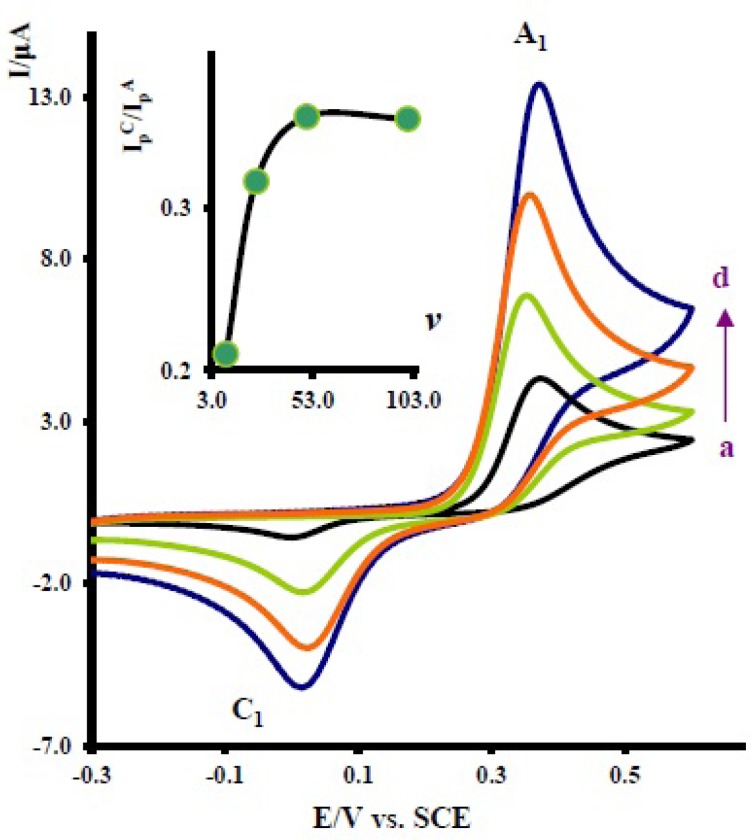
The effect of scan rate on the electrochemical behavior of 1 in the presence of 2x was also studied. Figure 3 shows the typical cyclic voltammograms obtained for 1 mM of 1 in the presence of 10 mM nortriptyline (2x) at various potential scan rates. As can be seen, with increasing scan rate, the peak current ratio,
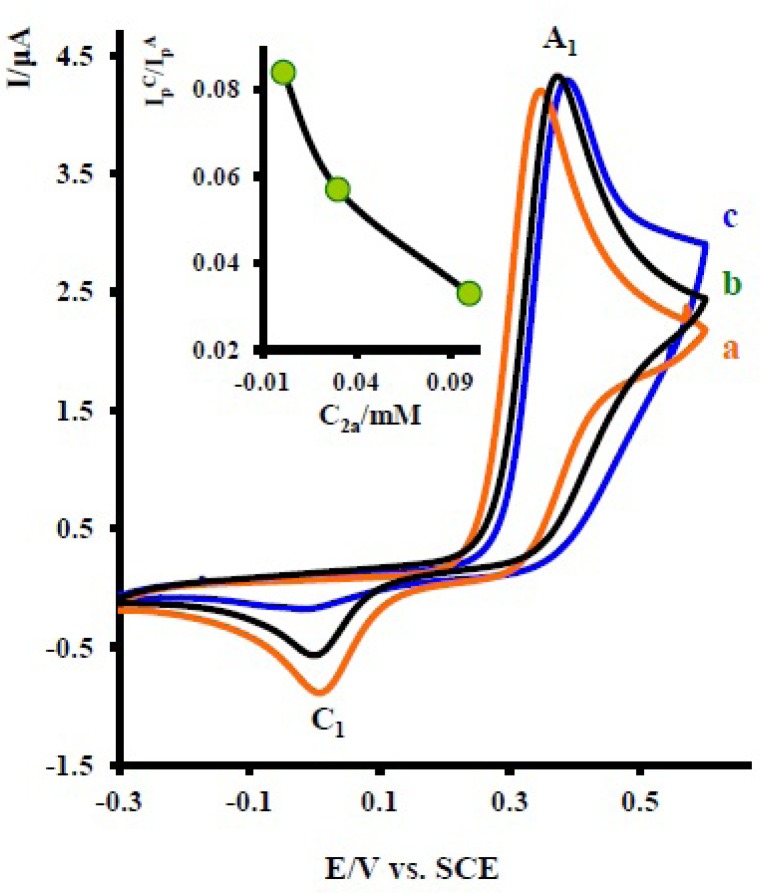
The existence of a subsequent chemical reaction between NAPQI and 2x is supported by the following evidences: (a) decrease in
Controlled-potential coulometry was performed in aqueous solution containing 0.1 mmol of 1 and 0.1 mmol of 2x at 0.30 V versus saturated calomel electrode (SCE). The electrolysis progress was monitored using cyclic voltammetry (Figure 5). It is shown that, proportional to the advancement of coulometry, the anodic peak A1 decreases and a new anodic peak (A0) appears. The anodic peak A0 is probably related to the oxidation of an intermediate that is produced in the electrolysis cell (7a or 9a in Scheme 1). Anodic and cathodic peakas (A1/C1 and A0/C0) disappear when the charge consumption becomes about 6e- per molecule of 1.
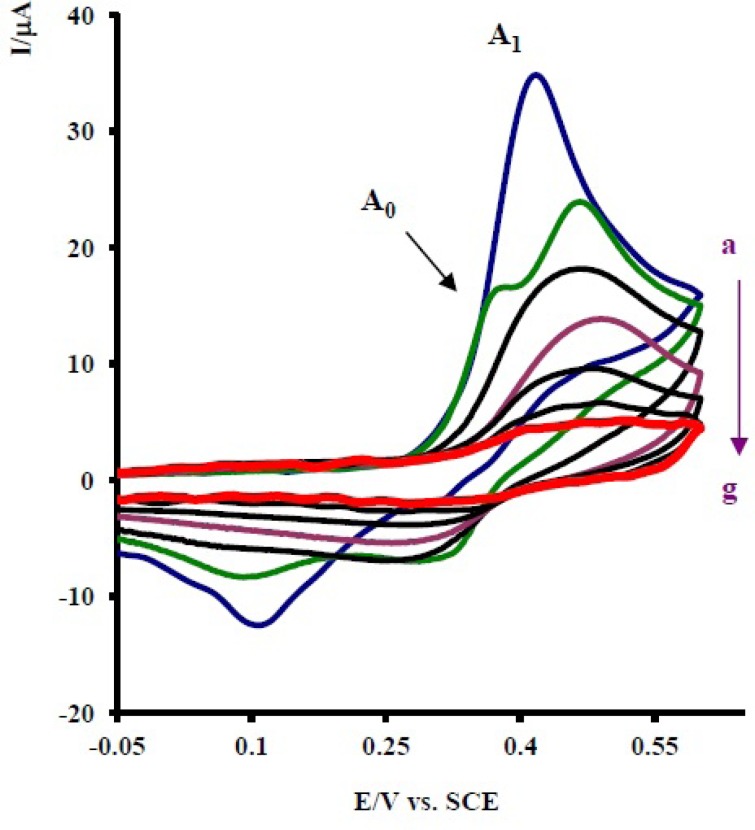
Electrochemical and spectroscopic data in accompanied by previously published data (12-15) allow us to propose the mechanism presented in Scheme 1 for the electrooxidation of acetaminophen (1) in the presence of 2x-z. It seems that because of the good reactivity (decrease in
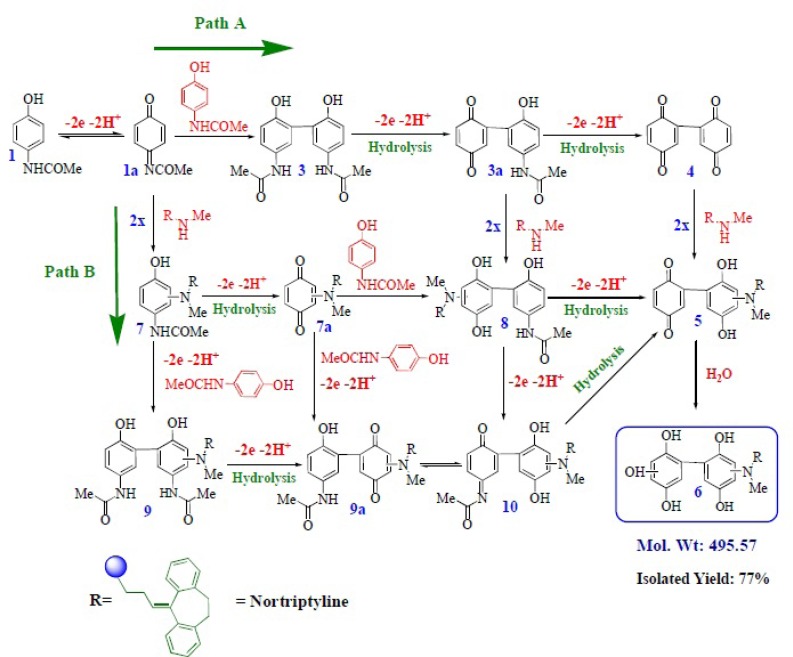
The proposed mechanism was established by using Ms and IR technique. In the mass spectrum of compound 6, molecular ion [M + 1H] was recorded. This mass is related to protonation of the nortriptyline (2x) group (20-22) and is another proof for production of 6 in electrooxidation of 1 in the presence of 2x. The electrochemical oxidation of acetaminophen (1) in the presence of sertraline (2y) and fluoxetine (2z) was also investigated (Figure 6). The same behavior has been seen for the electrochemical oxidation of 1 in the presence of 2y, z.
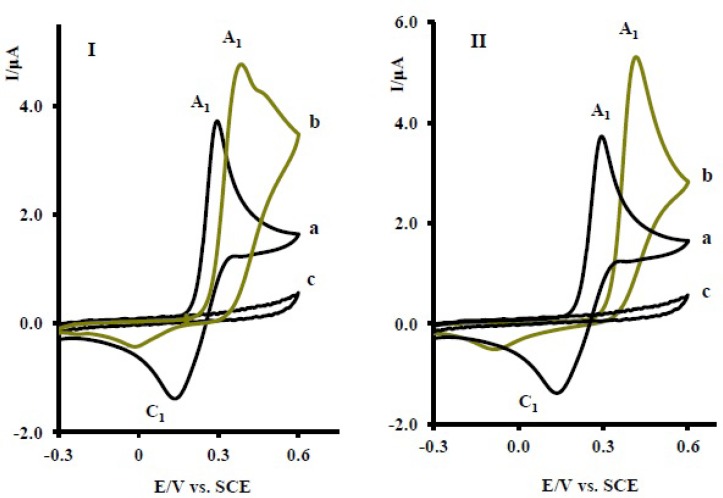
Simulation
Electrochemical oxidation of acetaminophen (1) in various pHs was tested by digital simulation. The simulation was carried out assuming semi-infinite one-dimensional diffusion and planar electrode geometry (14). The experimental parameters entered for digital simulation consisted of the following: Estart, Eswitch, Eend, t=25◦C and analytical concentration of acetaminophen (1). The transfer coefficient (α) was assumed to be 0.5 and the formal potentials were obtained experimentally as the mid point potential between the anodic and cathodic peaks (Emid). The heterogeneous rate constant (0.002cms−1) for oxidation of acetaminophen (1) was estimated by use of an experimental working curve (14,23). All these parameters were kept constant through out the fitting of the digitally simulated voltammogram to the experimental data. The parameter
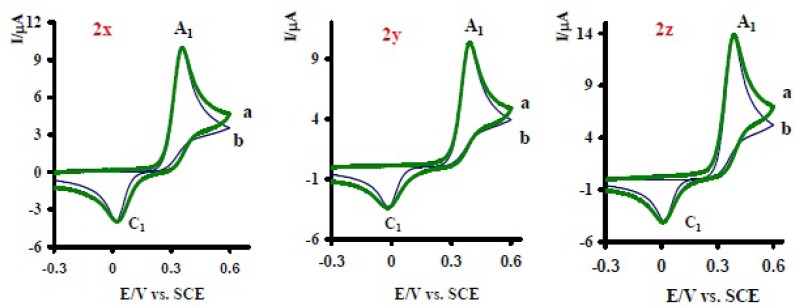
Observed homogeneous rate costants, kobs(s-1), for the studied acetaminophen in the presence of nortriptyline (2x), sertraline (2y) and fluoxetine (2z).
Conclusions
This work presents sound electrochemical data for the reaction of N-acetyl-p-benzoquinone-imine (NAPQI) derived from the oxidation of acetaminophen (1) with antidepressant drugs (2x-z). This reaction (see scheme 1), reduces the concentration of NAPQI and decreases the effective concentration of antidepressants. In addition, this work introduces electrochemistry as a "powerful tool" to accomplish diagnostic tests in medical and pharmaceutical sciences (25-28) and the synthesis of new organic compounds.
Acknowledgements
References
-
1.
pina E, Santoro V, D'Arrigo C. Clinically relevant pharmacokinetic drug interactions with second-generation antidepressants: an update. Clin Ther. 2008;30:1206-1227. [PubMed ID: 18691982].
-
2.
Dresser GK, Bailey DG. A basic conceptual and practical overview of interactions with highly prescribed drugs. Can J Clin Pharmacol. 2002;9:191-198. [PubMed ID: 12584577].
-
3.
Nematollahi D, Nasseri Y, Amani A, Fatemi F. Kinetic and mechanistic study of drug–drug interaction between acetaminophen and β-lactam antibiotics. Prog React Kinet Mec. 2013;38:213-219.
-
4.
Malone DC, Hutchins DS, Haupert H, Hansten P, Duncan B, Van Bergen RC, Solomon SL, Lipton RB. Assessment of potential drug-drug interactions with a prescription claims database. Am J Health Syst Pharm. 2005;62:1983-1991. [PubMed ID: 16174833].
-
5.
Hansten PD, Horn JR, Hazlet TK. ORCA: Operational classificAtion of drug interactions. J Am Pharm Assoc (Wash). 2001;41:161-165. [PubMed ID: 11297327].
-
6.
Baker GB, Fang J, Sinha S, Coutts RT. Metabolic drug interactions with selective serotonin reuptake inhibitor (SSRI) antidepressants. Neurosci Biobehav R. 1998;22:325-333.
-
7.
Wallerstedt SM, Gleerup H, Sundstrom A, Stigendal L, Ny L. Risk of clinically relevant bleeding in warfarin-treated patients--influence of SSRI treatment. Pharmacoepidemiol Drug Saf. 2009;18:412-416. [PubMed ID: 19301238].
-
8.
Hauta-Aho M, Tirkkonen T, Vahlberg T, Laine K. The effect of drug interactions on bleeding risk associated with warfarin therapy in hospitalized patients. Ann. Med. 2009;41:619-628. [PubMed ID: 19711211].
-
9.
Schelleman H, Brensinger CM, Bilker WB, Hennessy S. Antidepressant-warfarin interaction and associated gastrointestinal bleeding risk in a case-control study. Plos One. 2011;6:e21447. [PubMed ID: 21731754].
-
10.
Tavakoli-Ardakani M, Kazemian K, salamzadeh J, Mehdizadeh M. Potential of drug interactions among hospitalized cancer patients in a developing country. Iran. J. Phram. Res. 2013;12:175-182.
-
11.
Kennon J, Heard MD. Acetylcysteine for acetaminophen poisoning. N. Engl. J. Med. 2008;359:285-292. [PubMed ID: 18635433].
-
12.
Miner DJ, Rice JR, Riggin RM, Kissinger PT. Voltammetry of acetaminophen and its metabolites. Anal. Chem. 1981;3:2258-2263.
-
13.
Shafiei H, Haqgu M, Nematollahi D, Gholami MR. An experimental and computational study on the rate constant of electrochemically generated N-Acetyl-p-Quinoneimine with dimethylamine. Int. J. Electrochem. Sci. 2008;3:1092-1107.
-
14.
Nematollahi D, Shayani-Jam H, Alimoradi M, Niroomand S. Electrochemical oxidation of acetaminophen in aqueous solutions: Kinetic evaluation of hydrolysis, hydroxylation and dimerization processes. Electrochim. Acta. 2009;54:7407-7415.
-
15.
Shayani-Jam H, Nematollahi D. Electrochemical evidences in oxidation of acetaminophen in the presence of glutathione and N-acetylcysteine. Chem. Commun. 2010;46:409-411.
-
16.
Forouzandeh H, Azemi MA, Rashidi I, Goudarzi M, Kalantari H. Study of the protective effect of teucrium polium L. Extract on acetaminophen-induced hepatotoxicity in Mice. Iran. J. Phram. Res. 2013;12:123-129.
-
17.
Amani A, Khazalpour S, Nematollahi D. Electrochemical oxidation of acetaminophen and 4-(Piperazin-1-yl)phenols in the presence of 4-Hydroxy-1-methyl-2(1H)-quinolone. J. Electrochem. Soc. 2013;160:H33-H40.
-
18.
Rudolph M. Digital simulations on unequally spaced grids. Part 1. Critical remarks on using the point method by discretisation on a transformed grid. J. Electroanal. Chem. 2002;529:97-108.
-
19.
Bard AJ, Faulkner LR. Electrochemical Methods. 2nd. New York: Wiley; 2001.
-
20.
Bittner S, Meenakshi C, Temtsin G. N,N-bis(quinonyl)amines; synthesis and X-ray structure. Tetrahedron. 2001;57:7423-7429.
-
21.
Pachatouridis C, Couladouros EA, Papageorgiou VP, Liakopoulou-Kyriakides M. Derivatives of aminoquinones with N- protected amino acids. Lett. Pept. Sci. 1998;5:259-262.
-
22.
Nematollahi D, Hesari M. Electrochemical synthesis of amino-substituted 1,2-benzoquinone derivatives. J. Electroanal. Chem. 2005;577:197-203.
-
23.
Greef R, Peat R, Peter LM, Pletcher D, Robinson J. Instrumental Methods in Electrochemistry. New York; 1990.
-
24.
Nematollahi D, Mohammadi-Behzad L, Hossainy Davarani SS. Kinetic study of the oxidation of catechols in the presence of some aza-crown ethers by digital simulation of cyclic voltammograms. Electroanal. 2009;21:1099-1106.
-
25.
Qin X, Ai-ju Y, Rui Zh, Xiaojun B, Da Chen, Xiaoya H. Application of Electrochemical Methods for Pharmaceutical and Drug Analysis. Current Pharmaceutical. Analysis. 2009;5:144-155.
-
26.
Lunte CE, Osbourn D. Electrochemical Detection for Pharmaceutical Analysis. Encyclopedia of Pharmaceutical Technology. Third Edition. 2006.
-
27.
Petro R, Schlesinger M. Applications of Electrochemistry in Medicine. Springer; 2013.
-
28.
Omar MA, Abdelmageed OH, Abdelgaber AA, Saleh Safaa F. Assay of amlodipine besylate in tablets and human biological fluids by square wave adsorptive cathodic stripping voltammetry. Int. Res. J. Pure. Appl. Chem. 2013;3:133-146.