Abstract
Keywords
Introduction
Propofol (2, 6-diisopropylphenol) is a widely used intravenous sedative-hypnotic agent for both induction/maintenance of anesthesia and sedation of critically ill patients (1) Advantages of this agent over others of similar applications include lower incidence of side effects (2) and better quality of anesthesia (3). Propofol's structure contains a phenolic hydroxyl group and thus resembles that of a-tocopherol (vitamin E), a natural anti-oxidant. As shown by both in-vitro and in-vivo studies, the anti-oxidant activity of propofol results partly from this phenolic chemical structure (4). Numerous studies have demonstrated anti-oxidant effects of propofol in-vitro (5, 6) and in-vivo (7), but actions of propofol on different cells are varied and multiple mechanisms may be involved (8). Propofol has been demonstrated to prevent oxidative stress-mediated endothelial cell activation and dysfunction induced by hydrogen peroxide (8, 9) and tumour necrosis factor-a (TNF-a) (10, 11). The anti-oxidant status of a cell determines its susceptibility to oxidative damage, and is usually altered in response to oxidative stress (12). When reactive oxygen species (ROS) generation overwhelms the anti-oxidant defense, the free radicals can interact with endogenous macromolecules and alter cellular function (12). The mitochondrial respiratory chain is the major source of intracellular generation of ROS and at the same time, an important target for the damaging effects of ROS (13).
Carbon tetrachloride (CCl4) is known to induce reactive free radicals and induction of cell damage through covalent binding to the membrane proteins (14).
CCl4 is converted in to trichloromethyl radical (CCl3•) and its derivative peroxy trichloromethyl radical (•OOCCl3) by cytochrome P450 in liver microsomes. These free radicals are highly reactive and are capable of reacting with polyunsaturated fatty acids of the membranous system leading to oxidative injuries such as lipid peroxidation (15). The aims of this study were to investigate the anti-oxidative effects of propofol on liver mitochondrial function in rat treated with CCl4.
Experimental
Chemicals
Tetraethoxypropane (MDA), 2-thiobarbituric acid (TBA), trichloroace tic acid (TCA), n-butanol, propofol , sucrose, ethylenediamine tetra acetic acid (EDTA), Comassie blue, bovine serum album in (BSA), 4,5(dimethylthiazol-2-yl)-2,5-diphenyltetrazolium bromide (MTT), GPx and SOD (Ransel kit, Randox Laboratories Ltd, Crumlin, UK), bioxytech GSH kit (Oxis Research, USA), Comassie blue, bovine serum albumin (BSA), 4,5-(dimethylthiazol-2-yl)-2,5-diphenyltetrazolium bromide (MTT), were used in this study. All other chemicals were obtained from the Sigma.
Animals and treatments
Adult male Wistar rats weighing 180–250 g maintained on a 12-hour light/dark cycle with free access to tap water and standard laboratory chow were used. Animals were randomly divided into six groups (n=5) and treated for 2 week intraperitoneally (IP). The groups were as follows: control group, propofol group, CCl4 group, propofol and CCl4 group, vitamin E group, and CCl4 and vitamin E group.
Propofol was administered (10 mg/Kg/day, IP) alone or in combination with CCl4 (0.2 mL/Kg /day, IP) and vitamin E as (15 mg/Kg/day, SC) One group of animals received only normal saline and was assigned as control. At the end of the treatment, 24 hours post the last dose of treatment, animals were killed, liver tissue was separated and stored in liquid nitrogen. Blood samples were collected from heart in heparinized tubes and plasma was isolated. The experiments were conducted according to the ethical rules approved by Institutional Review Board (IRB).
Preparation of liver mitochondria
The liver was removed and minced with small scissors in a cold manitol solution containing 0.225 M D-manitol, 75 mM sucrose, and 0.2 mM ethylenediaminetetraacetic acid (EDTA). The minced liver (30 g) was gently homogenized in a glass homogenizer with a Teflon pestle and then centrifuged at 700 × g for 10 min at 4 ◦C at remove nuclei, unbroken cells, and other non-subcellular tissue. The supernatants were centrifuged at 7,000 × g for 20 min. These second supernatants were pooled as the crude microsomal fraction and the pale loose upper layer, which was rich in swollen or broken mitochondria, lysosomes, and some microsomes, of sediments was washed away.
The dark packed lower layer (heavy mitochondrial fraction) was resuspended in the manitol solution and recentrifuged twice at 7,000×g for 20 min. The heavy mitochondrial sediments were suspended in Tris solution containing 0.05 M Tris-HCl buffer (pH 7.4), 0.25 M sucrose, 20 mM KCl, 2.0 mM MgCl2, and 1.0 mM Na2HPO4 at 4 ◦C before assay (16).
Measurement of Cu/Zn- SOD
The activity of Cu/Zn- SOD was measured using a commercial kit (Ransod kit, Randox Laboratories Ltd, Crumlin, UK). Measurement of the enzyme was based on the generation of superoxide radicals produced by xanthine and xanthine oxidase and reacted with 2-(4-iodophenyl)-3-(4-nitrofenol) 5-phenyltetrazolium chloride (INT) to form a red formazan dye. The formazan was read at 505 nm. One unit of Cu/Zn- SOD was defined as the amount of enzyme necessary to produce 50% inhibition in the INT reduction rate.
Measurement of GPx
The amount of GPx was determined using a commercially available kit (Ransel kit, Randox Laboratories Ltd, Crumlin, UK) by measuring the rate of oxidation of NADPH at 340 nm. A unit of enzyme was expressed as the amount of enzyme needed to oxidize 1 nmol of NADPH oxidase/minute.
Measurement of reduced glutathione assay (GSH)
Level of reduced glutathione (GSH) was measured using colorimetric assay kit. The kit uses 5, 50-dithiobis-2-nitrobenzoic acid (DTNB) and glutathione reductase. The procedure was followed according to manufacturer’s instruction and the levels were quantitated as micromolar GSH based on standard supplied along with the kit.
Measurement of lipid peroxidation (LPO)
The LPO product in tissues was determined by thiobarbituric acid reagent expressed as the extent of malondialdehyde (MDA) productions during an acid heating reaction. Briefly, the samples were diluted by 1.5 mL TCA (20% w/v) was added to 250 μL o f this samples and centrifuged in 3000 g for 10 min. Then, the precipitation was dissolved in sulfuric acid and 1.5 mL of the mixture was added to 1.5 mL of TBA (0.2% w/v). The mixture was then incubated for 1 h in a boiling water bath. Following incubation, 2 m l of n-butanol was added, the solution centrifuged, cooled and the absorption of the supernatant was recorded in 532 nm. The calibration curve of tetraethoxypropane standard solutions was used to determine the concentrations of TBA+MDA adducts in samples (17).
Total Protein
The protein content was quantified by the method of Bradford. Concentrated Coomassie blue (G250) was diluted in 250 μL distilled water, and then 750 μL of this diluted dye was added to 50 μL of sample. The mixture was incubated at room temperature for 10 min and an absorbance measurement was taken at 595 nm by a spectrophotometer. A standard curve was constructed by using bovine serum albumin ranging between 0.25 and 1 mg/mL (18).
Mitochondrial viability
This assay is a quantitative colorimetric method to determine mitochondrial toxicity or viability of liver mitochondria. It utilizes the yellow tetrazolium salt (MTT), which is metabolized by mitochondrial dehydrogenase enzyme from viable cells to yield a purple formazan reaction product that was determined spectrophotometrically at wavelength of 570 nm. The percentage (of control) of mitochondrial viability of each test sample was calculated (19).
Statistical analysis
Mean and standard error values were determined for all the parameters and the results were expressed as Mean + SEM. All data were analyzed with SPSS Version 18 employing one-way ANOVA followed by Tukey post hoc test. Duplicate of experiments were performed. Differences between groups was considered significant when P < 0.05.
Results
Lipid peroxidation (LPO)
In blood: CCl4 caused a significant increase in LPO when compared to control (p-value < 0.001). Propofol caused a significant decrease in LPO when compared to CCl4 group (p-value < 0.001). Vitamin E reduced LPO when compared to CCl4 (p-value < 0.001). Co administration of Vitamin E with CCl4 significantly reduced CCl4 induced LPO (p-value < 0.001).
In liver mitochondria: CCl4 caused a significant increase in LPO when compared to control (p-value < 0.001). Propofol caused a significant decrease in LPO when compared to CCl4 group (p-value < 0.001). Vitamin E reduced LPO when compared to CCl4 (p-value < 0.001). Coadministration of propofol or Vitamin E with CCl4 significantly reduced CCl4 induced LPO (p-value = 0.050 and p-value = 0.010, respectively); Figure 1.
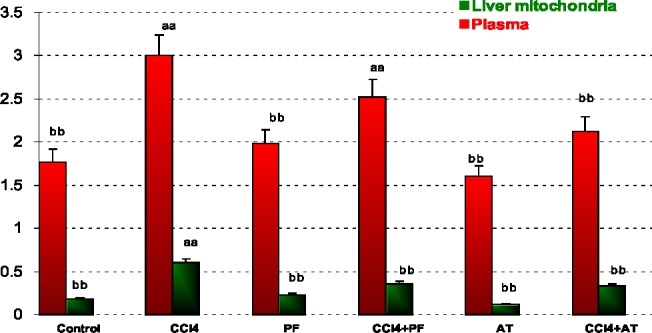
Superoxide dismutase
In blood: CCl4 caused a significant increase in SOD activity when compared to control (p-value < 0.001). Propofol caused a significant decrease in SOD activity when compared to CCl4 group (p-value < 0.001). Vitamin E reduced SOD activity when compared to CCl4 (p-value < 0.001).
In liver mitochondria: Vitamin E reduced SOD activity when compared to CCl4 (p-value = 0.014); Figure 2.
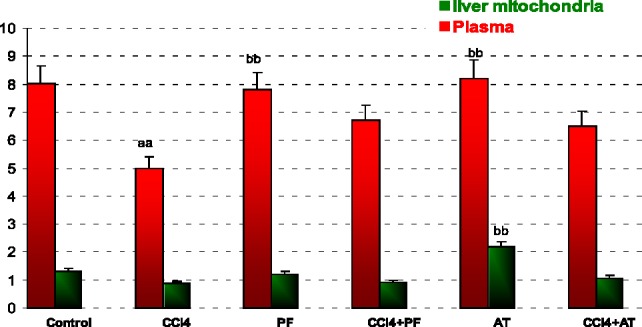
Glutathione peroxidase
In blood: Vitamin E reduced GPx activity when compared to CCL4 (p-value = 0.004). Coadministration of propofol with Vitamin E significantly reduced CCl4 induced SOD activity (p-value = 0.003 and p-value = 0.013, respectively).
In liver mitochondria: Vitamin E reduced GPx activity when compared to CCl4 (p-value = 0.001); Figure 3.
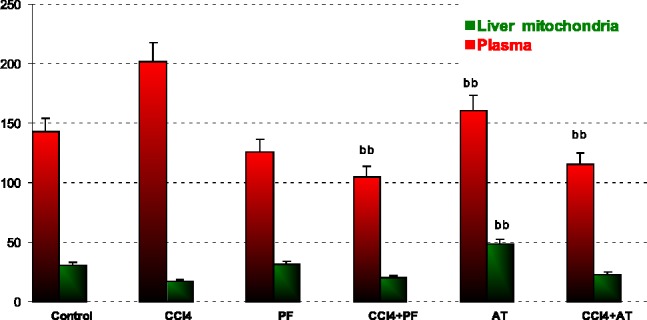
Reduced glutathione
In plasma: Treatment with CCl4 decreased GSH as compared to control (p-value = 0.002). Propofol increased GSH as compared to CCl4 group (p-value = 0.002). Vitamin E induced GSH when compared to CCl4 (p-value = 0.001).
In liver mitochondria: Administration of CCl4 decreased GSH in comparison to controls (p- value < 0.001). Treatment with propofol increased GSH as compared to CCl4 group (p-value < 0.001). Vitamin E significantly increased GSH level as compared to CCl4 group (p-value < 0.001). Coadministration of Vitamin E with CCl4 decreased GSH in comparison to propofol (p-value < 0.001); Figure 4.
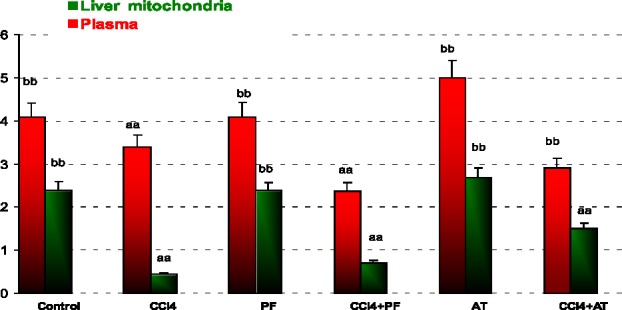
MTT cocentration
In liver mitochondria: administration of CCl4 decreased viability of cells in comparison to Vitamin E group (p-value < 0.001). Treatment with propofol increased viability of cells in comparison to CCl4 group ( p-value < 0.001).Coadministration of Vitamin E and propofol with CCl4 increased viability of mitochondrial cell when compared to CCL4 respectively(p- value < 0.001, p-value = 0.002,); Figure 5.
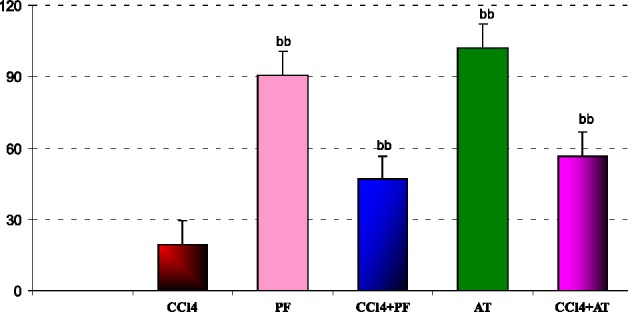
Discussion
Overall results of the present subchronic model of toxicity confirmed potential of CCl4 in induction of oxidative injuries and mitochondrial damage. Results indicated that anti-oxidant enzymes GPx, SOD and oxidative marker such as LPO are stimulated in response to exposure to CCl4, but their defense was not sufficient to overcome offending free radicals. As observed in the present results, the GSH is also reduced by CCl4. Fascinatingly, propofol indicate anti-oxidant properties as well as vitamin E were able to attenuate CCl4 induced changes in almost most tested biomarkers.
In other words, CCl4 induced oxidative stress by increasing free radicals like induction of cellular damage shown as increase of LPO and reduction of GSH, finally affecting anti-oxidant enzymes activities. The amelioration effect of propofol may be due to chemically similar to endogenous anti-oxidant a-tocopheral (Vitamin E) and theoretically should demonstrate similar properties (20). Numerous studies have demonstrated anti-oxidant effects of propofol in-vitro (5, 6, 21) and in-vivo (7), but actions of propofol on different cells are varied and multiple mechanisms may be involved. This result indicates reduction of LPO in propofol treatment group induced by CCl4. The anti-oxidant effects of propofol may also be due to its ability to attenuate the formation of lipid peroxides (22), to induce the expression of anti-oxidant enzyme heme oxygenase-1 (6), to decrease the expression of nitric oxide synthase (NOS), (23) and to stabilize the mitochondrial membrane (24). Our findings showed that, propofol reduced oxidative biomarkers against CCl4 toxicity in plasma and liver mitochondria. We determined that the propofol, mitochondrial toxicity significantly decreased, whereas it increased significantly in liver mitochondria following CCl4 administration. We think it is due to anti-oxidant properties of propofol in liver mitochondria. Propofol was also shown to promote mitochondrial function by stabilizing the transmembrane electrical potential (25, 26) and inhibiting mitochondrial permeability transition pore opening (27), both contributing to suppression of mitochondrion-dependent apoptotic signaling (28).These findings indicate that the toxic stress of CCl4 and the protective effects of propofol extensively involve mitochondrial viability (Figure 5). The injuries induced by CCl4 are resulted from free radicals through lipid peroxidation of cell membranes, reduces anti-oxidant enzyme and anti-oxidant substrates to induce oxidative stress that is an important factor in acute and chronic injuries in various tissues (15). The low levels of LPO in the groups receiving propofol in our study suggest that propofol prevents the lipid peroxidation caused by CCl4 (Figure 1).
Oxidative stress may result in overproduction of oxygen free-radical precursors and/or decreased efficiency of the anti-oxidant system. CCl4 and oxygen free-radical generation is associated with impaired glutathione metabolism, alterations in the anti-oxidant status (29). However, oxidative stress through generation of reactive oxygen species (ROS) plays an important role in producing liver damage and initiating hepatic fibrogenesis. Oxidative disruption of lipids, proteins, and DNA induces necrosis and apoptosis of hepatocytes, and amplifies the inflammatory response, resulting in initiation of fibrosis. Additionally, ROS stimulate the production of profibrogenic mediators from Kupffer cells and circulating inflammatory cells (30). In present study propofol inhibition of ROS formation, scavenging ROS, or interfering with ROS pathogenic signaling pathways might be the potential ways to protect mitochondrial cells from dysfunction by CCl4. While the contribution of Complex III to mitochondrial ROS production in the absence of respiratory inhibitors remains unresolved, two known effects of mitochondrial Ca+2 could conceivably promote ROS production at this site. One of the key events that cause mitochondrial injury is an abnormal increase in intracellular Ca+2 (31, 32). It is well known that mtPT can be mediated by a concerted action between Ca2+ and ROS, where ROS promote the oxidation and crosslinkage of mitochondrial membrane protein thiol groups such as GSH (33). The mechanisms of Ca+2-mediated mitochondrial damage involves activation of degradative enzymes, e.g., calpain proteases and phospholipases, or enzymes that generate reactive oxygen species (ROS) capable of oxidatively modifying mitochondrial proteins and lipids (34, 35). Reduced glutathione is a substrate for mitochondrial matrix glutathione peroxidase, an anti-oxidant enzyme that detoxifies H2O2 and other peroxides (36-38). The results of our present investigation showed that 0.2 mL/Kg CCl4 administration in rats caused significant induction in the activity of anti-oxidant enzymes and decreased GSH. GSH is a critical endogenous anti-oxidant involved in cell defense (39). Although GSH tends to be conserved preferentially in mitochondria, the concentration of GSH in mitochondria may be most relevant for the disposal of ROS and cell fate (40-42). The intracellular distribution of GSH, particulary interms of relative amounts of GSH and disulfide (GSSG), invarious compartments remains unclear. It is a evident, however, that GSH is present in cytosolic, mitochondrial and nuclear pools, can distribute to the nucleous under various condition and is involved in the redox regulation of signal transduction (43-45). Decrease in GSH activity during CCl4 toxicity might be due to the decreased availability of GSH resulted during the enhanced oxidative damage (46-48). Improvement of blood and liver mitochondrial GSH levels in rats treated with propofol in comparison to CCl4 administration further demonstrated the anti-oxidant properties of this drug (Figure 4). This effect is established via activating the glutathione anti-oxidant system (49, 50).
ROS are produced in normal metabolic processes and are inactivated by endogenous anti-oxidant systems (51). The primary ROS produced by mitochondria is superoxide [89]. This highly reactive free radical is extremely short-lived (52-54) and dismutates either spontaneously or with the help of the mitochondrial superoxide dismutase forming the more ROS, H2O2 (55, 56). According to our opinion, the significant decrease of GPx and SOD activity in Groups Vitamin when compared to CCl4 group may be explained with the suppression of physiologic ROS production by anti-oxidant agents (Figure 2, 3).
Recently in-vitro studies showed that propofol could effectively suppress apoptotic signaling and prevent apoptotic death of myocardial cells encountering fatal stimuli (6, 57, 58). In experimental hearts, propofol inhibited mitochondrial permeability transition (27) and ameliorated ischemia–reperfusion injury (59-61). In fact, sustained hepatic inflammation provoked by long-term treatment with CCl4 is believed to be through NF-κB pathway (62, 63). NF-κB is a transcription factor consisting of p65 and p50 subunits of the Rel protein family that regulates host inflammatory and immune responses by increasing the expression of specific cellular genes (64, 65). Released activated NF-κB complex tranlocates to the nucleus, where it regulates gene expression by binding to κB binding sites (61, 66).
These findings support the premise that propofol can guard against the sequences of oxidative stress. Hence, the anti-oxidant properties of propofol are involved in its hepatoprotective mechanism. The powerful anti-oxidant activity of propofol has been examined previously in different oxidative stress situations (67). Interestingly, as evidenced by MTT assay, the present data confirm that CCl4-induced mitochondrial dysfunction can be improved by propofol. However, the exact molecular mechanisms of a putative protective role of propofol in hepatoprotection should be further investigated.
Acknowledgements
References
-
1.
Marik PE. Propofol: an immunomodulating agent Pharmacotherapy. J. Hum. Pharm. Drug Ther. 2005;25:28-33.
-
2.
Tramer M, Moore A, McQuay H. Propofol anaesthesia and postoperative nausea and vomiting: quantitative systematic review of randomized controlled studies. Br J. Anaes. 1997;78:247-255.
-
3.
Schaer H. Propofol infusion for the maintenance of short-term anesthesia. Anaes. 1988;37:187-192.
-
4.
Ansley DM, Lee J, Godin DV, Garnett ME, Qayumi AK. Propofol enhances red cell antioxidant capacity in swine and humans. Can J. Anest. 1998;45:233-239.
-
5.
Tesauro M, Thompson W, Moss J. Effect of staurosporine-induced apoptosis on endothelial nitric oxide synthase in transfected COS-7 cells and primary endothelial cells. Cell Death Differen. 2005;13:597-606.
-
6.
Xu J, Wang Y. Propofol attenuation of hydrogen peroxide-mediated oxidative stress and apoptosis in cultured cardiomyocytes involves haeme oxygenase-1. Eur J. Anaes. 2008;25:395-402.
-
7.
Kobayashi K, Yoshino F, Takahashi SS, Todoki K, Maehata Y, Komatsu T, Yoshida K, Lee MC. Direct assessments of the anti-oxidant effects of propofol medium chain triglyceride/long chain triglyceride on the brain of stroke-prone spontaneously hypertensive rats using electron spin resonance spectroscopy. Anest. 2008:426-435.
-
8.
Chen J, Gu Y, Shao Z, Luo J, Tan Z. Propofol protects against hydrogen peroxide-induced oxidative stress and cell dysfunction in human umbilical vein endothelial cells. Mole Cell Biochem. 2010;339:43-54.
-
9.
Wang B, Shravah J, Luo H, Raedschelders K, Chen DDY, Ansley DM. Propofol protects against hydrogen peroxide-induced injury in cardiac H9c2 cells via Akt activation and Bcl-2 up-regulation. Biochem Biophys. Res. Commun. 2009;389:105-111. [PubMed ID: 19703415].
-
10.
Luo T, Xia Z. A small dose of hydrogen peroxide enhances tumor necrosis factor-alpha toxicity in inducing human vascular endothelial cell apoptosis: reversal with propofol. Anest Analg. 2006;103:110-116.
-
11.
Xia Z, Luo T, Liu H, Wang F, Irwin MG, Vanhoutte PM. L-arginine enhances nitrative stress and exacerbates tumor necrosis factor-[alpha] toxicity to human endothelial cells in culture: prevention by propofol. J Cardiol. Pharm. 2010;55:358.
-
12.
Abdollahi M, Ranjbar A, Shadnia S, Nikfar S, Rezaie A. Pesticides and oxidative stress: a review. Med Sci. Monitor. 2004;10:141.
-
13.
Ranjbar A, Ghahremani MH, Sharifzadeh M, Golestani A, Ghazi-Khansari M, Baeeri M, Abdollahi M. Protection by pentoxifylline of malathion-induced toxic stress and mitochondrial damage in rat brain. Hum Experi. Toxicol. 2010;29:851-864.
-
14.
Soleimani H, Ranjbar A, Baeeri M, Mohammadirad A, Khorasani R, Yasa N, Abdollahi M. Rat plasma oxidation status after Nigella Sativa L. botanical treatment in CCl4-treated rats. Toxicol. Mech. Meth.
-
15.
Szymonik-Lesiuk S, Czechowska G, Stryjecka-Zimmer M, Słomka M, Madro A, Celiński K, Wielosz M. Catalase, superoxide dismutase, and glutathione peroxidase activities in various rat tissues after carbon tetrachloride intoxication. J Hep. Bili. Pan. Sur. 2003;10:309-315.
-
16.
Ghazi-Khansari M, Mohammadi‐Bardbori A, Hosseini MJ. Using janus green B to study paraquat toxicity in rat liver mitochondria. Ann New York Acad. Sci. 2007;1090:98-107. [PubMed ID: 17384251].
-
17.
Moore K, Roberts LJ. Measurement of lipid peroxidation. Free Rad Res. 1998;28:659-671.
-
18.
Kruger NJ. The Bradford method for protein quantitation. Methods Mol Biol. 1994;32:9-15. [PubMed ID: 7951753].
-
19.
Morgan DML. Tetrazolium (MTT) assay for cellular viability and activity. Method Molec Biol. Clif. Totow. 1997;79:179-183.
-
20.
Bayona NA, Gelb AW, Jiang Z, Wilson JX, Urquhart BL, Cechetto DF. Propofol neuroprotection in cerebral ischemia and its effects on low-molecular-weight antioxidants and skilled motor tasks. Anest. 2004;100:1151.
-
21.
Wang B, Luo T, Chen D, Ansley DM. Propofol reduces apoptosis and up-regulates endothelial nitric oxide synthase protein expression in hydrogen peroxide-stimulated human umbilical vein endothelial cells. Anest Analg. 2007;105:1027-1033.
-
22.
Kahraman S, Kilinc K, Dal D, Erdem K. Propofol attenuates formation of lipid peroxides in tourniquet-induced ischaemia-reperfusion injury. Brit J. Anaes. 1997;78:279-281.
-
23.
Goode HF, Cowley HC, Walker BE, Howdle PD, Webster NR. Decreased antioxidant status and increased lipid peroxidation in patients with septic shock and secondary organ dysfunction. Crit Care Med. 1995;23:646-651. [PubMed ID: 7712754].
-
24.
Eriksson O. Effects of the general anaesthetic Propofol on the Ca2+-induced permeabilization of rat liver mitochondria. FEBS Lett. 1991;279:45-48. [PubMed ID: 1704857].
-
25.
Branca D, Roberti MS, Lorenzin P, Vincenti E, Scutari G. Influence of the anesthetic 2, 6-diisopropylphenol on the oxidative phosphorylation of isolated rat liver mitochondria. Biochem Pharm. 1991;42:87-90. [PubMed ID: 2069600].
-
26.
Tsujimoto Y, Shimizu S. Role of the mitochondrial membrane permeability transition in cell death. Apop. 2007;12:835-840.
-
27.
Javadov SA, Lim KHH, Kerr PM, Suleiman MS, Angelini GD, Halestrap AP. Protection of hearts from reperfusion injury by propofol is associated with inhibition of the mitochondrial permeability transition. Card Res. 2000;45:360-369.
-
28.
Crompton M. The mitochondrial permeability transition pore and its role in cell death. Biochem J. 1999;341:233. [PubMed ID: 10393078].
-
29.
Gilbert MT, Haselkorn T, Bunce M, Sanchez JJ, Lucas SB, Jewell LD, Van Marck E, Worobey M. The isolation of nucleic acids from fixed, paraffin-embedded tissues–which methods are useful when? PLoS One. 2007;2:537.
-
30.
Friedman SL. Mechanisms of hepatic fibrogenesis. Gastro. 2008;134:1655-1669.
-
31.
Schild L, Huppelsberg J, Kahlert S, Keilhoff G, Reiser G. Brain mitochondria are primed by moderate Ca2+ rise upon hypoxia/reoxygenation for functional breakdown and morphological disintegration. J Biol. Chem. 2003;278:25454-25460. [PubMed ID: 12702720].
-
32.
Abo-Hashema KAH, Cake MH, Potter IC. Liver mitochondria, confirmed as intact by complete suppression of succinate uptake and oxidation, possess a carnitine palmitoyltransferase I that is totally inhibited by malonyl CoA. Biochem Biophys. Res. Commun. 1999;258:778-783. [PubMed ID: 10329463].
-
33.
Maciel EN, Vercesi AE, Castilho RF. Oxidative stress in Ca2+‐induced membrane permeability transition in brain mitochondria. J Neurochem. 2002;79:1237-1245. [PubMed ID: 11752064].
-
34.
Starkov AA, Chinopoulos C, Fiskum G. Mitochondrial calcium and oxidative stress as mediators of ischemic brain injury. Cell Cal. 2004;36:257-264.
-
35.
Borutaite V. Mitochondria as decision‐makers in cell death. Environm Mol. Mut. 2010;51:406-416.
-
36.
Andreyev AY, Kushnareva YE, Starkov A. Mitochondrial metabolism of reactive oxygen species. Biochem. 2005;70:200-214. [PubMed ID: 15807660].
-
37.
Marí M, Morales A, Colell A, García-Ruiz C, Fernández-Checa JC. Mitochondrial glutathione, a key survival antioxidant. Antioxid Redox. Signal. 2009;11:2685-2700. [PubMed ID: 19558212].
-
38.
Shlafer M, Myers CL, Adkins S. Mitochondrial hydrogen peroxide generation and activities of glutathione peroxidase and superoxide dismutase following global ischemia. J Mol. Cell Card. 1987;19:1195-206.
-
39.
Fernández‐Checa J, García‐Ruiz C, Colell A, Morales A, Marí M, Miranda M, Ardite E. Oxidative stress: role of mitochondria and protection by glutathione. Biof. 2008;8:7-11.
-
40.
Griffith OW, Meister A. Origin and turnover of mitochondrial glutathione. Proceed. Nat. Acad. Sci. USA; 1985. 4668 p.
-
41.
Kurosawa K, Hayashi N, Sato N, Kamada T, Tagawa K. Transport of glutathione across the mitochondrial membranes. Biochem Biophy. Res. Commun. 1990;167:367-372.
-
42.
von Montfort C, Matias N, Fernandez A, Fucho R, Conde de la Rosa L, Martinez-Chantar ML, Mato JM, Machida K, Tsukamoto H, Murphy MP, Mansouri A, Kaplowitz N, Garcia-Ruiz C, Fernandez-Checa JC. Mitochondrial GSH determines the toxic or therapeutic potential of superoxide scavenging in steatohepatitis. J Hep. 2012;57:852-859.
-
43.
Voehringer D, McConkey D, McDonnell T, Brisbay S, Meyn R. Bcl-2 expression causes redistribution of glutathione to the nucleus. Proceed Nat. Aca. Sci. 1998;95:2956-2960.
-
44.
Briviba K, Fraser G, Sies H, Ketterer B. Distribution of the monochlorobimane-glutathione conjugate between nucleus and cytosol in isolated hepatocytes. Biochem J. 1993;294:631. [PubMed ID: 8379916].
-
45.
Nunes C, Barbosa RM, Almeida L, Laranjinha J. Nitric oxide and DOPAC-induced cell death: from GSH depletion to mitochondrial energy crisis. Mol Cell. Neurosci. 2011;48:94-103. [PubMed ID: 21708261].
-
46.
Khan RA, Khan MR, Ahmed M, Sahreen S, Shah NA, Shah MS, Bokhari J, Rashid U, Ahmad B, Jan S. Hepatoprotection with a chloroform extract of Launaea procumbens against CCl4-induced injuries in rats. BMC Complem Alter. Med. 2012;12:114.
-
47.
Mujeeb M, Khan SA, Aeri V, Ali B. Hepatoprotective Activity of the Ethanolic Extract of Ficus carica Linn. Leaves in Carbon Tetrachloride-Induced Hepatotoxicity in Rats. Iran. J. Pharma. Sci. 2011;10:301-306.
-
48.
Balbis E, Patriarca S, Furfaro AL, Millanta S, Sukkar SG, Marinari UM, Pronzato MA, Cottalasso D, Traverso N. Whey proteins influence hepatic glutathione after CCl4 intoxication. Toxicol Indus. Health. 2009;25:325-328.
-
49.
De La Cruz J, Zanca A, Carmona J, de la Cuesta FS. The effect of propofol on oxidative stress in platelets from surgical patients. Anest Analg. 1999;89:1050.
-
50.
Oztürk E, Demirbilek S, Köroğlu A, But A, Begeç ZO, Gülec M, Akyol O, Ersoy MO. Propofol and erythropoietin antioxidant properties in rat brain injured tissue. Prog Neuro-Psychopharm. Biol. Psych. 2008;32:81-86.
-
51.
Halliwell B. Free radicals and antioxidants: a personal view. Nut Rev. 1994;52:253-565.
-
52.
Dionisi O, Galeotti T, Terranova T, Azzi A. Superoxide radicals and hydrogen peroxide formation in mitochondria from normal and neoplastic tissues. Biochim Biophys. Acta. 1975;403:292. [PubMed ID: 241399].
-
53.
Buettner GR. Superoxide dismutase in redox biology: the roles of superoxide and hydrogen peroxide. Anti-can Age Med. Chem. 2011;11:341.
-
54.
Zhang L, Yu L, Yu CA. Generation of Superoxide Anion by Succinate-Cytochromec Reductase from Bovine Heart Mitochondria. J Biol. Chem. 1998;273:33972-33976. [PubMed ID: 9852050].
-
55.
Murphy MP. How mitochondria produce reactive oxygen species. Biochem J. 2009;417:1. [PubMed ID: 19061483].
-
56.
Sawyer DT, Valentine JS. How super is superoxide? Acco. Chem Res. 1981;14:393-400.
-
57.
Kim HS, Chang WC, Hwang KC, Choi IG, Park WK. Effect of propofol on calcium homeostasis in hypoxia-reoxygenated neonatal rat cardiomyocytes. Eur J. Pharm. 2008;594:139-145.
-
58.
Noh HS, Shin IW, Ha JH, Hah YS, Baek SM, Kim DR. Propofol protects the autophagic cell death induced by the ischemia/reperfusion injury in rats. Mol Cells. 2010;30:455-460. [PubMed ID: 20821058].
-
59.
Dehkordi ME, Razavi SS, Momenzadeh S. A Comparison between Sedative Effect of Propofol-Fentanyl and Propofol-Midazolam Combinations in Microlaryngeal Surgeries. Iran J. Pharma. Sci. 2012;11:287-94.
-
60.
Xia W, Liu Y, Zhou Q, Tang Q, Zou H. Comparison of the effects of propofol and midazolam on inflammation and oxidase stress in children with congenital heart disease undergoing cardiac surgery. Yon Med. J. 2011;52:326-332.
-
61.
Lai HC, Yeh YC, Wang LC, Ting CT, Lee WL, Lee HW, Wang KY, Wu A, Su CS, Liu TJ. Propofol ameliorates doxorubicin-induced oxidative stress and cellular apoptosis in rat cardiomyocytes. Toxicol App. Pharm. 2011;257:437-448.
-
62.
Orfila C, Lepert JC, Alric L, Carrera G, Béraud M, Pipy B. Immunohistochemical distribution of activated nuclear factor κB and peroxisome proliferator-activated receptors in carbon tetrachloride-induced chronic liver injury in rats. Histochem Cell Bio. 2005;123:585-593. [PubMed ID: 15959796].
-
63.
Son G, Iimuro Y, Seki E, Hirano T, Kaneda Y, Fujimoto J. Selective inactivation of NF-κB in the liver using NF-κB decoy suppresses CCl4-induced liver injury and fibrosis. Am J. Physiol. Gastro. Liver Physiol. 2007;293:631-639.
-
64.
Ali S, Mann DA. Signal transduction via the NF‐κB pathway: a targeted treatment modality for infection, inflammation and repair. Cell Biochem Func. 2004;22:67-79.
-
65.
Hamdy N, El-Demerdash E. New therapeutic aspect for carvedilol: Antifibrotic effects of carvedilol in chronic carbon tetrachloride-induced liver damage. Toxicol Appl. Pharm. 2012;261:292-299.
-
66.
Hayden M, West A, Ghosh S. NF-κB and the immune response. Oncogene. 2006;25:6758-6780. [PubMed ID: 17072327].
-
67.
Kostopanagiotou GG, Grypioti AD, Matsota P, Mykoniatis MG, Demopoulos CA, Papadopoulou-Daifoti Z, Pandazi A. Acetaminophen-induced liver injury and oxidative stress: protective effect of propofol. Eur J. Anaest. 2009;26:548-553.